DOI:
10.1039/C5RA24384J
(Paper)
RSC Adv., 2016,
6, 16691-16696
Alginate-assisted enrichment and purification of mesenchymal stem cells†
Received
18th November 2015
, Accepted 4th February 2016
First published on 4th February 2016
Abstract
The umbilical cord contains different populations of stem/progenitor cells such as mesenchymal, hematopoietic, trophoblastic and endothelial cells. However, the isolation methods currently in use are different, and the resulted cells are liable to cross contamination. Thus it is increasingly difficult to compare and contrast the study outcomes, which hinders progress in the field of stem cell applications. In an attempt to address this issue, we have used a magnetism assisted self-assembly method for cell capturing and releasing in combination with antibodies to mesenchymal stem cell antigens, CD105, CD73 and CD90, to identify and localize stem cells within the placenta umbilical cord. This approach is capable of detecting a minimum of 10
000 mesenchymal stem cells, and has also shown excellent specificity to distinguish other types of cells.
Introduction
The umbilical cord represents the link between mother and fetus during pregnancy. This cord is usually discarded as biological waste after birth, but its importance as a “store house” of stem cells has been explored recently.1–7 Identification and discrimination of stem cells is important in two aspects: appropriate stem cell sources for tissue engineering and direct differentiation of stem cells to somatic cells. Therefore, an efficient approach for the isolation of stem cells is critical to scientific research and clinical study.
Identification of umbilical cord-derived mesenchymal stem cells (UC-MSCs) has relied heavily on the use of explant culture method, but this approach is time-consuming and cumbersome to perform.8–11 Ten to fourteen days are needed before stem cells migrate out from tissue pieces, which precluded the clinical application of the cells. To exploit MSCs as a new thesis for tissue engineering and guide implementation of cell therapy, several strategies have been put forward and developed to enumerate MSCs, such as specific marker for the prospective isolation of MSC and fibrin microbead-based preferential adherence method.12–14 Most of the methods for stem cell identification, except the flow cytometric analysis and immunofluorescence staining method, are based on the analysis of cell lysate, such as reverse transcription-polymerase chain reaction, Western blot, and Northern blot.15,16 They use specifically expressed proteins and messenger RNAs in stem cells as markers. These methods are time-consuming and tedious to perform, and therefore, there is an urgent need to develop a new MSC assay that can capture MSCs with high efficiency, and also release MSCs without disrupting its viability and biological functions.
Recently, various affinity nanosubstrates or microfluidic chips modified by thermoresponsive polymers or aptamers have been constructed for capturing and releasing cells, and several groups have reported cancer cell sorting using these methods.17–20 However, no assay for capturing and releasing MSCs has been reported. Therefore, the development of simple, sensitive and efficient methods for capturing and releasing of MSCs would provide alternative isolating and sorting methods for clinical applications of stem cells.
The aim of the present study is to construct a new method to capture and release mesenchymal stem cell marker-positive cells, and to develop a simple and effective protocol to isolate UC-MSCs. To make this isolation protocol more efficient, the digestion reagent, digestion time, cell yield, cell growth, and culture medium have been optimized. The sorted cells showed typical MSCs morphology when observed with microscope and were characterized using fluorochrome-labeled surface marker antibodies. Additionally, these cells were cultured for up to 20 passages without differentiation. These data suggest that this method shows great promise and provides a new choice for simple, efficient and specific isolation and sorting of MSCs.
Materials and methods
All experiments were performed in compliance with the relevant laws and institutional guidelines and Guangzhou Institutes of Biomedicine and Health Ethical Committee have approved the experiments. All volunteers who donated umbilical cord samples have provided their written informed consent and the Ethics Commit-tees have approved this consent procedure.
Materials
Streptavidin (SA), alginate sodium, 4,6-diamidino-2-phenylindole (DAPI), were purchased from Sigma-Aldrich. EDC [1-ethyl-3- (3-dimethylaminopropyl) carbodiimide hydrochloride], sulfo-NHS (N-hydroxysuccinimide), Succinimidyl-6-(biotinamido) hexanoate (EZ-Link-NHS-LC-Biotin) was obtained from Thermo Fisher Scientific. All medium used for cell culture were obtained from Gibco Corporation. Mouse anti-human anti-CD73 monoclonal antibody (mAb), rabbit anti-human anti-CD90, anti-CD105 monoclonal antibodies, Alexa Fluor 488-conjugated goat anti-rabbit and Alexa Fluor 647 conjugated goat anti-mouse secondary antibodies were purchased from Abcam. Cell counting kit (CCK-8) was obtained from Beyotime Biotechnology. Chemicals for synthesis magnetic beads were obtained from Aladdin Industrial Corporation.
Methods
Preparation of Ca2+-assisted self-assembly of magnetic alginate beads (MABs)
First, we prepared three different sizes of magnetic beads (0.8–1.0 μm, 1.0–1.2 μm, 1.2–1.4 μm) according to previously reported methods.21,22 The magnetic alginate beads were formed by dropping a 50 mL mixture of 1% alginate solution and magnetite (Fe3O4) through a syringe into a 100 mL 0.1 M gently agitated calcium chloride brine (CaCl2) that is a divalent crosslinking solution. The MABs obtained were incubated for 30 min at room temperature with the same concentration of CaCl2 solution that was used for the bead formation. The MABs were collected through magnetic enrichment and stored in a 6 mM CaCl2 solution at 4 °C.
Synthesis of streptavidin-alginate (SA-Alg) conjugate and fabrication of SA labeled MABs (SA-MABs)
To prepare SA-Alg conjugate, 10 mg of alginate sodium dissolved in 1 mL of ultrapure water and incubate with EDC/NHS (4.8 mg/13.2 mg) for 30 min at room temperature to activate the carboxylic acid groups of the alginate. Then 100 μL of SA (2 mg mL−1) was added to react with activated alginate for another 4 h at 4 °C. The resulted solution was purified through dialysis and stored in 4 °C after adjusting to a concentration of 10 mg mL−1.
To fabricate SA-MABs, MABs were incubated 1
:
2 (v/v) with 10 mg mL−1 SA-Alg conjugate for 30 min. SA-Alg self-assembled on the MABs with the help of Ca2+ around the MABs. The excess SA-Alg was removed by centrifugation to obtain SA-MABs.
Biotin-assisted stem cell marker immobilization on SA-MABs
Biotin conjugated antibodies were prepared using EZ-Link-NHS-LC-Biotin according to the manufacturer's instruction. Briefly, EZ-Link-NHS-LC-Biotin was dissolved in an organic solvent such as DMSO or DMF immediately before use, with a concentration of 10 mM. Anti-CD105, anti-CD90, anti-CD73 antibodies were diluted into 2 mg mL−1 with PBS buffer separately. 27 μL of 10 mM EZ-Link-NHS-LC-Biotin solution was added into the antibody solution and incubated for 30 min at room temperature or 2 hours on ice. The excess non-reacted EZ-Link-NHS-LC-Biotin and reaction byproducts were removed by size exclusion using either desalting columns or dialysis. The purified biotin labeled anti-CD105, anti-CD90, anti-CD73 antibodies were stored at 4 °C until use.
When incubated with the biotin labeled antibodies, cells with MSC cell surface antigens (CD105, CD90, CD73) will form cell-antibody complexes with the antibody. After incubation with SA-MABs, cell-antibody complexes were immobilized on SA-MABs through the affinity between biotin and streptavidin. We defined the proses as biotin-assisted stem cell marker immobilization on SA-MABs.
Optimization and performance of the method
About 2 × 106 mesenchymal stem cells were harvested from a flask by treatment with trypsin/EDTA, and collected by centrifugation at 200G for 4 min at room temperature. Subsequently, the cells were washed with different solutions including PBS, TBS and SSC and adjust the cell solution at a concentration of 2 × 105 cell per mL. Cells bathed in different buffer solutions were incubated with biotin-labeled anti-CD105/anti-CD73/anti-CD90 antibody for 30 min at room temperature and followed by addition of SA-MABs of different diameters. Cells bound by biotin-labeled antibodies were then separated through magnetic enrichment.
Cell recovery
The UC-MSCs captured on magnetic beads using SA-MABs and biotin-labeled antibodies, were released by incubating for 5 minutes with chelate agent EDTA (20 mM). The beads were collected on a magnetic rack and the supernatant containing MSCs was transferred into a new Eppendorf tube. The released cells were counted and reseeded on a conventional 96-well culture plate at a density of 10
000 cells per well. The viability of the isolated cells was tested with the observation of the cell morphology and cell doubling time.
Viability of released mesenchymal stem cells after culture
Cell viability assay was performed using CCK-8 kit on released mesenchymal stem cells. Cells in 96-well culture plate were washed with complete cell culture medium three times and supplemented with 100 mL of 10% CCK-8 agent (v/v) in serum-free DMEM. The plates were incubated in a 37 °C/5% CO2 incubator for 2 hours. The absorbance at 450 nm was measured with a microplate reader. The experiment was performed five times, based on which the SD was calculated.
Growth and population doubling number (PDN) of sorted mesenchymal stem cells
Light microscopy visualization showed that the outgrowth cells from human umbilical cord explants and alginate assisted magnetic enrichment both displayed a spindle-shape and fibroblast-like morphology. After several passages, cell morphology showed no differences to that of the first passage. Population doubling number (PDN) was calculated according to the formulae PDN = log
N/N0 × 3.31 where N is the cell number at the end of the cultivation period, N0 is the cell number at culture initiation. Population doubling time (PDT) is the time by which cell population doubles in number. Population doubling time calculated according to the equation PDT = culture time (CT)/PDN. Cells were plated 104 cells per cm2 in 25 cm2 culture flasks and cultured until reaching confluence. Subsequently, cells were trypsinized and counted for calculating PDN.
Immunostaining. Adhered cells were trypsinized for 7–10 min with 0.25% trypsin/EDTA, washed and resuspended in 24-well plates (5 × 104 cells per well). Cells were incubated at 37 °C, 7% CO2 for 24 h. Medium was discarded and cells were washed gently with 0.05% Tween 20 diluted in PBS. Cells were fixed with fresh paraformaldehyde (PFA, 4%) for 20 min at RT. For staining of intracellular components, cells were permeabilized with 0.5% Triton 100× added for 7 min. For blocking unspecific binding, cells were rinsed with 5% BSA in PBS for 60 min at RT on a slowly plane-rotated plate. Then, cells were washed by 0.05% Tween 20 diluted in PBS and were incubated with the following primary antibodies: mouse anti-human CD73 (1
:
10), rabbit anti-human CD90 (1
:
10), rabbit anti-human CD105 (1
:
10) diluted to required concentrations with FACS buffer containing 1% BSA. Cells were washed gently in PBS with 0.05% Tween 20. For detection, cells were incubated with the following secondary antibodies: Alexa Fluor 488-conjugated goat anti-rabbit (1
:
100) or Alexa Fluor 647 conjugated goat anti-mouse (1
:
100), diluted in 1% BSA buffer, on a slowly plane-rotated plate for 45 min in darkness at RT. Cells were mounted with mounting solution and viewed by fluorescence and light by Nomarsky's optics microscopy.
Real-time reverse transcriptase-polymerase chain reaction
We performed analysis of cytokines by using real-time PCR. The cytokines examined included stem cell factor (SCF), leukemia inhibitor factor (LIF), interleukin-3 (IL-3), interleukin-6 (IL-6) and vascular endothelial growth factor (VEGF). Total RNA was extracted from 1 × 106 cells using Qiagen Rneasy Mini Kit according to the manufacturer's instruction. Real-time RT-PCR was done using Toyobo Real-time PCR Master Mix according to the manufacturer's instruction. β-Actin was used as a control and the primers used are listed in Table 1.
Table 1 Primers for real-time RT-PCR
|
Primer sequence (5′–3′) |
Size |
SCF |
F: 5′-CTCCTATTTAATCCTCTCGTC-3′ |
177bp |
R: 5′-TACTACCATCTCGCTTATCCA-3 |
|
LIF |
F: 5′-AACAACCTCATGAACCAGATCAGGAGC-3′ |
405bp |
R: 5′-ATCCTTACCCGAGGTGCAGGGCCGTAGC-3′ |
|
IL-3 |
F: 5′-CAAGCTCCCATGACCCAGAC-3′ |
400bp |
R: 5′-AGATCGCGAGGCTCAAAG-3′ |
|
IL-6 |
F: 5′-GTAGCCGCCCCACACAGACAGCC-3′ |
173bp |
R: 5′-GCCATCTTTGGAAGGTTCAGG-3′ |
|
VEGF |
F: 5′-TCGGGCCTCCGAAACCATGA-3′ |
516bp |
R: 5′-CCTGGTGAGAGATCTGGTTC-3′ |
|
β-Actin |
F: 5′-TCTGGCCTTGAGGCTATCCAGCGT-3′ |
267bp |
R: 5′-GTGGTTCACACGGCAGGCATACTC-3′′ |
|
Statistical analysis. Data were expressed as mean ± standard error of the mean. Statistical comparisons were performed using Student's t-test. p values < 0.05 were considered statistically significant.
Results
Principle of the stem cell isolation method
In this method, mesenchymal stem cell surface antigens CD90, CD105, and CD73 were used as specific markers to identify mesenchymal stem cells. As biotin is a small molecule (244 Da) and it can be conjugated to antibodies without altering the antibody activities, we obtained functional biotin-labeled anti-CD90, anti-CD105 and anti-CD73 antibodies. Alginate was assembled onto the surface of magnetic beads to form a layer of alginate around the magnetic beads by using Ca2+ as an activator. With the same method, SA-Alg conjugate was further anchored onto the surface of MABs to form another layer of alginate labeled with streptavidin. The constructed SA-MABs can be easily destroyed by treatment of EDTA that is used to “sequester” Ca2+ acting as a divalent crosslinking reagent. Once cells were bound by specific biotin-labeled antibody, in the presence of SA-MABs, the cells can be sorted using magnetic enrichment, as there is a high affinity of biotin with streptavidin. By treating with EDTA, alginate shell on Alg-MB was disintegrated, allowing the detachment of the magnetic beads from the surface of marker-positive cells. Namely, the capture and release of mesenchymal stem cell marker-positive cells were successfully achieved by using antibody-biotin-SA-MABs. Three different antibodies were used one at a time and repeat the same process with the other two different antibodies to obtain CD90+/CD105+/CD73+ cells (Fig. 1).
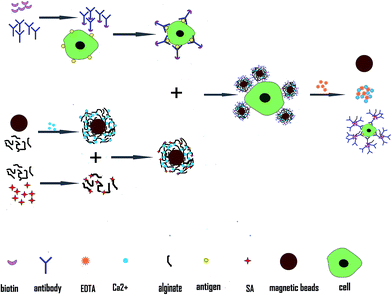 |
| Fig. 1 Scheme illustration of the magnetism assisted self-assembly method for enrichment and purification of mesenchymal stem cells from the umbilical cord. | |
Optimization of experimental conditions
To optimize the experimental conditions, buffer solutions of different component and magnetic beads of different size were tested to get the best sorting results. SA-MABs with different diameters were used to incubate with biotin-labeled antibody conjugated cells. Considering current commercial immunomagnetic beads used in cell sorting mostly are small size to avoid mechanical damage, we firstly used the magnetic beads with a diameter of 0.8–1.0 μm in the performance of alginate assisted cell sorting. Buffer solutions including PBS, TBS and SSC were used as washing and resuspending buffer. After separating the marker-positive cells through magnetic enrichment and releasing magnetic beads from targeted cells by EDTA treatment, the captured and released cells were counted and reseeded on the 96-well culture plate. After four days' culture of the sorted cells, we observed the cell morphology and counted the cell colonies of the reseeded cells. It showed that cells washed and resuspended with TBS had the most colonies (Fig. 2A). So TBS was used as buffer solution in the following experiments. To get the best cell sorting efficiency, two more kinds of magnetic beads with different diameters were tested. The results showed that the magnetic beads with a diameter of 0.8–1.0 μm got the lowest percentage of marker-positive cells, while the 1.2–1.4 μm beads do not have higher efficiency than that of 1.0–1.2 μm beads (Fig. 2B). It's clear that size of magnetic beads and component of the buffer solution significantly affect cell sorting efficiency of the antibody-biotin-SA-MABs assay, and the 1.0–1.2 μm magnetic beads with TBS buffer yield the best results.
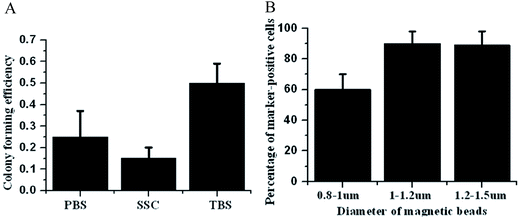 |
| Fig. 2 Optimization and performance of the method. (A) Effect of different sample buffer solutions on the assay. (B) Effect of the diameter of magnetic beads on the capture and release of UC-MSCs. Amount of stem cells: 4 × 104; volume of buffer solution: 500 μL (n = 3). | |
Performance of the method
After enzyme digestion of umbilical cord tissue, cells were isolated and released using current method (antibody-biotin-SA-MABs assay). The obtained cells were seeded at a density of 1.0 × 106 cm−2 on 6 well plates. Adherent cells showed a spindle-shape after 24 hours' culture. The cells formed a monolayer of homogenous bipolar fibroblastic-like cells with a whirlpool-like array after confluence.
Mesenchymal stem cell-like cells were successfully isolated from human full term umbilical cord and the resulted MSC-like cells were characterized by analyzing their morphology (Fig. 3A and B), immunophenotype (Fig. 4) and growth kinetics (Fig. 3D). Compared with previously reported methods, Fig. 2B showed that the isolation efficiency (90%) of the current method was significantly higher than previously reported methods.9,10 The total number of positive cells harvested from UC is 1.5–2 × 106 cells per cm using the current method, which was much higher than that of previously reported method (2.5–25 × 104 cells per cm).10,11,17,18
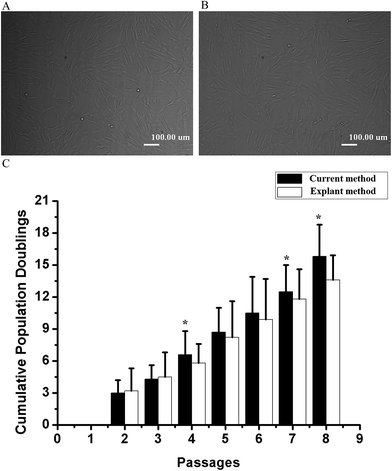 |
| Fig. 3 The morphologic characteristics, colony-forming unit-fibroblast and growth kinetics assay of resulted MSCs isolated by reported method and traditional explant culture method. (A and B) After culturing for 3 days, resulted MSCs isolated by both methods displayed a fibroblastic morphology similar to that of the adherent cells. Scale bars: 100 μm. (C) Cumulative population doubling times of the sorted MSCs were calculated to compare the two methods. The mean doubling time of cells isolated by explant culture method was shorter than that of the current method during P1 to P8. The p values were analyzed by a t-test. *p < 0.05. | |
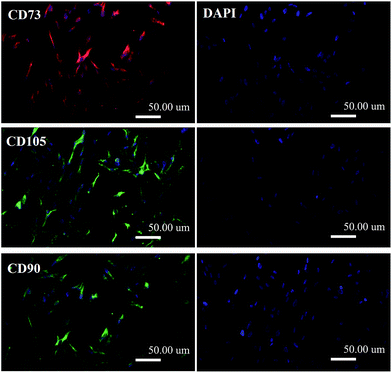 |
| Fig. 4 Surface marker profiles of resulted MSCs after culturing. The sorted cells expressed all the mesenchymal markers CD90, CD105, CD73. | |
To characterize the isolated cells, we did immunofluorescence assay with fluorochrome-labeled antibodies to surface markers (Fig. 4). We cultured the cells for 8 passages, and the cells proliferated normally and kept the mesenchymal stem cell characters as well. Interestingly, Fig. 3C showed that the proliferation analysis revealed that UC-MSCs isolated by the current method had more population doublings, which means they grew at a higher population doubling level and had a faster population doubling time. Such proliferation characteristic did not change even after 20 passages.
To measure the expression spectrum of cytokines in the isolated cells, real-time RT-PCR was performed in cells isolated by explant culture method and the current method (n = 3, P3 to P5). Fig. 5 shows that the cells isolated using both explant culture method and the current method expressed the mRNA of SCF, LIF, IL-6 and VEGF, but not that of IL-3. The expression patterns of cells isolated with the two methods have no difference.
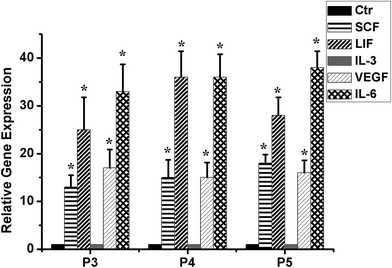 |
| Fig. 5 Gene expressions of the markers of mesenchymal stem cell including stem cell factor (SCF), leukemia inhibitor factor (LIF), interleukin-3 (IL-3), interleukin-6 (IL-6) and vascular endothelial growth factor (VEGF) after culture from passage 3 to 5 relative to control medium (normalized to 1). The differences in gene expressions between reported method and explant culture method were not statistically significant (t-test, p < 0.05). The data are mean and standard deviation values. | |
Discussion
In this study, biotin-avidin system (SAS) and Ca2+ assisted self-assembly of alginate magnetic nanoparticles are combined to capture and release mesenchymal stem cells. Biotinylated antibodies are used to recognize targeted cells, and streptavidin modified magnetic beads are used to capture the antibody bound target cells. The target cells are then isolated through magnetic enrichment. When EDTA is introduced, the SA-alginate-MB structures are destroyed and then the magnetic beads are freed from the cells.
Compared with traditional MACS, this method doesn't cause mechanical damage of the target cells, as the magnetic beads don't directly interact with the cells. In addition, the magnetic beads are released and therefore don't cause cytotoxicity or other influence to the sorted cells.23–25
This method is more efficient than traditional explant culture method when applied in the isolation of mesenchymal stem cells from umbilical cord. With this method, 1.5–2 × 106 cells per cm were harvested within 7 days, which is much higher than previously reported (2.5–25 × 104 cells per cm within 14 days) using explant culture method.10,11,17,18
Conclusion
We successfully developed a magnetism assisted self-assembly method for cell capture and release technology. In this method, Ca2+-assisted self-assembly of magnetic alginate beads (MABs) and streptavidin were used to construct SA-MABs. Anti-CD90, anti-CD105, anti-CD73 antibodies were modified by EZ-Link-NHS-LC-Biotin. SA-MABs captured marker-positive cells bound by biotin-labeled antibodies, and followed by targeted cell separation through magnetic enrichment. EDTA helps the detachment of magnetic beads from the sorted cells. The sorted cells grew well in later culture even after 20 passages. Upon optimization, we found that TBS as the buffer solution and magnetic beads of 0.8–1.0 μm were the optimum conditions for best cell sorting results. Their proliferation potential and colony-forming ability were evaluated in vitro, mesenchymal stem-cell-marker expression was verified using immunostaining and the expression spectrum of cytokines were measured by real-time RT-PCR. The cell-proliferation potential, colony-forming ability, expression level of mesenchymal stem cell marker and cytokines were higher in the current method than that in explant culture method. Compared with conventional cell sorting methods, the reported method is convenient, time-saving and the sorted cells showed better cell yield, cell growth as well as cell viability.
Acknowledgements
This work was financially supported by the Ministry of Science and Technology 973 Project (2013CB967100).
References
- H. S. Wang, S. C. Hung, S. T. Peng, C. C. Huang, H. M. Wei, Y. J. Guo, Y. S. Fu, M. C. Lai and C. C. Chen, Stem Cells, 2004, 22, 1330–1337 CrossRef PubMed.
- M. Secco, E. Zucconi, N. M. Vieira, L. L. Fogaca, A. Cerqueira, M. D. Carvalho, T. Jazedje, O. K. Okamoto, A. R. Muotri and M. Zatz, Stem Cells, 2008, 26, 146–150 CrossRef CAS PubMed.
- Y. Romanov, V. Svintsitskaya and V. Smirnov, Stem Cells, 2003, 21, 105–110 CrossRef PubMed.
- K. Mitchell, M. Weiss, B. Mitchell, P. Martin, D. Davis, L. Morales, B. Helwig, M. Beerenstrauch, K. Abou-Easa, T. Hildreth, D. Troyer and S. Medicetty, Stem Cells, 2003, 21, 50–60 CrossRef CAS PubMed.
- S. Karahuseyinoglu, O. Cinar, E. Kilic, F. Kara, G. G. Akay, D. O. Demiralp, A. Tukun, D. Uckan and A. Can, Stem Cells, 2007, 25, 319–331 CrossRef CAS PubMed.
- K. Chao, K. Chao, Y. Fu and S. Liu, PloS One, 2008, 3, e1451 Search PubMed.
- S. S. Kadam, S. Tiwari and R. R. Bhonde, In Vitro Cell. Dev. Biol.: Anim., 2009, 45, 23–27 CrossRef PubMed.
- M. N. Cécile De Bruyn, G. Raicevic, N. Meuleman, K. Pieters, B. Stamatopoulos, A. Delforge, D. Bron and L. Lagneaux, Stem Cells Dev., 2011, 20, 547–557 CrossRef PubMed.
- I. Majore, P. Moretti, F. Stahl, R. Hass and C. Kasper, Stem Cell Rev., 2011, 7, 17–31 CrossRef PubMed.
- Y. F. Han, R. Tao, T. J. Sun, J. K. Chai, G. Xu and J. Liu, Cytotechnology, 2013, 65, 819–827 CrossRef CAS PubMed.
- F. Hendijani, H. Sadeghi-Aliabadi and S. Haghjooy Javanmard, Cell Tissue Banking, 2014, 15, 555–565 CrossRef CAS PubMed.
- I. Kassis, L. Zangi, R. Rivkin, L. Levdansky, S. Samuel, G. Marx and R. Gorodetsky, Bone Marrow Transplant., 2006, 37, 967–976 CrossRef CAS PubMed.
- R. Rivkin, A. Ben-Ari, I. Kassis, L. Zangi, E. Gaberman, L. Levdansky, G. Marx and R. Gorodetsky, Cloning Stem Cells, 2007, 9, 157–175 CrossRef CAS PubMed.
- V. L. Battula, S. Treml, H. Abele and H. J. Buhring, Differentiation, 2008, 76, 326–336 CrossRef CAS PubMed.
- P. A. Zuk, M. Zhu, P. Ashjian, D. A. De Ugarte, J. I. Huang, H. Mizuno, Z. C. Alfonso, J. K. Fraser, P. Benhaim and M. H. Hedrick, Mol. Biol. Cell, 2002, 13, 4279–4295 CrossRef CAS PubMed.
- B.-M. Seo, M. Miura, S. Gronthos, P. Mark Bartold, S. Batouli, J. Brahim, M. Young, P. Gehron Robey, C. Y. Wang and S. Shi, Lancet, 2004, 364, 149–155 CrossRef CAS.
- S. S. Sungyoung Choi, C. Choi and J. Park, Anal. Chem., 2009, 81, 1964–1968 CrossRef PubMed.
- D. R. Gossett, W. M. Weaver, A. J. Mach, S. C. Hur, H. T. Tse, W. Lee, H. Amini and D. Di Carlo, Anal. Bioanal. Chem., 2010, 397, 3249–3267 CrossRef CAS PubMed.
- B. N. Blackstone, J. J. Willard, C. H. Lee, M. T. Nelson, R. T. Hart, J. J. Lannutti and H. M. Powell, Integr. Biol., 2012, 4, 1112–1121 RSC.
- Y.-Q. Li, B. K. Chandran, C. T. Lim and X. Chen, Adv. Sci., 2015 DOI:10.1002/advs.201500118.
- Z. Jiang, J. Llandro, T. Mitrelias and J. A. C. Bland, J. Appl. Phys., 2006, 99, 08S105 Search PubMed.
- F. Q. Wang, P. Li, J. P. Zhang, A. Q. Wang and Q. Wei, Pharm. Dev. Technol., 2011, 16, 228–236 CrossRef CAS PubMed.
- L. L. Wang, K. Zhang, C. Y. Xiong and Z. G. Ge, Mol. Cell. Toxicol., 2012, 8, 217–227 CrossRef CAS.
- M. Mahmoudi, A. Simchi, A. S. Milani and P. Stroeve, J. Colloid Interface Sci., 2009, 336, 510–518 CrossRef CAS PubMed.
- C. C. Berry, S. Wells, S. Charles and A. S. G. Curtis, Biomaterials, 2003, 24, 4551–4557 CrossRef CAS PubMed.
Footnote |
† Electronic supplementary information (ESI) available. See DOI: 10.1039/c5ra24384j |
|
This journal is © The Royal Society of Chemistry 2016 |