DOI:
10.1039/C5RA26182A
(Paper)
RSC Adv., 2016,
6, 8282-8289
Polymeric β-alanine incarcerated Pd(II) catalyzed allylic etherification in water: a mild and efficient method for the formation of C(sp3)–O bonds†
Received
8th December 2015
, Accepted 4th January 2016
First published on 8th January 2016
Abstract
A new heterogeneous palladium(II) catalyst has been developed through a convenient and economic way. The catalyst was synthesized by confining palladium metal with a polystyrenal β-alanine-imine network and characterized by FT-IR spectroscopy, thermogravimetric analysis, field emission scanning electron microscopy, energy dispersive X-ray, and elemental analysis. Polymeric imine can be prepared easily from chloromethylated polystyrene and β-alanine. Using this polymer incarcerated palladium(II) catalyst a useful and efficient procedure for stereospecific synthesis of allyl-aryl ethers has been developed. The benzylic, aromatic, and heteroaromatic phenols react with various substituted allyl acetates by this procedure to furnish a library of allyl-aryl and allyl-hetero-aryl ethers in high yields. The catalyst could be recovered easily and reused five times without any considerable loss of its catalytic activity.
1 Introduction
Homogeneous palladium complexes have been used successfully for a variety of reactions in organic synthesis.1 But due to the chance of metal contamination with the end product and the difficulty to recover and reuse the homogeneous catalyst, heterogeneous Pd catalysts have emerged as sustainable alternatives. Thus the immobilization of palladium on a heterogeneous support has received considerable attention to provide an effective catalyst overcoming the drawbacks of homogeneous catalysis.2 Furthermore, the use of water as the reaction medium is a top priority because of its unique reactivity and selectivity which is different from those in organic solvents, in addition to its environmental acceptability and low cost.3 Thus, the combination of heterogeneous, inexpensive, ligand-free, robust, recyclable catalysts and water as a solvent for a chemical transformation is highly desirable in the context of environmental and industrial concerns. Recently, polymer-supported phosphine and amine ligand have been developed to immobilize palladium metals onto polymers.4 Our group has explored few polymer supported metal catalyst for hydrogenation,5 C–C and C–N cross coupling reaction6 and catalytic oxidation.7 We report here a simple preparation and characterization of a new polymeric β-alanine-imine incarcerated highly active Pd(II) catalyst and exemplified its application for the stereo-specific allylic etherification reactions in water under mild condition. The catalyst was prepared easily by two step reaction. The initial step is the formation of polymeric imine by the condensation of chloromethylated polystyrene with readily available and inexpensive β-alanine. Finally the PS-Pd-ala catalyst was prepared by the immobilizing the palladium metal on polymeric imine, for the first time.
The construction of C(sp3)–O bond is very important in organic synthesis as it constitutes the key step in the synthesis of many pharmaceuticals.8 Allyl ethers are employed as useful starting material in a wide variety of reactions such as 1,3-hydrogen shift, [3,3]-sigmatropic rearrangement, and polymerization reactions.9 They are also found as core units of several biologically active molecules and natural products.10 In addition, allyl groups act as efficient protecting groups for phenol derivatives.11 Usually allylation is performed using allyl alcohol derivatives or halides.12 But, easy availability and configurational stability of allyl acetate make it preferable allylating agents compared to highly activated, unstable allyl halides and tosylates.13 Transition metal-catalyzed allylation of carbon, nitrogen and sulfur nucleophile is one of the most frequently employed transformations in organic synthesis.14–17 Generally, allyl ethers are prepared by the reaction between alkyl halide and phenols using strong base e.g. metal alkoxide.18 However, allylation with soft phenolic nucleophile has received less attention. Few transition metal complexes such as Pd,19 Ru,20 Ir,21 Ni22 have been explored as the catalysts for the synthesis of allyl ethers. In fact, majority of the methods involved non recyclable, costly homogeneous transition metal catalyst. Therefore an alternative sustainable heterogeneous catalyst is highly desirable for the synthesis of allyl ether. Varma et al. reported a mild procedure for the general O-allylation of phenols with allyl acetates using magnetically separable heterogeneous Pd catalyst.23 Here we report the application of our catalyst for a mild and efficient allylic C(sp3)–O bond formation (Scheme 1).
 |
| Scheme 1 Palladium catalyzed allylic etherification. | |
2 Results and discussion
2.1 Allylic etherification
To probe the optimum reaction conditions, a series of experiments were performed with variation of reaction parameters such as base, solvent, temperature and time for a representative O-allylation of cinnamyl acetate (1b) with p-cresol (2b). The results are summarized in Table 1. Water was found to be best solvent among THF, DMF, toluene, 1,4-dioxane, CH3CN, NMP and DMSO tried for this reaction. Among various bases K2CO3 was found more effective than Cs2CO3 and K3PO4 under identical condition. NaHCO3 shows almost similar activity compared to K2CO3.
Table 1 Standardization of reaction conditionsa

|
Entry |
Solvent |
Base |
Temp. (°C) |
Time (h) |
Yielda (%) |
Yields refer to those of purified products characterized by 1H and 13C NMR spectroscopic data. Without using catalyst. In presence of TEMPO. Palladium(II) acetate was used as a catalyst. |
1 |
THF |
K2CO3 |
70 |
16 |
39 |
2 |
DMF |
K2CO3 |
110 |
16 |
62 |
3 |
Toluene |
K2CO3 |
110 |
16 |
18 |
4 |
Dioxane |
K2CO3 |
105 |
16 |
43 |
5 |
MeCN |
K2CO3 |
80 |
16 |
16 |
6 |
NMP |
K2CO3 |
110 |
16 |
38 |
7 |
DMSO |
K2CO3 |
110 |
16 |
44 |
8 |
H2O |
K2CO3 |
100 |
16 |
90 |
9 |
H2O |
K3PO4 |
100 |
16 |
42 |
10 |
H2O |
Cs2CO3 |
100 |
16 |
37 |
11 |
H2O |
NaHCO3 |
100 |
16 |
85 |
12 |
H2O |
K2CO3 |
100 |
30 |
91 |
13 |
H2O |
K2CO3 |
rt |
30 |
— |
14 |
H2O |
K2CO3 |
70 |
20 |
83 |
15b |
H2O |
K2CO3 |
100 |
12 |
— |
16c |
H2O |
K2CO3 |
100 |
16 |
88 |
17d |
H2O |
K2CO3 |
100 |
16 |
76 |
The reaction failed to initiate at room temperature and these are then carried out under refluxing condition. There was no reaction in the absence of supported Pd(II) catalyst (Table 1, entry 15). The yield of the product (3c) was not affected after the addition of 1 equiv. of TEMPO (Table 1, entry 16). Best yield was obtained using H2O as a solvent and 10 mol% Pd(II) catalyst in presence of K2CO3 under reflux for 16 h (argon atmosphere) (Table 1, entry 8). In our preliminary screening, it was exciting to demonstrate that polymer incarcerated Pd(II) catalyst was slightly more reactive than the homogeneous Pd(II) acetate catalyst (Table 1, entry 17).
Both aliphatic and aromatic allyl acetates were found to be efficient substrates for the O-allylation reaction. The allyl acetate bearing phenyl with an electron-donating group at para-position formed the product in good yield and the reactions are more facile than those with unsubstituted one. Significantly, the reaction is uniform with ortho- and para-substituted cinnamyl acetates. The O-allylation of (E)-3-(4-bromophenyl)allyl acetate is highly chemoselective as the attack of nucleophile occurs only at allylic position leaving –Br group on the aromatic ring unaffected (Table 2, 3r).24 A series of diversely substituted phenols underwent reactions with substituted allyl acetates by this procedure to provide the corresponding allyl-aryl ethers. The phenols bearing various electron donating (–Me, –OMe) and withdrawing moieties (–F, –COMe) at the different positions of the aromatic ring underwent coupling with allyl and cinnamyl acetates without any difficulty (Table 2). With an ortho-2,6-dimethoxy-substituted phenol, the cinnamyl acetate (1b) gave a reasonable yield of the allylation product, although the nucleophile is more sterically hindered (Table 2, 3g). It was found that the reactivity of hydroxy groups attached with aromatic system is greater than aliphatic one. We performed the reaction of cinnamyl acetate with 4-(hydroxymethyl)phenol and (4-(cinnamyloxy)phenyl)methanol was obtained as the single product (Table 2, 3j). We also investigated the reactions of several heterocyclic phenols with allyl and cinnamyl acetates and the coupling reaction occurs without any difficulty (Table 2, 3l and 3m).
Table 2 Synthesis of allyl aryl ether using Pd catalysta

|
Yields refer to those of purified products characterized by 1H and 13C NMR spectroscopic data. |
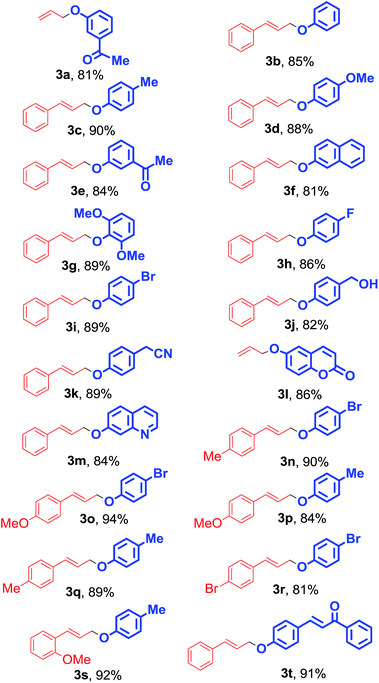 |
Significantly, the (E)-cinnamyl acetates and substituted phenol produced the corresponding (E)-allyl aryl ether with complete retention of stereochemistry. Interestingly, the (Z)-cinnamyl acetates also provided (E) products with excellent stereospecificity (Scheme 2). Substituted benzyl alcohols also coupled with cinnamyl acetate successfully to produce the corresponding ethers (Scheme 3, 5a). In this reaction we have used strong base like NaOH instead of K2CO3 for the completion of reaction.
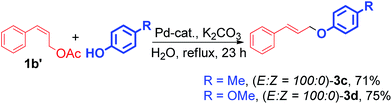 |
| Scheme 2 Synthesis of (E)-allyl-aryl ether using (Z)-cinnamyl acetate. | |
 |
| Scheme 3 Synthesis of benzyl-allyl ether using benzyl alcohol. | |
In case of branched allyl acetate (Scheme 4, 3c and 3u), this reaction shows equal efficiency to provide linear allyl-aryl ether which also supports the mechanism that entails the intermediacy of an η3-π-allyl Pd complex as the key intermediate in the reaction (Scheme 5).
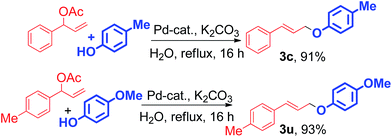 |
| Scheme 4 O-Allylation using branched cinnamyl acetate. | |
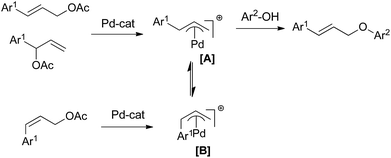 |
| Scheme 5 Plausible mechanism. | |
We suggest that the reaction proceeds through an interaction of Pd(II) with allyl acetate followed by oxidative addition with substituted phenol resulting a η3-π-allyl complex, which undergoes reductive elimination to give the product (Scheme 5).17a In case of trans-allyl acetate, it is predicted that the direct interaction of π-allyl complex [A] with phenol, followed by reductive elimination gives the trans product. However in case of cis-allyl acetate, the intermediate [B], formed after the oxidative addition, is likely to equilibrate with stable, less congested intermediate [A] and finally gives the trans product.
3 Experimental
3.1 Loading of metal ions on to the polymeric ligand
Polymeric ligand (3) (1 g) was stirred for 20 h with palladium acetate (20 mg) in acetic acid (10 mL) at 100 °C (Scheme 6). At the end of the reaction, the resulting metal-loaded polymer was filtered, washed with water, followed by methanol and finally dried under vacuum for 6 h at 90 °C.
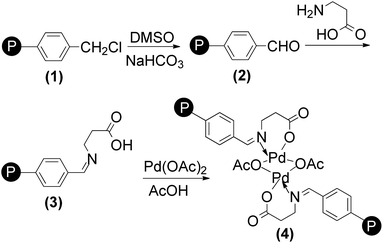 |
| Scheme 6 Outline for the synthesis of polymer incarcerated palladium(II) catalyst. | |
3.2 Characterization of supported palladium(II) complex
Due to insolubility of the polymer supported Pd(II) complex in all common organic solvents, characterization was limited to the physicochemical properties, chemical analysis, SEM, TGA, IR, and UV-Vis spectral data. Elemental analysis data for polymer supported ligand and catalyst are tabulated in Table 3. Amount of metal was determined by stripping the bound metal from the support and analysis by using atomic absorption spectrophotometer. Palladium content was found in the catalyst is 10.34 wt% of Pd analyzed by AAS (Table 3). Palladium content remained almost unchanged even after recycling the catalyst for five cycles.
Table 3 Chemical composition of polymer supported ligand (3) and Pd-PS-ala catalyst (4)
Compound |
C% |
H% |
N% |
Pd% |
Palladium content of recycled catalyst after 5th cycles. |
Ligand (3) |
74.96 |
6.36 |
4.61 |
— |
Pd-PS-ala (4) |
71.94 |
6.97 |
4.78 |
10.34 (10.23a) |
IR study. The mode of attachment of β-alanine and palladium metal onto the support was confirmed by IR spectral bands (Fig. 1). In the FT-IR spectrum of aldehyde functionalized polymer (2), a new peak appeared at 1705 cm−1 that was assigned to carbonyl C
O bond stretching vibration of the aldehyde group. In the polymer (2) the sharp C–Cl peak due to –CH2Cl group at 1264 cm−1 was absent.25 The formation of the Schiff base moiety on the polymeric support (3) is indicated by a peak at 1633 cm−1 assigned to C
N stretching frequency. This band shifted to lower wave numbers after complexation with palladium metal. The polymer supported Pd-catalyst (4) exhibited IR peaks at 1574 cm−1, 1423 cm−1 (ν COO bridged).26 It suggests that, Schiff base coordinated to the central palladium ion through the azomethine nitrogen.
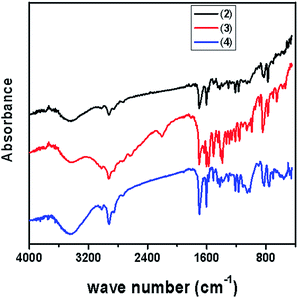 |
| Fig. 1 FT-IR spectra of (2) aldehyde functionalised polymer, (3) polymer supported Schiff base ligand and (4) polymer supported Pd(II) catalyst, Pd-PS-ala. | |
DRS-UV study. The electronic spectrum (Fig. 2) of the polymer supported schiff base ligand and palladium catalyst (Pd-PS-ala) have been recorded in the diffuse reflectance spectrum mode as MgCO3/BaSO4 disc due to its solubility limitations in common organic solvents. In the catalyst, broad bands around 260 to 350 nm are observed. This absorption bands may be attributed to π → π* and n → π* transition in phenyl moiety. The bands at 390–430 nm arise due to LMCT.
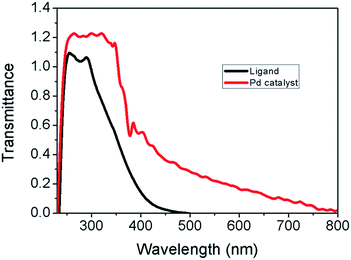 |
| Fig. 2 DRS-UV-Visible spectra of ligand and Pd-PS-ala catalyst. | |
Scanning electron micrographs (SEM) and energy dispersive X-ray analysis (EDX). The morphology of the catalyst was studied using Scanning Electron Microscope (SEM). The micrographs of the polymer anchored β-alanine ligand and palladium(II) catalyst obtained from the scanning electron microscope are presented in Fig. 3a and b respectively. The morphological changes in the polymer supported ligand and polymer supported catalyst are quite evident from these images. The micrograph of five time reused Pd-PS-ala catalyst is also presented in Fig. 3c. Quite uniform and negligible variation in size is observed throughout the both specimens. Energy dispersive spectroscopy analysis of X-rays (EDX) data for the polymer supported palladium complex is given in Fig. 4. The EDX data also inform the attachment of palladium on the surface of the polymer matrix.
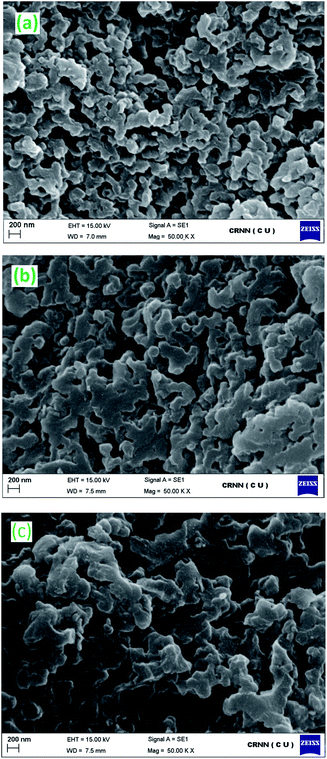 |
| Fig. 3 SEM images of (a) polymer supported Schiff base ligand, (b) polymer supported Pd(II) catalyst, Pd-PS-ala, (c) five times recycled polymer supported Pd(II) catalyst, Pd-PS-ala. | |
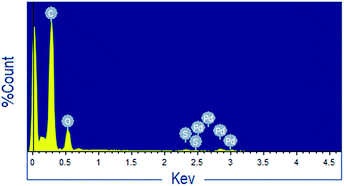 |
| Fig. 4 EDAX data of polymer supported Pd(II) catalyst, Pd-PS-ala. | |
TGA analysis. Thermal stability of the complex was investigated using TGA at a heating rate of 10 °C min−1 in air over a temperature range of 30–600 °C. TGA curve of the polymer supported Pd(II) catalyst is shown in Fig. 5. The Pd(II)-complex is stable up to 370–390 °C and above that temperature it is decomposed. Thermogravimetric study suggests that the polymer supported palladium complex degrade at considerably higher temperature.
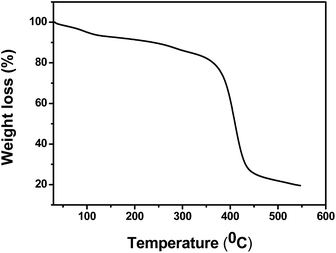 |
| Fig. 5 TGA plots for Pd-PS-ala catalyst. | |
3.3 General procedure for coupling of phenol with allyl acetate
A mixture of polymer supported Pd(II) catalyst (30 mg), cinnamyl acetate (1 mmol), p-cresol (1 mmol), potassium carbonate (2 mmol) and water (3 mL) was placed in a 10 mL round bottom flask and was stirred for 16 h at 110 °C. The reaction was monitored by TLC. After completion of reaction, the catalyst was removed by simple filtration through celite-545. Then ethyl acetate was added to the reaction mixture and the organic layer was separated from water. The desired product was isolated from the extracted organic part by column chromatography over silica gel (ethyl acetate/hexane) to provide pure 1-(cinnamyloxy)-4-methylbenzene (3c) as colourless oil; yield: 209 mg (90%). The spectroscopic data (1H NMR and 13C NMR) of this product are in good agreement with those of an authentic sample.
3.4 Heterogeneity test
To verify the heterogeneity of the catalyst, 1 mmol p-cresol, 1 mmol cinnamyl acetate and 30 mg catalyst were allowed to stir in 3 mL water at 110 °C. The reaction was stopped after 8 h, and the conversion was found to be 56% by GC-analysis. At this stage, the catalyst was separated from the reaction medium and the reaction was continued with the filtrate for next 8 h. After total 16 h the extent of conversion was found to remain the same. Moreover, UV-Visible spectra of the catalyst free reaction mixture did not show any absorption peak responsible for palladium. This information indicates that the reaction was followed by a heterogeneous pathway. Further, the leaching of palladium from the catalyst was checked by analyzing (EDX, IR) the used catalyst. Analysis of the used catalyst did not show appreciable loss in palladium content as compared to that of fresh catalyst. Beside, IR-spectrum of the recycled catalyst was quite similar to that of the fresh sample indicating the heterogeneous nature of the catalyst. The UV-Visible spectroscopy was also used to determine the stability of the catalyst. The metal content of the recycled catalyst was determined with the help of AAS and it was found that palladium content of the recycled catalyst remained almost unchanged. These observations strongly suggest that the catalyst is truly heterogeneous in nature.
3.5 Recovery of catalyst
At the end of the reaction, the reaction mixture was filtered. Then the recovered catalyst was thoroughly washed with methanol, dried and then further washed with diethyl ether to remove any traces of organic materials. The catalyst was then finally dried at 55 °C for 5 h under vacuum to activate. The catalyst can be recycled for five times without losing the activity (Fig. 6).
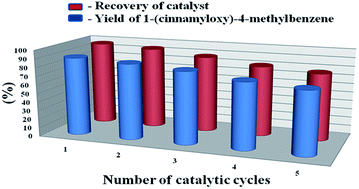 |
| Fig. 6 Recyclability chart for Pd-PS-ala catalyst. | |
The known compounds were identified by comparison of their spectra with those of authentic samples 3a,27a 3b,23a 3c,27b 3d,27b 3f,23a 3i,27c 3l,27d 3o,27e 3p,23a 3q,19f 3u.27f The unknown compounds were properly characterized by their spectroscopic data (IR, 1H NMR, 13C NMR and HRMS) data and elemental analysis and these data are provided below in order of their numbers in the respective tables and schemes.
1-[3-(3-Phenyl-allyloxy)-phenyl]-ethanone (Table 3, 3e). Yellow viscous oil; IR (neat): 3415, 3027, 2923, 2853, 1684, 1580, 1484, 1438, 1356, 1269, 1212, 1018 cm−1; 1H NMR (400 MHz, CDCl3): δ 2.46 (s, 3H), 4.59 (d, J = 5.6 Hz, 2H), 6.24–6.31 (m, 1H), 6.62 (d, J = 15.6 Hz, 1H), 7.02–7.29 (m, 7H), 7.42 (d, J = 7.6 Hz, 2H); 13C NMR (75 MHz, CDCl3): δ 26.7, 68.9, 113.6, 120.3, 121.4, 124.0, 126.7 (2C), 128.1, 128.7 (2C), 129.7, 133.4, 136.4, 138.6, 158.9, 197.9; HR-MS (EI): m/z = 275.1046 (M + Na+), calcd for C17H16O2: 275.1048.
1,3-Dimethoxy-2-(3-phenyl-allyloxy)-benzene (Table 3, 3g). Pale yellow solid; IR (KBr): 3417, 3036, 2989, 2958, 2865, 1889, 1609, 1510, 1460, 1252, 1241, 1177, 1112, 1031 cm−1; 1H NMR (400 MHz, CDCl3): δ 3.78 (s, 6H), 4.59 (d, J = 6.0 Hz, 2H), 6.45–6.53 (m, 1H), 6.65 (dd, J1 = 7.6 Hz, J2 = 16 Hz, 3H), 6.91 (d, J = 8.4 Hz, 1H), 7.41 (d, J = 15.6 Hz, 2H), 7.45–7.51 (m, 3H); 13C NMR (75 MHz, CDCl3): δ 56.1 (2C), 73.8, 105.4 (2C), 123.7, 123.9, 126.5 (2C), 128.2, 128.65 (2C), 129.1, 132.9, 152.6 (2C), 153.8; anal. calcd for C17H18O3: C 75.53, H 6.71; found: C 74.98, H 6.65%.
1-Fluoro-4-(3-phenyl-allyloxy)-benzene (Table 3, 3h). White solid; IR (KBr): 3564, 3390, 3020, 2854, 1635, 1506, 1215 cm−1; 1H NMR (400 MHz, CDCl3): δ 4.59 (d, J = 1.2 Hz, 2H), 6.31–6.36 (m, 1H), 6.65 (d, J = 16 Hz, 1H), 6.81–6.84 (m, 2H), 6.89–6.93 (m, 2H), 7.18–7.21 (m, 2H), 7.24–7.27 (m, 2H), 7.33–7.35 (m, 2H); 13C NMR (75 MHz, CDCl3): δ 69.5, 114.2, 116.0 (d, J = 18.7 Hz, 2C), 116.1, 124.5 (2C), 126.7 (2C), 128.7 (2C), 133.3, 136.6, 155.8 (d, J = 207.5 Hz, 1C), 158.7; anal. calcd for C15H13FO: C 78.93, H 5.74; found: C 78.81, H 5.82%.
(4-(Cinnamyloxy)phenyl)methanol (Table 3, 3j). Yellowish viscous liquid; IR (neat): 3645, 3583, 3415, 3361, 3294, 3242, 2927, 2856, 1747, 1650, 1508 cm−1; 1H NMR (400 MHz, CDCl3): δ 4.65 (s, 2H), 4.73 (d, J = 6.5 Hz, 2H), 6.41–6.47 (m, 1H), 6.76 (d, J = 16.0 Hz, 1H), 6.98 (d, J = 8.4 Hz, 1H), 7.26–7.34 (m, 5H), 7.36 (d, J = 7.6 Hz, 2H); 13C NMR (75 MHz, CDCl3): δ 65.2, 68.9, 115.1 (2C), 124.5, 126.7, 128.1 (2C), 128.7 (2C), 128.8 (2C), 133.2, 136.6, 158.4; anal. calcd for C16H16O2: C 79.97, H 6.71; found: C 80.06, H 6.68%.
2-(4-(Cinnamyloxy)-phenyl)-acetonitrile (Table 3, 3k). White solid; IR (KBr): 3406, 2924, 2853, 2251, 1953, 1731, 1612, 1512, 1462, 1247, 1014 cm−1; 1H NMR (400 MHz, CDCl3): δ 3.62 (s, 2H), 4.63 (d, J = 5.6 Hz, 2H), 6.31–6.35 (m, 1H), 6.66 (d, J = 16 Hz, 1H), 6.89 (d, J = 8.4 Hz, 2H), 7.16–7.35 (m, 7H); 13C NMR (75 MHz, CDCl3): δ 22.8, 68.7, 114.0, 115.4 (2C), 118.1, 122.0, 124.1, 126.6 (2C), 127.9, 128.6 (2C), 129.1 (2C), 133.3, 158.4; anal. calcd for C17H15NO: C 81.90, H 6.06, N 5.62; found: C 81.88, H 6.11, N 5.66%.
7-(3-Phenyl-allyloxy)-quinoline (Table 3, 3m). Dirty white solid; IR (KBr): ν 3382, 3066, 2959, 2863, 2752, 2682, 1634, 1598, 1442, 1381, 1221, 1040 cm−1; 1H NMR (400 MHz, CDCl3): δ 4.69 (d, J = 6 Hz, 2H), 6.36–6.41 (m, 1H), 6.68 (d, J = 16 Hz, 1H), 7.27–7.48 (m, 7H), 7.68 (d, J = 5.6 Hz, 1H), 7.83 (d, J = 8.8 Hz, 1H), 8.28 (d, J = 5.6 Hz, 1H), 9.09 (s, 1H); 13C NMR (75 MHz, CDCl3): δ 64.7, 108.3, 120.6, 123.7, 124.2, 126.5, 126.9 (2C), 128.4, 128.6, 129.0, 129.1 (2C), 131.1, 133.4, 140.3, 150.8, 156.7; anal. calcd for C18H15NO: C 82.73, H 5.79, N 5.36; found: C 81.78, H 5.66, N 5.41%.
(E)-1-Bromo-4-((3-(p-tolyl)-allyl)-oxy)-benzene (Table 3, 3n). White solid; IR (KBr): 3375, 2933, 2863, 1742, 1650, 1606, 1509, 1463, 1380, 1287, 1241, 1175, 1105, 1063, 1032 cm−1; 1H NMR (400 MHz, CDCl3): δ 1.97 (s, 3H), 4.58 (d, J = 6.0 Hz, 2H), 6.26 (m, 1H), 6.61 (d, J = 16.0 Hz, 1H), 6.76 (d, J = 8.8 Hz, 2H), 7.06 (d, J = 8.0 Hz, 2H), 7.23 (d, J = 8.0 Hz, 2H), 7.29–7.32 (m, 2H); 13C NMR (75 MHz, CDCl3): δ 21.8, 68.9, 114.0, 116.6 (2C), 122.8, 126.5, 126.6 (2C), 129.3 (2C), 132.2 (2C), 133.5, 137.9, 157.7; anal. calcd for C16H15BrO: C 63.38, H 4.99; found: C 63.41, H 4.83%.
1-(4-Bromocinnamyloxy)-4-bromobenzene (Table 3, 3r). White solid; IR (KBr): 3368, 3081, 2932, 2854, 1780, 1604, 1591, 1496, 1463, 1378, 1290, 1175, 1117 cm−1; 1H NMR (400 MHz, CDCl3): δ 4.65 (d, J = 4.4 Hz, 2H), 6.36–6.39 (m, 1H), 6.66 (d, J = 16 Hz, 1H), 6.83 (dd, J1 = 2 Hz, J2 = 4.8 Hz, 2H), 7.27 (d, J = 7.6 Hz, 2H), 7.38 (d, J = 4.8 Hz, 2H), 7.45 (d, J = 6.8 Hz, 2H); 13C NMR (75 MHz, CDCl3): δ 67.6, 113.0, 115.5, 120.8, 123.7, 127.7, 127.1, 130.7, 130.9, 131.3, 138.3, 156.7; anal. calcd for C15H12Br2O: C 48.95, H 3.29; found: C 48.88, H 3.34%.
1-(2-Methoxycinnamyloxy)-4-methyl benzene (Table 3, 3s). Pale yellow solid; IR (KBr): 3397, 3031, 2958, 2914, 2840, 2052, 1895, 1655, 1606, 1510, 1461, 1383, 1328, 1283, 1241, 1177, 1111, 1031 cm−1; 1H NMR (400 MHz, CDCl3): δ 2.21 (s, 3H), 3.77 (s, 3H), 4.59 (d, J = 0.8 Hz, 2H), 6.33–6.4 (m, 1H), 6.78–6.87 (m, 4H), 6.95–7.02 (m, 3H), 7.14–7.18 (m, 1H), 7.39 (dd, J1 = 1.2 Hz, J2 = 7.6 Hz, 1H); 13C NMR (75 MHz, CDCl3): δ 22.3, 55.0, 68.9, 110.5, 114.3 (2C), 120.2, 125.0, 125.2, 126.8, 127.7, 128.5, 129.6 (2C), 129.5, 156.3, 156.4; anal. calcd for C17H18O2: C 80.28, H 7.13; found: C 80.67, H 7.11%.
(2E)-3-(4-(Cinnamyloxy)-phenyl)-1-phenylprop-2-en-1-one (Table 3, 3t). White solid; IR (KBr): 3393, 3026, 2923, 2853, 1904, 1660, 1631, 1601, 1509, 1445, 1381, 1333, 1290, 1248, 1178, 1113, 1070, 1008 cm−1; 1H NMR (400 MHz, CDCl3): δ 4.76 (dd, J1 = 1.2 Hz, J2 = 6 Hz, 2H), 6.38–6.45 (m, 1H), 6.75 (d, J = 16 Hz, 1H), 6.99 (d, J = 8.8 Hz, 2H), 7.26–7.28 (m, 1H), 7.32–7.36 (m, 2H), 7.41–7.44 (m, 3H), 7.48–7.52 (m, 2H), 7.56–7.58 (m, 1H), 7.59–7.63 (m, 2H), 7.79 (d, J = 15.6 Hz, 1H), 8.00–8.02 (m, 2H); 13C NMR (75 MHz, CDCl3): δ 68.7, 115.2 (2C), 119.9, 123.7, 126.6 (2C), 127.8, 128.0, 128.3 (2C), 128.4 (2C), 128.5 (2C), 130.2 (2C), 132.5, 133.4, 136.2, 138.5, 144.6, 160.7, 190.5; HR-MS (EI): m/z = 341.1542 (M + H+), calcd for C24H20O2: 341.1540.
1-Chloro-4-(3-phenyl-allyloxymethyl)-benzene (Scheme 4, 5a). Yellow viscous liquid; IR (neat): 3355, 3050, 2952, 2920, 2760, 1911, 1605, 1490, 1468, 1360, 1298, 1288, 1274, 1109, 1085 cm−1; 1H NMR (400 MHz, CDCl3): δ 4.26 (d, J = 4.4 Hz, 2H), 4.59 (s, 2H), 6.27–6.34 (m, 1H), 6.56 (d, J = 16.0 Hz, 1H), 7.21–7.36 (m, 9H); 13C NMR (75 MHz, CDCl3): δ 63.0, 63.7, 126.0 (2C), 127.3, 127.8 (2C), 127.9, 128.1 (4C), 130.7, 132.8, 136.3, 138.9; anal. calcd for C16H15ClO: C 74.27, H 5.84; found: C 74.21, H 5.66%.
4 Conclusions
In conclusion, we have demonstrated the synthesis of polymer supported air and moisture stable, oxygen-insensitive and reusable palladium(II) catalyst and its application for the O-allylation of allyl/cinnamyl acetates with substituted phenol under refluxing condition in water. Ease of recovery and reuse of the catalyst make this method an economic and environmentally-benign process. In addition, high yields, excellent stereo-specificity, use of water as solvent, scope of wide functionalization and simple work-up procedure make this method more attractive and valuable to the existing methodologies for allylic C–O coupling reactions.
Acknowledgements
SA thanks Prof. Brindaban C. Ranu of the Indian Association for the Cultivation of Science, Kolkata and Prof. Abu T. Khan of the Aliah University, Kolkata for his support. SMI gratefully acknowledges Department of Science and Technology (DST), New Delhi, India for funding (Project No. SB/S1/PC-107/2012 Dated: 10.06.2013). We acknowledge DST and UGC for providing support to the University of Kalyani under FIST, Purse and SAP programme. M. H. acknowledges Dr Kamalika Sen and Dr Anupam Singha Roy for his entire help and expresses sincere thanks to the UGC for providing necessary fellowship. MMI acknowledges UGC, New Delhi for Maulana Azad National Fellowship.
Notes and references
-
(a) J. Tsuji, Palladium Reagents and Catalysts. New Perspectives for the 21st Century, Wiley, Chichester, 2004 Search PubMed;
(b) E. Negishi and A. de Meijre, Organopalladium Chemistry for Organic Synthesis, Wiley, New York, 2002 Search PubMed;
(c) J. Hartwig, Organotransition Metal Chemistry, University Science Books, Sausalito, CA, 2010 Search PubMed;
(d) N. Miyaura and A. Suzuki, Chem. Rev., 1995, 95, 2457 CrossRef CAS;
(e) N. Selander and K. J. Szabo, Chem. Rev., 2011, 111, 2048–2076 CrossRef CAS PubMed;
(f) T. W. Lyons and M. S. Sanford, Chem. Rev., 2010, 110, 1147–1169 CrossRef CAS PubMed.
-
(a) M. J. Climent, A. Corma and S. Iborra, RSC Adv., 2012, 2, 16–58 RSC;
(b) N. Mizuno and M. Misono, Chem. Rev., 1998, 98, 199–217 CrossRef CAS PubMed;
(c) J. Lu and P. H. Toy, Chem. Rev., 2009, 109, 815–838 CrossRef CAS PubMed.
-
(a) P. A. Grieco, Organic Synthesis in Water, Blackie Academic and Professional, London, 1998 Search PubMed;
(b) Z. P. Demko and K. B. Sharpless, J. Org. Chem., 2001, 66, 7945 CrossRef CAS PubMed;
(c) C. J. Lee, Chem. Rev., 2005, 105, 3095 CrossRef PubMed.
-
(a) B. M. Trost, J. Am. Chem. Soc., 1979, 101, 6432–6433 CrossRef CAS;
(b) Y. Uozumi, H. Danjo and T. Hayashi, Tetrahedron Lett., 1997, 38, 3557–3560 CrossRef CAS;
(c) M. Zecca, R. Fisera, G. Palma, S. Lora, M. Hronec and M. Kra'lik, Chem.–Eur. J., 2000, 6, 1980–1986 CrossRef CAS PubMed;
(d) C. A. Parrish and S. L. Buchwald, J. Org. Chem., 2001, 66, 3820–3827 CrossRef CAS PubMed;
(e) Y. Niu, L. K. Yeung and R. M. Crooks, J. Am. Chem. Soc., 2001, 123, 6840–6846 CrossRef CAS;
(f) A. M. Jansson, M. Grotli, K. M. Halkes and M. Meldal, Org. Lett., 2002, 4, 27–30 CrossRef CAS PubMed.
- S. M. Islam, A. S. Roy, P. Mondal and N. Salam, Appl. Organomet. Chem., 2012, 26, 625–634 CrossRef CAS.
-
(a) A. S. Roy, J. Mondal, B. Banerjee, P. Mondal, A. Bhaumik and S. M. Islam, Appl. Catal., A, 2014, 469, 320–327 CrossRef CAS;
(b) S. M. Islam, S. Mondal, P. Mondal, A. S. Roy, K. Tuhina, M. Mobarok, S. Paul, N. Salam and D. Hossain, Catal. Lett., 2011, 141, 1171–1181 CrossRef.
- S. M. Islam, A. S. Roy, P. Mondal and N. Salam, J. Mol. Catal. A: Chem., 2012, 358, 38–48 CrossRef CAS.
-
(a) J. Buckingham, Dictionary of Natural Products, University Press, Cambridge, MA, 1994 Search PubMed;
(b) B. M. Trost and P. Renaud, J. Am. Chem. Soc., 1982, 104, 6668–6672 CrossRef CAS;
(c) B. M. Trost and J. P. Genêt, J. Am. Chem. Soc., 1976, 98, 1816–1817 Search PubMed.
-
(a) J. Tsuji, in Handbook of Organopalladium Chemistry for Organic Synthesis, ed. E. Negishi, Wiley, New York, 2002, pp. 1669–1687, vol. 5 Search PubMed;
(b) B. M. Trost and D. L. VanVranken, Chem. Rev., 1996, 96, 395–422 CrossRef CAS PubMed;
(c) S. A. Godleski, in Comprehensive Organic Synthesis, ed. B. M. Trost and I. Fleming, Pergamon, Oxford, 1991, pp. 585–661, vol. 4 Search PubMed.
-
(a) A. Monte, M. S. Kabir, J. M. Cook, M. Rott, W. R. Schwan and L. Defoe, U. S. Pat. Appl. Publ., 2007, 37, 60 Search PubMed;
(b) O. E. O. Hormi and L. Hirvela, Tetrahedron Lett., 1993, 34, 6463–6466 CrossRef CAS;
(c) D. Gopal and K. Rajagopalan, Tetrahedron Lett., 1987, 28, 5327–5330 CrossRef CAS.
- S. Tanaka, H. Saburi, Y. Ishibashi and M. Kitamura, Org. Lett., 2004, 6, 1873–1875 CrossRef CAS PubMed.
-
(a) A. Heumann, in Transition Metals for Organic Synthesis, ed. M. Beller and C. Bolm, Wiley-VCH, Weinheim, Germany, 2004, p. 251, vol. 1 Search PubMed;
(b) B. M. Trost and T. S. Scanlan, Tetrahedron Lett., 1986, 27, 4141–4144 CrossRef CAS;
(c) B. M. Trost and D. L. van Vranken, Chem. Rev., 1996, 96, 395–422 CrossRef CAS PubMed;
(d) M. Johannsen and K. A. Jorgensen, Chem. Rev., 1998, 98, 1689–1708 CrossRef CAS PubMed;
(e) Y. Yatsumonji, Y. Ishida, A. Tsubouchi and T. Takeda, Org. Lett., 2007, 9, 4603–4606 CrossRef CAS PubMed;
(f) C. Juslen and T. Enkvist, Acta Chem. Scand., 1958, 12, 287–297 CrossRef CAS;
(g) B. C. Ranu and R. Jana, Adv. Synth. Catal., 2005, 347, 1811–1818 CrossRef CAS;
(h) S. Vijaikumar and K. Pitchumani, J. Mol. Catal. A: Chem., 2004, 217, 117–120 CrossRef CAS;
(i) R. N. Salvatore, R. A. Smith, A. K. Nischwitz and T. Gavin, Tetrahedron Lett., 2005, 46, 8931–8935 CrossRef CAS;
(j) R. S. Varma and T. I. Reddy, Chem. Commun., 1997, 621–622 Search PubMed.
- B. M. Trost and T. R. Verhoeven, J. Am. Chem. Soc., 1980, 102, 4730–4743 CrossRef CAS.
-
(a) J. Tsuji, Transition Metal Reagents and Catalysts, Wiley, New York, 2000 Search PubMed;
(b) S. A. Godleski, in Comprehensive Organic Synthesis, ed. B. M. Trost and I. Fleming, Pergamon, Oxford, 1991, p. 585, vol. 4. Search PubMed;
(c) M. Shibasaki, in Advances in Metal-Organic Chemistry, ed. L. S. Liebeskind, JAI, Greenwich, CT, 1996, vol. 5 Search PubMed;
(d) P. J. Harrington, in Comprehensive Organometallic Chemistry II, ed. E. W. Abel, F. G. A. Stone and G. Wilkinson, Pergamon, Oxford, 1995, pp. 798–903, vol. 12 Search PubMed;
(e) Comprehensive Asymmetric Catalysis, ed. E. N. Jacobsen, A. Pfaltz and H. Yamamoto, Springer, Berlin, 1999, vol. 2 Search PubMed;
(f) J. Tsuji, Palladium Reagents and Catalysts: Innovations, in Organic Synthesis, John Wiley and Sons, New York, 1995 Search PubMed.
-
(a) B. M. Trost, R. Madsen, S. D. Guile and B. Brown, J. Am. Chem. Soc., 2000, 122, 5947–5956 CrossRef CAS;
(b) T. M. Pedersen, E. L. Hansen, J. Kane, T. Rein, P. Helquist, P.-O. Norrby and D. Tanner, J. Am. Chem. Soc., 2001, 123, 9738–9742 CrossRef CAS PubMed;
(c) M. E. Krafft, A. M. Wilson, Z. Fu, M. J. Procter and O. A. Dasse, J. Org. Chem., 1998, 63, 1748–1749 CrossRef CAS;
(d) H. Nakamura, K. Aoyagi, J.-G. Shim and Y. Yamamoto, J. Am. Chem. Soc., 2001, 123, 372–377 CrossRef CAS.
-
(a) S. L. You, X. Z. Zhu, Y. M. Luo, X. L. Hou and L. X. Dai, J. Am. Chem. Soc., 2001, 123, 7471–7472 CrossRef CAS PubMed;
(b) T. Konno, K. Nagata, T. Ishihara and H. Yamanaka, J. Org. Chem., 2002, 67, 1768–1775 CrossRef CAS PubMed;
(c) L. F. Tietze, H. Schirok, M. Wohrmann and K. Schrader, J. Org. Chem., 2000, 65, 2433–2444 Search PubMed;
(d) M.-C. Liao, X.-H. Duan and Y.-M. Liang, Tetrahedron Lett., 2005, 46, 3469–3472 CrossRef CAS.
-
(a) S. Ahammed, A. Saha and B. C. Ranu, RSC Adv., 2012, 2, 6329–6335 RSC;
(b) Y. Yatsumonji, Y. Ishida, A. Tsubouchi and T. Takeda, Org. Lett., 2007, 9, 4603–4606 CrossRef CAS PubMed;
(c) S. Divekar, M. Safi, M. Soufiaoui and D. Sinou, Tetrahedron, 1999, 55, 4369–4376 CrossRef CAS;
(d) T. Ishiyama, M. Mori, A. Suzuki and N. Miyaura, J. Organomet. Chem., 1996, 525, 225–232 CrossRef CAS;
(e) H. Danjo, D. Tanaka, T. Hayashi and Y. Uozumi, Tetrahedron, 1999, 55, 14341–14352 CrossRef CAS.
- A. W. Williamson, J. Chem. Soc., 1852, 4, 229–239 Search PubMed.
-
(a) R. Akiyama and S. Kobayashi, J. Am. Chem. Soc., 2003, 125, 3412–3413 CrossRef CAS PubMed;
(b) T. Satoh, M. Ikeda, M. Miura and M. Nomura, J. Org. Chem., 1997, 62, 4877–4879 CrossRef CAS;
(c) C. Goux, M. Massacret, P. Lhoste and D. Sinou, Organometallics, 1995, 14, 4585–4593 CrossRef CAS;
(d) Y. Kayaki, T. Koda and T. Ikariya, J. Org. Chem., 2004, 69, 2595–2597 CrossRef CAS PubMed;
(e) H. Kim and C. Lee, Org. Lett., 2002, 4, 4369–4371 CrossRef CAS PubMed;
(f) F. L. Lam, T. T.-L. Au-Yeung, F. Y. Kwong, Z. Zhou, K. Y. Wong and A. S. C. Chan, Angew. Chem., Int. Ed., 2008, 47, 1280–1283 CrossRef CAS PubMed.
-
(a) H. Saburi, S. Tanaka and M. Kitamura, Angew. Chem., Int. Ed., 2005, 44, 1730–1732 CrossRef CAS PubMed;
(b) Z. Sahli, N. Derrien, S. Pascal, B. Demerseman, T. Roisnel, F. Barriere, M. Achard and C. Bruneau, Dalton Trans., 2011, 40, 5625–5630 RSC;
(c) J. A. v. Rijn, E. v. Stapele, E. Bouwman and E. Drent, J. Catal., 2010, 272, 220–226 CrossRef;
(d) R. C. v. d. Drift, M. Vailati, E. Bouwman and E. Drent, J. Mol. Catal. A: Chem., 2000, 159, 163–177 CrossRef;
(e) J. A. v. Rijn, M. A. Siegler, A. L. Spek, E. Bouwman and E. Drent, Organometallics, 2009, 28, 7006–7014 CrossRef;
(f) M. Austeri, D. Linder and J. Lacour, Adv. Synth. Catal., 2010, 352, 3339–3347 CrossRef CAS.
- H. Nakagawa, T. Hirabayashi, S. Sakaguchi and Y. Ishii, J. Org. Chem., 2004, 69, 3474–3477 CrossRef CAS PubMed.
- Y. Yatsumonji, Y. Ishida, A. Tsubouchi and T. Takeda, Org. Lett., 2007, 9, 4603–4606 CrossRef CAS PubMed.
-
(a) A. Saha, J. Leazer and R. S. Varma, Green Chem., 2012, 14, 67–71 RSC;
(b) R. B. N. Baig and R. S. Varma, Ind. Eng. Chem. Res., 2014, 53, 18625–18629 CrossRef.
- M. A. Zolfigol, V. Khakyzadeh, A. R. Eza Moosavi-Zare, A. Rostami, A. Zare, N. Iranpoor, M. H. Beyzavie and R. Luque, Green Chem., 2013, 15, 2132–2140 RSC.
- M. D. Angelino and P. E. Laibinis, Macromolecules, 1998, 31, 7581–7587 CrossRef CAS.
- P. A. Stephenson and G. Wilkinson, J. Inorg. Nucl. Chem., 1967, 29, 2122–2123 CrossRef.
-
(a) T.-W. Tsai, E.-C. Wang, S.-R. Li, Y.-H. Chen, Y.-L. Lin, Y.-F. Wang and K.-S. Huang, J. Chin. Chem. Soc., 2004, 51, 1307–1318 CrossRef CAS;
(b) W. Wang, R. Zhou, Z.-J. Jiang, K. Wang, H.-Y. Fu, X.-L. Zheng, H. Chen and R.-X. Li, Adv. Synth. Catal., 2014, 356, 616–622 CrossRef CAS;
(c) E. Lindstedt, R. Ghosh and B. Olofsson, Org. Lett., 2013, 15, 6070–6073 CrossRef CAS PubMed;
(d) F. Carta, A. Maresca, A. Scozzafava and C. T. Supuran, Bioorg. Med. Chem., 2012, 20, 2266–2273 CrossRef CAS PubMed;
(e) K. Watanabe, T. Mino, T. Abe, T. Kogure and M. Sakamoto, J. Org. Chem., 2014, 79, 6695–6702 CrossRef CAS PubMed;
(f) T. Mino, H. Shindo, T. Kaneda, T. Koizumi, Y. Kasashima, M. Sakamoto and T. Fujita, Tetrahedron Lett., 2009, 50, 5358–5360 CrossRef CAS.
Footnote |
† Electronic supplementary information (ESI) available: Copies of 1H NMR and 13C NMR spectra of all products listed in Table 2, Schemes 3–5. See DOI: 10.1039/c5ra26182a |
|
This journal is © The Royal Society of Chemistry 2016 |