DOI:
10.1039/C5RA26106F
(Communication)
RSC Adv., 2016,
6, 13027-13031
Inherently chiral heterocyclic resorcinarenes using a Diels–Alder reaction†
Received
7th December 2015
, Accepted 21st January 2016
First published on 26th January 2016
Abstract
This paper presents a novel approach to highly diastereoselective synthesis of resorcinarenes having enlarged cavities. Inherently chiral heterocyclic resorcinarenes have been obtained with unusually high diastereoselecivity by a “one pot” sequence involving thermal generation of o-quinomethide resorcinarene derivatives and the subsequent Diels–Alder reaction with dienophiles (exemplified by α-methylstyrene). The diastereoselectivity of this reaction is explained based on the thermodynamic stability of the products. The enantiomers of rac-4 have been separated by HPLC and their absolute configuration was assigned by comparison of their experimental and theoretical CD spectra.
Resorcinarenes and their derivatives are found among key macrocyclic compounds that have contributed to main developments and applications of supramolecular chemistry. In comparison to many other macrocycles, resorcinarene derivatives emerge as particularly convenient building blocks for formation of chiral structures, owing to their unique structural and conformational features.1,2 Chiral resorcinarenes have been widely employed, namely: for monitoring the formation of host–guest type complexes,3 preparing catalysts with catalytic stages occurring within the cavity,4 forming large molecular containers for transporting molecules and ions,5 as chiral agents for discrimination of enantiomers,6 as enzyme models,7 and also as chiral stationary phases in chromatography.8 Chiral resorcinarene derivatives can be obtained in several ways including classical modifications with chiral auxiliaries or by, utilized to a much less extent thus far, generation of inherent chirality (also called cyclochirality).2,9 The latter approach has been implemented by simultaneous modification of one of the hydroxyl groups and the central ortho position of resorcinol units leading to heterocyclic resorcinarenes that exhibit inherent chirality due to introduced directionality of the concave skeleton. At present, only few classes of inherently chiral resorcinarenes are known including oxazines,10 boronooxazino-oxazolidines,11 iminoresorcinarenes,12 mono-13 and tetra-substituted14 derivatives of resorcinarenes. The possibility of employing the Diels–Alder reaction for formation of a heterocyclic ring and building inherently chiral resorcinarenes has not been described in the literature yet.
Here we report the use of Diels–Alder reaction for highly regio- and diastereoselective preparation of a new class of heterocyclic resorcinarene derivatives – benzopyrane resorcinarenes rac-4 – using in situ generated resorcinarene diene 2 (Scheme 1). We analyse the possible reasons for such selectivity, separate inherently chiral enantiomers and assign their absolute configurations. The vast potential present in this approach allows not only for the synthesis of macrocycles with a broad array of various substituents, but also for a significant extension of the resorcinarene skeleton.
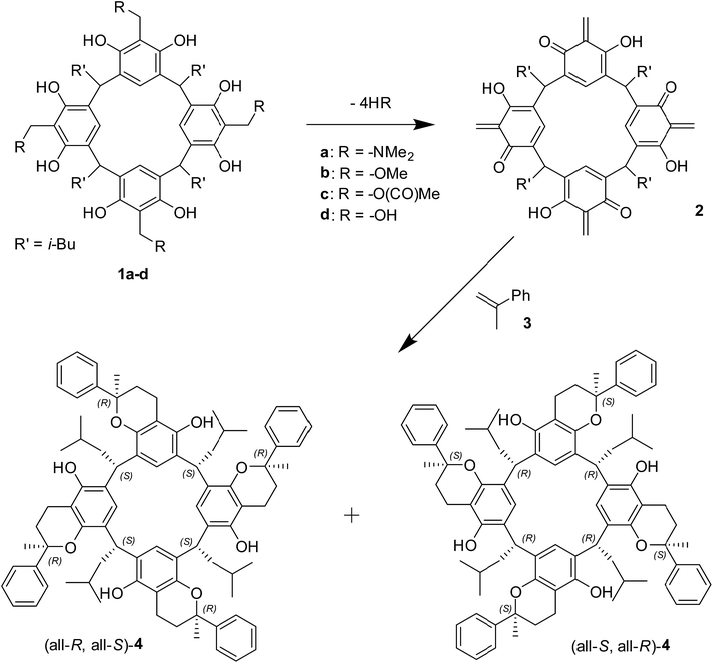 |
| Scheme 1 Cycloaddition reaction of resorcinarene derivatives. | |
The presence of highly reactive o-quinomethine intermediates has been postulated in many chemical reactions leading to the synthesis of natural products.15 The methods that has been used to generate o-quinomethines include thermolysis, oxidation, photolysis, or β-elimination reactions assisted with acids or bases.16 The major pathways of consecutive reactions of o-quinomethines are: (1) cycloaddition leading to benzopyrane derivatives; (2) 1,4-Michael nucleophilic addition leading to o-substituted phenols; (3) 1,5-sigmatropic shift leading to styrenes.17 Here, we have assumed that resorcinarene derivatives 1a–1d may potentially form transition intermediates having the o-quinomethide structure 2 that can further participate in cycloaddition reactions, for example with α-methylstyrene 3.
We have found that reaction of 1a with 3 under thermal conditions proceeds smoothly and gives the main product in a high yield (Table 1, entry 1), that can be isolated by simple precipitation/crystallization procedure. It is important to note that the Diels–Alder reaction of resorcinarene derivatives 1a with 3 can lead to formation of numerous isomers due to simultaneous generation of eight new stereogenic centres (absolute configuration marked in Scheme 1).
Table 1 Synthesis of rac-4via Diels–Alder reaction of resorcinarene derivatives with α-methylstyrene 3
Substrate |
Solvent |
T, °C |
Reaction time, h |
Yield of rac-4, % |
1a
|
3
|
140 |
7 |
88 |
1a
|
Chlorobenzene |
130 |
7 |
4 |
1b
|
3
|
120 |
3 |
70 |
1c
|
3
|
120 |
3 |
13 |
1d
|
3
|
120 |
3 |
8 |
The statistical abundance of all C4-symmetric isomers (four isomers) is 4/28 (statistical yield 1.6%). In the current reaction only a single diastereoisomer, rac-4, was isolated in yields up to 88%, meaning that the amplification of formation of rac-4 is ca. 110 times (88% vs. statistical yield 0.8%). The X-ray structure of rac-4 (Fig. 1) shows that the relative configuration of the amplified diastereoisomer is (all-R, all-S). Rac-4 exhibits a crown conformation stabilised by four intramolecular hydrogen bonds. Interestingly, bulky phenyl groups originating from α-methylstyrene point towards the interior of the resorcinarene cavity. The phenyl groups are involved in C–H⋯π interactions (Fig. 1b) that can be considered a favourable stabilizing force. They also enclose a small space inside the cavity which seems not accessible for any kind of guest molecules (Fig. 1c).
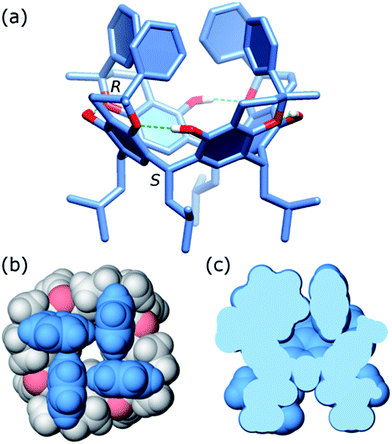 |
| Fig. 1 X-ray structure of rac-4: (a) side view in a stick representation showing relative configuration (all-R, all-S); (b) top view in a van der Waals representation, blue-phenyl rings forming C–H⋯π interactions; (c) slice through a van der Waals representation of the structure. | |
The best yield of rac-4 was obtained by subjecting the N,N-dimethylamino derivative 1a to a prolonged heating (7 h) at high temperature in α-methylstyrene solution. Analogous reactions of 1a with α-methylstyrene 3 in chlorobenzene lead to a lower yield of about 4%. In the case of derivatives 1b–1d, heating above 120 °C leads to partial formation of insoluble precipitates. This may result from the polymerisation of the resorcinarene derivatives used for these reactions, which would lower the yield of rac-4. Indeed, a test reaction has shown that 1b alone upon heating above 120 °C, forms an insoluble yellow precipitate.
In order to rationalize the fact that only one diastereoisomer is preferred, we considered the possibility, that under high temperature conditions the most thermodynamically stable products are amplified. For resorcinarene derivatives, presence of four intramolecular hydrogen bonds usually leads to stabilization of C4 symmetric structures. Therefore, we calculated the heat of formation (ΔH) only for the two possible C4-symmetric diastereoisomers (all-R, all-S) and (all-S, all-S) using the semi-empirical PM7 method implemented in MOPAC2012 software.18 The results confirmed that indeed (all-R, all-S) diastereoisomer is thermodynamically more stable (ΔH = −378.80 kcal mol−1) than (all-S, all-S) diastereoisomer (ΔH = −356.30 kcal mol−1, see Fig. S8† for full computational details).
Rac-4, the amplified product of the reaction, is formed as a mixture of two enantiomers (all-R, all-S)-4 and (all-S, all-R)-4. This was confirmed by separation of enantiomers using chiral HPLC (Fig. 2a). The CD spectra, that have been recorded for both fractions, exhibit mirror-image relationship (Fig. 2b) confirming the enantiomeric relationship between compounds. To assign the absolute configuration of enantiomers of 4 we have used quantum mechanical calculations (TD-DFT method at B3LYP/6-31G* level) for the model compound (S)-2,6,8-trimethyl-2-phenylchroman-5-ol (Fig. 2c).19 We have compared the theoretical ECD spectrum to the experimental spectra for both enantiomers. A good agreement of the theoretical ECD spectrum with the experimental spectrum of first eluting enantiomer allows us to assume that it has a (all-S, all-R) configuration (Fig. 2d).
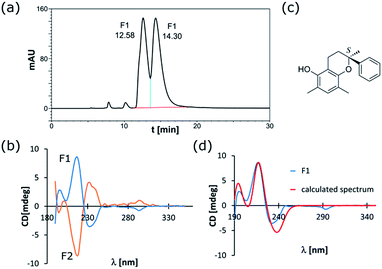 |
| Fig. 2 (a) HPLC separation of enantiomers of rac-4 (Nucleocel Delta S column from Macherey&Nagel); (b) CD spectra of enantiomers (blue – enantiomer having the shorter retention time, F1); (c) structure of (S)-2,6,8-trimethyl-2-phenylchroman-5-ol; (d) assignment of the absolute configurations using comparison of experimental and theoretical results (TD DFT B3LYP/6-31G*). | |
Although the existence of reactive o-quinomethide intermediate 2 seems to be a plausible explanation of participation of 1a–1d in the Diels–Alder reaction, we undertook an additional effort to prove their existence. The ESI-MS/MS spectra for both, the substrate 1a (Fig. 3) and the product rac-4, show the sequential appearance of the intermediates containing various number of o-quinomethide units in their structure. This observation supports the presence of o-quinomethide intermediates during the course of reaction.
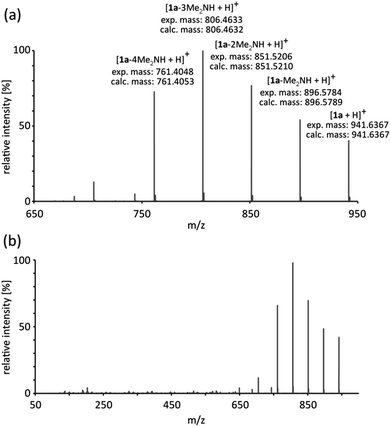 |
| Fig. 3 (a) ESI-MS spectrum of 1a demonstrating the sequential formation of o-quinomethide derivatives through gradual release of dimethylamine fragments (calculated and experimental high resolution m/z values are given); (b) the full measured range of the spectrum. | |
Conclusions
In conclusion, the paper presents a novel approach which allows for highly diastereoselective synthesis of chiral heterocyclic derivatives of resorcinarenes. Making use of the possibility of generating the o-quinomethide intermediates and their subsequent reactions with dienophiles opens new opportunities for obtaining resorcinarenes with various functionalities and new stereochemical features. The Diels–Alder reaction is one of the most widely studied and useful reactions for generation of carbon–carbon bonds as well as new stereogenic centres. Furthermore, a great number of substrates and chiral catalysts for this reaction are already known. Considering these facts we expect that our findings may open up new possibilities in the chemistry of resorcinarenes.
Acknowledgements
We acknowledge the financial support of the National Science Centre-Poland (Grant No. 2012/05/B/ST5/00306, 2014/13/N/-ST5/01765) and the Ministry of Science and Higher Education – Poland (Grant No. 612412).
Notes and references
-
(a) P. Timmerman, W. Verboom and D. N. Reinhoudt, Tetrahedron, 1996, 52, 2663 CrossRef CAS;
(b) M. Urbaniak and W. Iwanek, Tetrahedron, 2006, 62, 1508 CrossRef CAS;
(c) W. Iwanek, Ch. Wolff and J. Mattay, Tetrahedron Lett., 1995, 36, 8469 CrossRef;
(d) A. Shivanyuk, Ch. Schmidt, V. Boehmer, E. F. Paulus, O. Lukin and W. Vogt, J. Am. Chem. Soc., 1998, 120, 4319 CrossRef CAS;
(e) C. Schmidt, K. Airola, V. Boehmer, W. Vogt and K. Rissanen, Tetrahedron, 1997, 53, 17691 CrossRef CAS.
-
(a) A. Szumna, Chem. Soc. Rev., 2010, 39, 4274 RSC;
(b) M. J. McIldowie, M. Mocerino and M. I. Ogden, Supramol. Chem., 2010, 22, 13 CrossRef CAS.
-
(a) Z. Qi, T. Heinrich, S. Moorthy and Ch. A. Schalley, Chem. Soc. Rev., 2015, 44, 515 RSC;
(b) F. Pan, N. K. Beyeh and K. Rissanen, RSC Adv., 2015, 5, 57912 RSC;
(c) N. K. Beyeh, F. Pan, A. Valkonen and K. Rissanen, CrystEngComm, 2015, 17, 1182 RSC;
(d) M. Hong, Y. M. Zhang and Y. Liu, J. Org. Chem., 2015, 80, 1849 CrossRef CAS PubMed;
(e) N. K. Beyeh and R. Puttreddy, Dalton Trans., 2015, 44, 9881 RSC;
(f) K. Helttunen, T. R. Tero and M. Nissinen, CrystEngComm, 2015, 17, 3667 RSC;
(g) T. Sakano, T. Ohashi, M. Yamanaka and K. Kobayashi, Org. Biomol. Chem., 2015, 13, 8359 RSC;
(h) N. N. Valand, M. B. Patel and S. K. Menon, RSC Adv., 2015, 5, 8739 RSC.
-
(a) S. Giust, G. La Sorella, L. Sperni, F. Fabris, G. Strukul and A. Scarso, Asian J. Org. Chem., 2015, 4, 217 CrossRef CAS;
(b) T. Chavagnan, D. Sémeril, D. Matt, J. Harrowfield and L. Toupet, Chem.–Eur. J., 2015, 21, 6678 CrossRef CAS PubMed;
(c) A. Zhang and K. Tiefenbacher, Nat. Chem., 2015, 7, 197 CrossRef PubMed;
(d) G. La Sorella, L. Sperni, G. Strukul and A. Scarso, ChemCatChem., 2015, 7, 291 CrossRef CAS;
(e) A. Mouradzadegun, F. Abadast and S. Elahi, Monatsh. Chem., 2014, 145, 1663 CrossRef CAS;
(f) A. Mouradzadegun, S. Elahi and F. Abadast, RSC Adv., 2014, 4, 31239 RSC.
-
(a) P. Ballester, M. Fujita and J. Rebek Jr, Chem. Soc. Rev., 2015, 44, 392 RSC;
(b) S. Zarra, D. M. Wood, D. A. Roberts and J. R. Nitschke, Chem. Soc. Rev., 2015, 44, 419 RSC;
(c) V. Guralnik, L. Avram and Y. Cohen, Org. Lett., 2014, 16, 5592 CrossRef CAS PubMed;
(d) T. Sakano, T. Ohashi, M. Yamanaka and K. Kobayashi, Org. Biomol. Chem., 2015, 13, 8359 RSC;
(e) E. Mileo, S. Yi, P. Bhattacharya and A. E. Kaifer, Angew. Chem., Int. Ed., 2009, 48, 5337 CrossRef CAS PubMed.
-
(a) N. H. Pham and T. J. Wenzel, Chirality, 2012, 24, 193 CrossRef CAS PubMed;
(b) C. M. O'Farrell, J. M. Chudomel, J. M. Collins, C. F. Dignam and T. J. Wenzel, J. Org. Chem., 2008, 73, 2843 CrossRef PubMed;
(c) T. J. Wenzel, J. Inclusion Phenom. Macrocyclic Chem., 2014, 78, 1 CrossRef CAS.
-
(a) N. J. Rebilly and O. Reinaud, Supramol. Chem., 2014, 26, 454 CrossRef;
(b) A. Višnjevac, J. Gout, N. Ingert, O. Bistri and O. Reinaud, Org. Lett., 2010, 12, 2044 CrossRef PubMed;
(c) M. Raynal, P. Ballester, A. Vidal-Ferran and P. W. N. van Leeuwen, Chem. Soc. Rev., 2014, 43, 1734 RSC;
(d) T. K. Ronson, S. Zarra, S. P. Black and J. R. Nitschke, Chem. Commun., 2013, 49, 2476 RSC;
(e) V. Montes-García, J. Pérez-Juste, I. Pastoriza-Santos and L. M. Liz-Marzán, Chem.–Eur. J., 2014, 20, 10874 CrossRef PubMed.
-
(a) N. Li, R. G. Harrison and J. D. Lamb, J. Inclusion Phenom. Macrocyclic Chem., 2014, 78, 39 CrossRef CAS;
(b) T. Sokolies, U. Menyes, U. Roth and T. Jira, J. Chromatogr. A, 2002, 948, 309 CrossRef;
(c) A. Ruderisch, W. Iwanek, J. Pfeiffer, G. Fischer, K. Albert and V. Schurig, J. Chromatogr. A, 2005, 1095, 40 CrossRef CAS PubMed;
(d) H. M. Tan, S. F. Soh, J. Zhao, L. Yong and Y. H. Gong, Chirality, 2011, 23, 91 CrossRef PubMed;
(e) H. B. Zhang, R. J. Dai, Y. Ling, Y. X. Wen, S. Zhang, R. N. Fu and J. L. Gu, J. Chromatogr. A, 1997, 787, 161 CrossRef CAS;
(f) J. Pfeiffer and V. Schurig, J. Chromatogr. A, 1999, 840, 145 CrossRef CAS.
- W. Iwanek and A. Wzorek, Mini-Rev. Org. Chem., 2009, 6, 398 CrossRef CAS.
-
(a) W. Iwanek and J. Mattay, Eur. J. Org. Chem., 1995, 1463 CAS;
(b) R. Arnecke, V. Boehmer, S. Friebe, S. Gebauer, G. Krauss, I. Thondorf and W. Vogt, Tetrahedron Lett., 1995, 35, 6221 CrossRef;
(c) M. Y. ElGihani, H. Heaney and A. M. Slawin, Tetrahedron Lett., 1995, 36, 4905 CrossRef CAS.
-
(a) A. Wzorek, J. Mattay and W. Iwanek, Tetrahedron: Asymmetry, 2012, 23, 271 CrossRef CAS;
(b) W. Iwanek, R. Fröhlich, P. Schwab and C. Schurig, Chem. Commun., 2002, 2516 RSC;
(c) A. Wzorek, J. Mattay and W. Iwanek, Tetrahedron: Asymmetry, 2007, 18, 815 CrossRef CAS;
(d) W. Iwanek, R. Fröhlich and V. Schurig, J. Inclusion Phenom. Macrocyclic Chem., 2004, 49, 75 CrossRef CAS;
(e) W. Iwanek, R. Fröhlich and A. Wzorek, Inorg. Chem. Commun., 2005, 8, 603 CrossRef CAS;
(f) W. Iwanek, M. Urbaniak, B. Gawdzik and V. Schurig, Tetrahedron: Asymmetry, 2003, 14, 2787 CrossRef CAS.
- M. Grajda, M. Wierzbicki, P. Cmoch and A. Szumna, J. Org. Chem., 2013, 78, 11597 CrossRef CAS PubMed.
- C. Agena, Ch. Wolff and J. Mattay, Eur. J. Org. Chem., 2001, 2977 CrossRef CAS.
-
(a) S. Wiegmann, G. Fukuhara, B. Neumann, H. G. Stammler, Y. Inoue and J. Mattay, Eur. J. Org. Chem., 2013, 1240 CrossRef CAS;
(b) C. Fraschetti, M. C. Letzel, M. Paletta, J. Mattay, M. Speranza, A. Filippi, M. Aschi and A. Rozhenko, J. Mass Spectrom., 2012, 47, 72 CrossRef CAS PubMed;
(c) S. Wiegmann, B. Neumann, H. G. Stammler and J. Mattay, Eur. J. Org. Chem., 2012, 3955 CrossRef CAS;
(d) S. Wiegmann and J. Mattay, Org. Lett., 2011, 13, 3226 CrossRef CAS PubMed;
(e) J. Y. Boxhall, P. C. B. Page, M. R. J. Elsegood, Y. Chan, H. Heaney, K. E. Holmes and M. J. McGrath, Synlett, 2003, 1002 CAS;
(f) M. J. McIldowie, M. Mocerino, B. W. Skelton and A. H. White, Org. Lett., 2000, 2, 3869 CrossRef CAS PubMed;
(g) M. Klaes, C. Agena, M. Köhler, M. Inoue, T. Wada, Y. Inoue and J. Mattay, Eur. J. Org. Chem., 2003, 1404 CrossRef CAS.
-
(a) N. J. Willis and Ch. D. Bray, Chem.–Eur. J., 2012, 18, 9160 CrossRef CAS PubMed;
(b) N. Basari, K. Mlinaric-Majerski and M. Kralj, Curr. Org. Chem., 2014, 18, 3 CrossRef.
-
(a)
Quinone Methides, ed. Steven E. Rokita, John Wiley & Sons, New Jersey, 2009 Search PubMed;
(b) R. W. van de Water and T. R. R. Pettus, Tetrahedron, 2002, 58, 5367 CrossRef CAS.
- W. J. Bai, J. G. David, Z. G. Feng, M. G. Weaver, K. L. Wu and T. R. R. Pettus, Acc. Chem. Res., 2014, 47, 3655 CrossRef CAS PubMed.
-
J. J. P. Stewart, Computational Chemistry, MOPAC2012, Colorado Springs, CO, USA Search PubMed.
- F. Neese, Wiley Interdiscip. Rev.: Comput. Mol. Sci., 2012, 2, 73 CrossRef CAS.
Footnote |
† Electronic supplementary information (ESI) available: Experimental part, analytical data of 4, crystal data for 4; semiempirical calculation of the heat of formation of diastereoisomers of 4. CCDC 1405479. For ESI and crystallographic data in CIF or other electronic format see DOI: 10.1039/c5ra26106f |
|
This journal is © The Royal Society of Chemistry 2016 |
Click here to see how this site uses Cookies. View our privacy policy here.