DOI:
10.1039/C6QO00187D
(Research Article)
Org. Chem. Front., 2016,
3, 1131-1136
An easy access to carboxylic acids via Pd-catalyzed hydrocarboxylation of olefins with HCOOLi as a CO surrogate under mild conditions†
Received
7th May 2016
, Accepted 16th July 2016
First published on 18th July 2016
Abstract
This paper describes an easy access to carboxylic acids via Pd-catalyzed hydrocarboxylation of olefins with HCOOLi and Ac2O under mild conditions without using external CO gas.
Introduction
Carboxylic acids are a class of highly valuable organic compounds and are present in various bioactive molecules, such as ibuprofen, naproxen, and many other non-steroidal anti-inflammatory drugs.1 Hydrocarboxylation of olefins provides a direct route to this class of compounds.2 Traditional processes are often carried out with CO under high pressure at high temperature (Scheme 1),3 which may be inconvenient for their study and application in laboratories. To circumvent the handling of toxic CO gas, various surrogates have been explored for hydrocarboxylation and related processes.4–6 As a part of our own studies, we recently reported that olefins can be effectively hydrocarboxylated to give the corresponding carboxylic acids using HCOOPh/HCOOH7–9 or HCOOH/Ac2O10,11 in the presence of a Pd catalyst (Scheme 2). However, the strong acidity of HCOOH could impair substrate compatibility and cause olefin isomerization. To overcome this drawback, we have been exploring the feasibility of replacing HCOOH with less acidic formate anions for the hydrocarboxylation. During such studies, we have found that olefins can be effectively hydrocarboxylated with a combination of HCOOLi and Ac2O (Scheme 3). Herein, we wish to report our studies on this subject.
 |
| Scheme 1 Traditional hydrocarboxylation with CO. | |
 |
| Scheme 2 Hydrocarboxylation with HCOOH. | |
 |
| Scheme 3 Hydrocarboxylation with HCOOLi. | |
Results and discussion
Initial studies were carried out with α-methylstyrene (1a), 5 mol% Pd(OAc)2, 5 mol% Xantphos, 1.0 equiv. of Ac2O, and various formate anions in 1,2-dichloroethane (DCE) at 90 °C for 24 h (Table 1, entries 1–9). Selection of the formate anion was found to be crucial for the reaction. For example, little acid product was detected with HCOONa and HCOOK (Table 1, entries 1 & 2). However, the acid product (2a) was isolated in 90% yield when HCOOLi·H2O was used (Table 1, entry 7). In the case of HCOONa and HCOOK, trace amounts of acid or no acid were detected when the reactions were carried out in the presence of 1 equiv. of H2O (Table 1, entries 8 and 9), suggesting alkali metal salts are also important for the hydrocarboxylation. Lower yields were obtained with other Pd catalysts such as Pd(PPh3)4, Pd(dba)2, PdCl2 or Pd(TFA)2 (Table 1, entries 10–13). Further studies showed that the reaction was sensitive to the ligand used. Only trace amounts of the acid product were formed with PPh3, dppe or dppp (Table 1, entries 14–16). Moderate yields were obtained with dppb and dppf (Table 1, entries 17 & 18). Among the solvents examined, DCE gave the highest yield (90%) (Table 1, entry 7). When the reaction was carried out in DCM or CH3CN, acid 2a was isolated in 82% and 39% yield, respectively (Table 1, entries 19 & 20). Little acid product was detected with DMF and DMSO (Table 1, entries 21 & 22). Ac2O was crucial to this hydrocarboxylation process. Only 34% yield was obtained when the amount of Ac2O was reduced to 20 mol% (Table 1, entry 23), and no acid 2a was detected in the absence of Ac2O (Table 1, entry 24).
Table 1 Study of the reaction conditionsa

|
Entry |
HCOOM |
[Pd] |
Ligand |
Yieldb (%) |
The reactions were carried out with 1a (0.50 mmol), HCOOM (1.00 mmol or 0.50 mmol, HCOO− = 1.0 mmol), [Pd] (0.025 mmol), ligand (0.025 mmol or 0.050 mmol, P/Pd = 2/1), and Ac2O (0.50 mmol) in DCE (0.30 mL) at 90 °C for 24 h, unless otherwise stated.
Isolated yield.
Addition of 1.0 mmol H2O.
In DCM (0.30 mL).
In CH3CN (0.30 mL).
In DMF (0.30 mL).
In DMSO (0.30 mL).
With 20 mol% Ac2O.
Without Ac2O.
|
1 |
HCOONa |
Pd(OAc)2 |
Xantphos |
0 |
2 |
HCOOK |
Pd(OAc)2 |
Xantphos |
0 |
3 |
HCOONH4 |
Pd(OAc)2 |
Xantphos |
16 |
4 |
(HCOO)2Ca |
Pd(OAc)2 |
Xantphos |
27 |
5 |
HCOOCs |
Pd(OAc)2 |
Xantphos |
42 |
6 |
(HCOO)2Mg·2H2O |
Pd(OAc)2 |
Xantphos |
62 |
7 |
HCOOLi·H2O |
Pd(OAc)2 |
Xantphos |
90 |
8c |
HCOONa + H2O |
Pd(OAc)2 |
Xantphos |
Trace |
9c |
HCOOK + H2O |
Pd(OAc)2 |
Xantphos |
0 |
10 |
HCOOLi·H2O |
Pd(PPh3)4 |
Xantphos |
65 |
11 |
HCOOLi·H2O |
Pd(dba)2 |
Xantphos |
33 |
12 |
HCOOLi·H2O |
PdCl2 |
Xantphos |
68 |
13 |
HCOOLi·H2O |
Pd(TFA)2 |
Xantphos |
85 |
14 |
HCOOLi·H2O |
Pd(OAc)2 |
PPh3 |
Trace |
15 |
HCOOLi·H2O |
Pd(OAc)2 |
dppe |
Trace |
16 |
HCOOLi·H2O |
Pd(OAc)2 |
dppp |
Trace |
17 |
HCOOLi·H2O |
Pd(OAc)2 |
dppb |
48 |
18 |
HCOOLi·H2O |
Pd(OAc)2 |
dppf |
72 |
19d |
HCOOLi·H2O |
Pd(OAc)2 |
Xantphos |
82 |
20e |
HCOOLi·H2O |
Pd(OAc)2 |
Xantphos |
39 |
21f |
HCOOLi·H2O |
Pd(OAc)2 |
Xantphos |
0 |
22g |
HCOOLi·H2O |
Pd(OAc)2 |
Xantphos |
0 |
23h |
HCOOLi·H2O |
Pd(OAc)2 |
Xantphos |
34 |
24i |
HCOOLi·H2O |
Pd(OAc)2 |
Xantphos |
0 |
As shown in Table 2, the hydrocarboxylation with the HCOOLi/Ac2O system can be extended to a variety of olefins. α-Substituted aryl olefins were effectively hydrocarboxylated to provide the corresponding carboxylic acids in 70–90% yield (Table 2, entries 1–10). Various substituents can be introduced onto the phenyl group of the substrates. The reaction also worked well for non-conjugated terminal olefins, providing the acid products in 62–96% yield (Table 2, entries 11–19). Significant amounts of by-products were obtained with olefins like 1m, 1o, and 1r under the previous conditions with HCOOH/Ac2O, likely resulting from double bond isomerization and subsequent hydrocarboxylation. Under the current reaction conditions, the olefin isomerization was minimized, and by-product formation was suppressed for these olefins. Cycloalkenes were also effective substrates for the current process, giving the acid products in 70–89% yield (Table 2, entries 20–22). When styrene was subjected to the reaction conditions, two acid regioisomers were isolated in 95% yield (b
:
l = 1
:
2.8). A mixture of three acid regioisomers was isolated in 95% and 91% yield, respectively, when 1-hexene and 3-hexene were used as substrates. As shown in Scheme 4, this hydrocarboxylation process can be also carried out on gram scale. Carboxylic acid 2a was isolated in 86% yield.
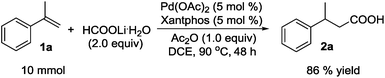 |
| Scheme 4 Gram scale hydrocarboxylation reaction. | |
Table 2 Pd-catalyzed hydrocarboxylation of olefinsa
The hydrocarboxylation reaction was also carried out in the presence of H2O18 (Scheme 5). The yield of the acid decreased and the relative content of O18 containing acid 3a (determined using GC-MS) increased with increase of the amount of H2O18 added, suggesting that H2O is involved in the reaction sequence.
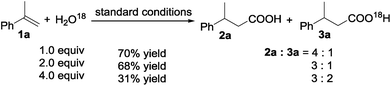 |
| Scheme 5 Isotopic experiment for the hydrocarboxylation. | |
A precise understanding of the reaction mechanism awaits further study. A couple of plausible catalytic cycles are outlined in Scheme 6. Mixed-anhydride HCOOAc (3) is likely to be generated from HCOOLi·H2O and Ac2O. The Pd(0) oxidatively adds to HCOOAc (3) to form the palladium hydride complex 4, which would hydropalladate the olefin (1a) to afford palladium complex 5. Reductive elimination of complex 5 leads to the formation of mixed-anhydride 6 and regeneration of the Pd(0) catalyst. Anhydride 6 is subsequently converted to 3-phenylbutanoic acid (2a). Alternatively, complex 4 may undergo a rearrangement to provide the palladium carbonyl complex 7. Subsequent hydropalladation of the olefin with 7 would provide complex 8, which undergoes a migratory insertion to form acylpalladium complex 9. Upon reductive elimination, complex 9 is converted to anhydride 6, with regeneration of the Pd(0) catalyst. In addition, complex 7 could also be formed from CO generated in situ via the decomposition of HCOOAc (3).12
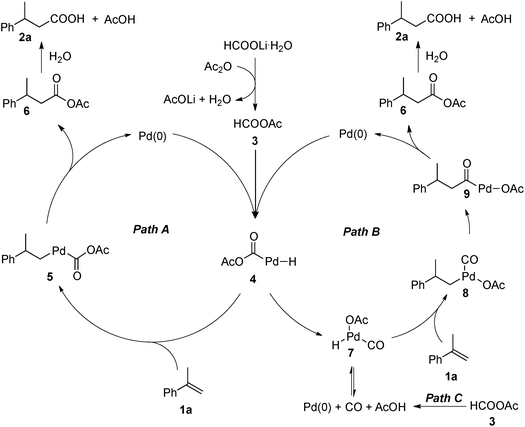 |
| Scheme 6 Proposed catalytic cycles for the hydrocarboxylation. | |
Experimental
General methods
All the commercially available reagents were used without further purification, unless otherwise noted. All solvents used for the reaction were purified with a solvent purification system. Column chromatography was performed on silica gel (200–300 mesh). 1H NMR spectra were recorded using a 400 MHz NMR spectrometer and 13C NMR spectra were recorded using a 100 MHz NMR spectrometer. IR spectra were recorded using a FT-IR spectrometer. Melting points are uncorrected.
Representative procedure for the hydrocarboxylation (Table 2, entry 1)
To a stirred mixture of Pd(OAc)2 (0.0056 g, 0.025 mmol), Xantphos (0.0145 g, 0.025 mmol), HCOOLi·H2O (0.070 g, 1.00 mmol), and DCE (0.30 mL) in a vial (1.5 mL), α-methylstyrene (1a) (0.0591 g, 0.50 mmol) and Ac2O (0.0511 g, 0.50 mmol) were added successively via a syringe. The vial was purged with Ar to remove the air and tightly sealed with a septum cap. The reaction mixture was stirred at 90 °C for 24 h, cooled to rt, diluted with CH2Cl2 (3.0 mL), and poured into 1 N aqueous NaOH (40 mL) in a separatory funnel. With vigorous shaking, the mixture was washed with CH2Cl2 (3 × 40 mL). The aqueous layer was acidified with 2 N HCl (40 mL), extracted with CH2Cl2 (3 × 30 mL), dried over Na2SO4, filtered, and concentrated to provide carboxylic acid 2a as a light yellow oil (0.0739 g, 90% yield) [for Table 2, entries 16 and 17, saturated aqueous NaHCO3 (40 mL) was used instead of 1 N aqueous NaOH (40 mL)].
Procedure for the gram scale hydrocarboxylation reaction (Scheme 4)
To a stirred mixture of Pd(OAc)2 (0.1123 g, 0.50 mmol), Xantphos (0.2893 g, 0.50 mmol), and DCE (6.0 mL) in a sealed tube (50.0 mL), α-methylstyrene (1a) (1.180 g, 10.0 mmol), HCOOLi·H2O (1.40 g, 20.0 mmol), and Ac2O (1.020 g, 10.0 mmol) were added successively via a syringe. The tube was purged with Ar to remove the air and tightly sealed. The reaction mixture was stirred at 90 °C for 48 h, cooled to rt, diluted with CH2Cl2 (6.0 mL), and poured into 1 N NaOH (80 mL) in a separatory funnel. With vigorous shaking, the mixture was washed with CH2Cl2 (3 × 100 mL). The aqueous layer was acidified with 2 N HCl (80 mL), extracted with CH2Cl2 (3 × 100 mL), dried over Na2SO4, filtered, and concentrated to provide carboxylic acid 2a as a light yellow oil (1.409 g, 86% yield).
3-Phenylbutanoic acid (2a)7a,10.
Light yellow oil. 73.4 mg (yield 90%). IR (film) 2966, 1707, 1452 cm−1. 1H NMR (400 MHz, CDCl3) δ = 7.34–7.25 (m, 2H), 7.25–7.15 (m, 3H), 3.33–3.19 (m, 1H), 2.66 (dd, J = 15.5, 6.8 Hz, 1H), 2.56 (dd, J = 15.5, 8.2 Hz, 1H), 1.31 (d, J = 7.0 Hz, 3H) ppm. 13C NMR (100 MHz, CDCl3) δ = 179.2, 145.6, 128.8, 126.9, 126.7, 42.8, 36.3, 22.0 ppm.
3-Phenylpentanoic acid (2b)7a.
Light yellow oil. 74.0 mg (yield 83%). IR (film) 2964, 1708 cm−1. 1H NMR (400 MHz, CDCl3) δ = 7.32–7.23 (m, 2H), 7.23–7.11 (m, 3H), 3.04–2.91 (m, 1H), 2.65 (dd, J = 15.6, 7.2 Hz, 1H), 2.59 (dd, J = 15.6, 7.9 Hz, 1H), 1.79–1.66 (m, 1H), 1.66–1.52 (m, 1H), 0.77 (t, J = 7.3 Hz, 3H) ppm. 13C NMR (100 MHz, CDCl3) δ = 179.3, 143.8, 128.6, 127.7, 126.7, 43.7, 41.4, 29.3, 12.0 ppm.
3-Phenylhexanoic acid (2c)13.
Light yellow oil. 67.2 mg (yield 70%). IR (film) 2958, 1708 cm−1. 1H NMR (400 MHz, CDCl3) δ = 7.31–7.23 (m, 2H), 7.23–7.12 (m, 3H), 3.14–3.00 (m, 1H), 2.64 (dd, J = 15.6, 7.2 Hz, 1H), 2.58 (dd, J = 15.0, 7.3 Hz, 1H), 1.69–1.50 (m, 2H), 1.28–1.04 (m, 2H), 0.84 (t, J = 7.3 Hz, 3H) ppm. 13C NMR (100 MHz, CDCl3) δ = 179.3, 144.0, 128.6, 127.6, 126.7, 41.8, 41.7, 38.6, 20.6, 14.1 ppm.
3-(o-Tolyl)butanoic acid (2d)7a,10.
White solid. m.p. 44–46 °C. 73.5 mg (yield 83%). IR (film) 2969, 1708 cm−1. 1H NMR (400 MHz, CDCl3) δ = 7.21–7.04 (m, 4H), 3.60–3.45 (m, 1H), 2.66 (dd, J = 15.6, 6.3 Hz, 1H), 2.55 (dd, J = 15.6, 8.6 Hz, 1H), 2.36 (s, 3H), 1.26 (d, J = 6.9 Hz, 3H) ppm. 13C NMR (100 MHz, CDCl3) δ = 179.4, 143.8, 135.5, 130.7, 126.6, 126.4, 125.1, 42.1, 31.3, 21.4, 19.6 ppm.
3-(m-Tolyl)butanoic acid (2e)7a,10.
Light yellow oil. 72.8 mg (yield 82%). IR (film) 2966, 1708 cm−1. 1H NMR (400 MHz, CDCl3) δ = 7.22–7.13 (m, 1H), 7.06–6.96 (m, 3H), 3.29–3.16 (m, 1H), 2.65 (dd, J = 15.5, 6.6 Hz, 1H), 2.55 (dd, J = 15.5, 8.4 Hz, 1H), 2.32 (s, 3H), 1.29 (d, J = 7.0 Hz, 3H) ppm. 13C NMR (100 MHz, CDCl3) δ = 179.3, 145.6, 138.3, 128.6, 127.7, 127.5, 123.9, 42.8, 36.2, 22.0, 21.6 ppm.
3-(p-Tolyl)butanoic acid (2f)7a,10.
White solid. m.p. 86–88 °C. 74.2 mg (yield 83%). IR (film) 2966, 1701 cm−1. 1H NMR (400 MHz, CDCl3) δ = 7.12 (s, 4H), 3.31–3.18 (m, 1H), 2.66 (dd, J = 15.4, 6.8 Hz, 1H), 2.56 (dd, J = 15.5, 8.2 Hz, 1H), 2.32 (s, 3H), 1.30 (d, J = 7.0 Hz, 3H) ppm. 13C NMR (100 MHz, CDCl3) δ = 178.6, 142.6, 136.2, 129.5, 126.8, 42.8, 36.0, 22.2, 21.2 ppm.
3-(4-Methoxyphenyl)butanoic acid (2g)7a.
Light yellow oil. 81.1 mg (yield 84%). IR (film) 2963, 1708 cm−1. 1H NMR (400 MHz, CDCl3) δ = 7.17 (d, J = 8.6 Hz, 2H), 6.87 (d, J = 8.7 Hz, 2H), 3.80 (s, 3H), 3.32–3.19 (m, 1H), 2.65 (dd, J = 15.4, 7.0 Hz, 1H), 2.57 (dd, J = 15.4, 8.1 Hz, 1H), 1.32 (d, J = 7.0 Hz, 3H) ppm. 13C NMR (100 MHz, CDCl3) δ = 179.1, 158.3, 137.7, 127.8, 114.1, 55.4, 43.1, 35.5, 22.2 ppm.
3-(4-Chlorophenyl)butanoic acid (2h)7a,10.
White solid. m.p. 89–90 °C. 81.4 mg (yield 82%). IR (film) 2963, 1702 cm−1. 1H NMR (400 MHz, CDCl3) δ = 7.26 (d, J = 8.4 Hz, 2H), 7.15 (d, J = 8.4 Hz, 2H), 3.30–3.18 (m, 1H), 2.62 (dd, J = 15.6, 7.2 Hz, 1H), 2.56 (dd, J = 15.6, 7.8 Hz, 1H), 1.29 (d, J = 7.0 Hz, 3H) ppm. 13C NMR (100 MHz, CDCl3) δ = 178.9, 144.0, 132.4, 128.9, 128.3, 42.7, 35.8, 22.1 ppm.
3-(4-Fluorophenyl)butanoic acid (2i)10.
White solid. m.p. 63–67 °C. 77.2 mg (yield 85%). IR (film) 2973, 1702 cm−1. 1H NMR (400 MHz, CDCl3) δ = 7.23–7.15 (m, 2H), 7.04–6.94 (m, 2H), 3.34–3.20 (m, 1H), 2.64 (dd, J = 15.6, 7.2 Hz, 1H), 2.58 (dd, J = 15.6, 7.9 Hz, 1H), 1.31 (d, J = 7.0 Hz, 3H) ppm. 13C NMR (100 MHz, CDCl3) δ = 179.1, 161.7 (d, J = 243 Hz), 141.2 (d, J = 3 Hz), 128.3 (d, J = 8 Hz), 115.5 (d, J = 21 Hz), 42.9, 35.7, 22.2 ppm.
3-(Naphthalen-2-yl)butanoic acid (2j)7a,10.
White solid. m.p. 106–108 °C. 80.6 mg (yield 75%). IR (film) 2973, 1696 cm−1. 1H NMR (400 MHz, CDCl3) δ = 7.87–7.78 (m, 3H), 7.70 (s, 1H), 7.54–7.43 (m, 2H), 7.43–7.38 (m, 1H), 3.55–3.41 (m, 1H), 2.81 (dd, J = 15.6, 6.8 Hz, 1H), 2.70 (dd, J = 15.6, 8.1 Hz, 1H), 1.43 (d, J = 6.9 Hz, 3H) ppm. 13C NMR (100 MHz, CDCl3) δ = 179.1, 143.0, 133.7, 132.5, 128.5, 127.9, 127.8, 126.2, 125.7, 125.6, 125.1, 42.7, 36.4, 22.1 ppm.
3-Methyl-4-phenylbutanoic acid (2k)7a,10.
Light yellow oil. 85.1 mg (yield 96%). IR (film) 2961, 1707 cm−1. 1H NMR (400 MHz, CDCl3) δ = 7.31–7.23 (m, 2H), 7.23–7.11 (m, 3H), 2.63 (dd, J = 13.4, 6.8 Hz, 1H), 2.51 (dd, J = 13.4, 7.4 Hz, 1H), 2.37 (dd, J = 14.8, 5.5 Hz, 1H), 2.33–2.21 (m, 1H), 2.16 (dd, J = 14.8, 7.9 Hz, 1H), 0.97 (d, J = 6.5 Hz, 3H) ppm. 13C NMR (100 MHz, CDCl3) δ = 180.1, 140.2, 129.4, 128.5, 126.3, 43.1, 41.0, 32.3, 19.8 ppm.
3,4,4-Trimethylpentanoic acid (2l)10.
Light yellow oil. 47.7 mg (yield 66%). IR (film) 2964, 1709 cm−1. 1H NMR (400 MHz, CDCl3) δ = 2.55 (dd, J = 14.9, 3.2 Hz, 1H), 1.99 (dd, J = 14.9, 10.8 Hz, 1H), 1.85–1.74 (m, 1H), 0.92 (d, J = 6.8 Hz, 3H), 0.88 (s, 9H) ppm. 13C NMR (100 MHz, CDCl3) δ = 181.4, 40.0, 37.6, 32.9, 27.3, 15.2 ppm.
3-Methylpentanoic acid (2m)14.
Light yellow oil. 36.0 mg (yield 62%). IR (film) 2964, 1709 cm−1. 1H NMR (400 MHz, CDCl3) δ = 2.36 (dd, J = 15.0, 6.0 Hz, 1H), 2.14 (dd, J = 15.0, 8.2 Hz, 1H), 1.96–1.81 (m, 1H), 1.46–1.32 (m, 1H), 1.32–1.16 (m, 1H), 0.96 (d, J = 6.7 Hz, 3H), 0.90 (t, J = 7.4 Hz, 3H) ppm. 13C NMR (100 MHz, CDCl3) δ = 180.5, 41.5, 31.9, 29.5, 19.4, 11.5 ppm. HRMS (ESI): m/z Calcd for [C6H11O2]−: 115.0765; Found: 115.0765.
3-Methylheptanoic acid (2n)15.
Light yellow oil. 49.8 mg (yield 69%). IR (film) 2959, 1709 cm−1. 1H NMR (400 MHz, CDCl3) δ = 2.35 (dd, J = 15.0, 6.0 Hz, 1H), 2.14 (dd, J = 14.9, 8.2 Hz, 1H), 2.02–1.87 (m, 1H), 1.40–1.14 (m, 6H), 0.96 (d, J = 6.6 Hz, 3H), 0.89 (t, J = 6.8 Hz, 3H) ppm. 13C NMR (100 MHz, CDCl3) δ = 180.4, 41.9, 36.6, 30.3, 29.3, 23.0, 19.9, 14.3 ppm. HRMS (ESI): m/z Calcd for [C8H15O2]−: 143.1078; Found: 143.1076.
3-Ethylpentanoic acid (2o).
Light yellow oil. 50.5 mg (yield 78%). IR (film) 2964, 1708 cm−1. 1H NMR (400 MHz, CDCl3) δ = 2.28 (d, J = 7.0 Hz, 2H), 1.82–1.68 (m, 1H), 1.47–1.24 (m, 4H), 0.88 (t, J = 7.4 Hz, 6H) ppm. 13C NMR (100 MHz, CDCl3) δ = 180.8, 38.5, 37.9, 26.0, 11.0 ppm. HRMS (ESI): m/z Calcd for [C7H13O2]−: 129.0921; Found: 129.0922.
5-Acetoxy-3 methylpentanoic acid (2p)7a.
Light yellow oil. 78.2 mg (yield 90%). IR (film) 2964, 1739, 1710 cm−1. 1H NMR (400 MHz, CDCl3) δ = 4.20–4.00 (m, 2H), 2.36 (dd, J = 15.2, 6.0 Hz, 1H), 2.20 (dd, J = 15.3, 7.8 Hz, 1H), 2.15–2.05 (m, 1H), 2.02 (s, 3H), 1.77–1.63 (m, 1H), 1.60–1.45 (m, 1H), 0.99 (d, J = 6.6 Hz, 3H) ppm. 13C NMR (100 MHz, CDCl3) δ = 179.1, 171.5, 62.6, 41.4, 35.1, 27.3, 21.1, 19.7 ppm.
5-Butoxy-3-methylpentanoic acid (2q)7a.
Light yellow oil. 68.6 mg (yield 73%). IR (film) 2960, 1709 cm−1. 1H NMR (400 MHz, CDCl3) δ = 3.51–3.35 (m, 4H), 2.39 (dd, J = 14.8, 5.7 Hz, 1H), 2.19 (dd, J = 14.8, 7.9 Hz, 1H), 2.15–2.00 (m, 1H), 1.72–1.60 (m, 1H), 1.60–1.42 (m, 3H), 1.42–1.27 (m, 2H), 0.98 (d, J = 6.6 Hz, 3H), 0.89 (t, J = 7.4 Hz, 3H) ppm. 13C NMR (100 MHz, CDCl3) δ = 179.2, 71.0, 68.9, 41.7, 36.3, 31.9, 27.8, 20.1, 19.5, 14.1 ppm.
2-Cyclohexylacetic acid (2r)16.
Light yellow oil. 63.5 mg (yield 90%). IR (film) 2925, 1707 cm−1. 1H NMR (400 MHz, CDCl3) δ = 2.21 (d, J = 6.8 Hz, 2H), 1.87–1.56 (m, 6H), 1.36–1.20 (m, 2H), 1.20–1.06 (m, 1H), 1.06–0.84 (m, 2H) ppm. 13C NMR (100 MHz, CDCl3) δ = 180.2, 42.2, 34.8, 33.2, 26.3, 26.2 ppm. HRMS (ESI): m/z Calcd for [C8H13O2]−: 141.0921; Found: 141.0921.
3-Cyclooctylpropanoic acid (2s).
Light yellow oil. 69.9 mg (yield 76%). IR (film) 2920, 1709 cm−1. 1H NMR (400 MHz, CDCl3) δ = 2.35 (t, J = 7.2 Hz, 2H), 1.73–1.35 (m, 15H), 1.35–1.19 (m, 2H) ppm. 13C NMR (100 MHz, CDCl3) δ = 181.2, 37.0, 32.9, 32.5, 32.1, 27.4, 26.4, 25.5 ppm. HRMS (ESI): m/z Calcd for [C11H19O2]−: 183.1391; Found: 183.1390.
Cyclopentanecarboxylic acid (2t)7a,10.
Light yellow oil. 39.9 mg (yield 70%). IR (film) 2961, 1704 cm−1. 1H NMR (400 MHz, CDCl3) δ = 2.82–2.69 (m, 1H), 1.99–1.77 (m, 4H), 1.77–1.65 (m, 2H), 1.65–1.52 (m, 2H) ppm. 13C NMR (100 MHz, CDCl3) δ = 183.8, 43.9, 30.2, 26.0 ppm.
Cyclohexanecarboxylic acid (2u)7a,10.
Light yellow oil. 56.8 mg (yield 89%). IR (film) 2934, 1704 cm−1. 1H NMR (400 MHz, CDCl3) δ = 2.32 (tt, J = 11.2, 3.6 Hz, 1H), 1.99–1.86 (m, 2H), 1.82–1.69 (m, 2H), 1.69–1.58 (m, 1H), 1.52–1.36 (m, 2H), 1.36–1.14 (m, 3H) ppm. 13C NMR (100 MHz, CDCl3) δ = 183.1, 43.2, 29.0, 25.9, 25.5 ppm.
Cycloheptanecarboxylic acid (2v)7a,10.
Light yellow oil. 58.4 mg (yield 82%). IR (film) 2928, 1703 cm−1. 1H NMR (400 MHz, CDCl3) δ = 2.56–2.45 (m, 1H), 2.02–1.89 (m, 2H), 1.79–1.62 (m, 4H), 1.62–1.39 (m, 6H) ppm. 13C NMR (100 MHz, CDCl3) δ = 184.0, 45.0, 30.8, 28.5, 26.4 ppm.
Conclusions
In summary, we have shown that a variety of olefins can be efficiently hydrocarboxylated with HCOOLi and Ac2O in the presence of a Pd catalyst under mild reaction conditions, providing the corresponding carboxylic acids in good yields without using external toxic CO gas. The reaction conditions are less acidic compared to the previous HCOOH/Ac2O system, which could be advantageous for certain acid-sensitive substrates. This hydrocarboxylation process is also operationally simple and can be carried out on gram scale. Further efforts will be devoted to understanding the reaction mechanism, expanding the substrate scope, and developing an asymmetric process for the reaction.
Acknowledgements
We gratefully acknowledge the National Natural Science Foundation of China (21472083) and Nanjing University for financial support.
Notes and references
- For leading reviews, see:
(a) J. P. Rieu, A. Boucherle, H. Cousse and G. Mouzin, Tetrahedron, 1986, 42, 4095 CrossRef CAS;
(b) E. Feng, D. Ye, J. Li, D. Zhang, J. Wang, F. Zhao, R. Hilgenfeld, M. Zheng, H. Jiang and H. Liu, ChemMedChem, 2012, 7, 1527 CrossRef CAS PubMed.
- For leading reviews on metal-catalyzed hydrocarboxylation, see:
(a) G. Kiss, Chem. Rev., 2001, 101, 3435 CrossRef CAS PubMed;
(b) I. Del Rio, C. Claver and P. W. N. M. Van Leeuwen, Eur. J. Inorg. Chem., 2001, 2719 CrossRef CAS;
(c)
B. E. Ali and H. Alper, in Handbook of Organopalladium Chemistry for Organic Synthesis, ed. E. Negishi, John Wiley & Sons, Inc., New York, 2002, p. 2333 Search PubMed;
(d)
B. E. Ali and H. Alper, in Transition Metals for Organic Synthesis, ed. M. Beller and C. Bolm, John Wiley & Sons, Inc., New York, 2nd edn, 2004, vol. 1, p. 113 Search PubMed;
(e) A. Brennfuhrer, H. Neumann and M. Beller, ChemCatChem, 2009, 1, 28 CrossRef.
- For leading references on metal-catalyzed hydrocarboxylation of olefins with CO gas, see:
(a) H. Alper, J. B. Woell, B. Despeyroux and D. J. H. Smith, J. Chem. Soc., Chem. Commun., 1983, 1270 RSC;
(b) T. Fuchikami, K. Ohishi and I. Ojima, J. Org. Chem., 1983, 48, 3803 CrossRef CAS;
(c) H. Alper and N. Hamel, J. Am. Chem. Soc., 1990, 112, 2803 CrossRef CAS;
(d) B. E. Ali and H. Alper, J. Mol. Catal., 1992, 77, 7 CrossRef;
(e) B. E. Ali and H. Alper, J. Org. Chem., 1993, 58, 3595 CrossRef;
(f) J. Y. Yoon, E. J. Jang, K. H. Lee and J. S. Lee, J. Mol. Catal. A: Chem., 1997, 118, 181 CrossRef CAS;
(g) D. M. Serrano, A. Aghmiz, M. Dieguez, A. M. M. Bulto, C. Claver and D. Sinou, Tetrahedron: Asymmetry, 1999, 10, 4463 CrossRef;
(h) J. Real, E. Prat, S. G. Cabello, M. Pagès and A. Polo, Organometallics, 2000, 19, 4715 CrossRef CAS;
(i) I. Del Rio, N. Ruiz, C. Claver, L. A. Veen and P. W. N. M. Van Leeuwen, J. Mol. Catal. A: Chem., 2000, 161, 39 CrossRef CAS;
(j) B. E. Ali and M. Fettouhi, J. Mol. Catal. A: Chem., 2002, 182, 195 CrossRef;
(k) A. Aghmiz, M. G. Pedros, A. M. M. Bulto and F. P. Schmidtchen, Catal. Lett., 2005, 103, 191 CrossRef CAS;
(l) B. R. Sarkar and R. V. Chaudhari, Catal. Surv. Asia, 2005, 9, 193 CrossRef CAS;
(m) C. T. Estorach, A. Orejon, N. Ruiz, A. M. M. Bulto and G. Laurenczy, Eur. J. Inorg. Chem., 2008, 3524 CrossRef CAS;
(n) C. Tang, Y. Zeng, X. Yang, Y. Lei and G. Wang, J. Mol. Catal. A: Chem., 2009, 314, 15 CrossRef CAS;
(o) T. M. Konrad, J. A. Fuentes, A. M. Z. Slawin and M. L. Clarke, Angew. Chem., Int. Ed., 2010, 122, 9383 CrossRef.
- For leading reviews on carbonylation with CO surrogates, see:
(a) G. Jenner, Appl. Catal., A, 1995, 121, 25 CrossRef CAS;
(b) T. Morimoto and K. Kakiuchi, Angew. Chem., Int. Ed., 2004, 43, 5580 CrossRef CAS PubMed;
(c) T. Morimoto, K. Fuji and K. Kakiuchi, J. Synth. Org. Chem., Jpn., 2004, 62, 861 CrossRef CAS;
(d) H. Konishi and K. Manbe, Synlett, 2014, 1971 CAS;
(e) L. Wu, Q. Liu, R. Jackstell and M. Beller, Angew. Chem., Int. Ed., 2014, 53, 6310 CrossRef CAS PubMed;
(f) B. Liu, F. Hu and B. Shi, ACS Catal., 2015, 5, 1863 CrossRef CAS.
- For leading references on Ru-catalyzed hydroesterification with formates in the absence of CO gas, see:
(a) W. Ueda, T. Yokoyama, Y. Morikawa, Y. Moro-oka and T. Ikawa, J. Mol. Catal., 1988, 44, 197 CrossRef CAS;
(b) E. M. Nahmed and G. Jenner, J. Mol. Catal., 1990, 59, L15 CrossRef CAS;
(c) G. Lavigne and N. Lugan, J. Am. Chem. Soc., 1992, 114, 10669 CrossRef CAS;
(d) G. Jenner and A. B. Taleb, J. Mol. Catal., 1994, 91, 31 CrossRef CAS;
(e) C. Legrand, Y. Castanet, A. Mortreux and F. Petit, J. Chem. Soc., Chem. Commun., 1994, 1173 RSC;
(f) S. Fabre, P. Kalck and G. Lavigne, Angew. Chem., Int. Ed., 1997, 36, 1092 CrossRef CAS;
(g) S. Ko, Y. Na and S. Chang, J. Am. Chem. Soc., 2002, 124, 750 CrossRef CAS PubMed;
(h) Y. Na, S. Ko, L. K. Hwang and S. Chang, Tetrahedron Lett., 2003, 44, 4475 CrossRef CAS;
(i) L. Wang and P. E. Floreancig, Org. Lett., 2004, 6, 4207 CrossRef CAS PubMed;
(j) E. J. Park, J. M. Lee, H. Han and S. Chang, Org. Lett., 2006, 8, 4355 CrossRef CAS PubMed;
(k) H. Konishi, T. Ueda, T. Muto and K. Manabe, Org. Lett., 2012, 14, 4722 CrossRef CAS PubMed;
(l) I. Profir, M. Beller and I. Fleischer, Org. Biomol. Chem., 2014, 12, 6972 RSC;
(m) B. Li, S. Lee, K. Shin and S. Chang, Org. Lett., 2014, 16, 2010 CrossRef CAS PubMed.
- For leading references on Pd-catalyzed hydroesterification with formates in the absence of CO gas, see:
(a) J. Grevin and P. Kalck, J. Organomet. Chem., 1994, 476, C23 CrossRef CAS;
(b) Y. Katafuchi, T. Fujihara, T. Iwai, J. Terao and Y. Tsuji, Adv. Synth. Catal., 2011, 353, 475 CrossRef CAS;
(c) I. Fleischer, R. Jennerjahn, D. Cozzula, R. Jackstell, R. Franke and M. Beller, ChemSusChem, 2013, 6, 417 CrossRef CAS PubMed.
-
(a) Y. Wang, W. Ren, J. Li, H. Wang and Y. Shi, Org. Lett., 2014, 16, 5960 CrossRef CAS PubMed;
(b) J. Dai, W. Ren, H. Wang and Y. Shi, Org. Biomol. Chem., 2015, 13, 8429 RSC.
- For our related work on regioselective hydroesterification of alkenylphenols to provide lactones with phenyl formate, see:
(a) H. Wang, B. Dong, Y. Wang, J. Li and Y. Shi, Org. Lett., 2014, 16, 186 CrossRef CAS PubMed;
(b) J. Li, W. Chang, W. Ren, W. Liu, H. Wang and Y. Shi, Org. Biomol. Chem., 2015, 13, 10341 RSC.
- For our related work on regiodivergent hydroesterification of aryl olefins with phenyl formate, see: W. Ren, W. Chang, Y. Wang, J. Li and Y. Shi, Org. Lett., 2015, 17, 3544 CrossRef CAS PubMed.
- Y. Wang, W. Ren and Y. Shi, Org. Biomol. Chem., 2015, 13, 8416 CAS.
- For a related report on hydrocarboxylation of alkynes, see: J. Hou, J. Xie and Q. Zhou, Angew. Chem., Int. Ed., 2015, 54, 6302 CrossRef CAS PubMed.
- For a report on the Pd-catalyzed hydroxycarbonylation of aryl and vinyl halides or triflates with in situ generated CO from acetic anhydride and formate anions, see: S. Cacchi, G. Fabrizi and A. Goggiamani, Org. Lett., 2003, 5, 4269 CrossRef CAS PubMed.
- M. C. Estévez, R. Galve, F. S. Baeza and M. P. Marco, Anal. Chem., 2005, 77, 5283 CrossRef PubMed.
- E. I. Troyanskii, I. V. Svitan and G. I. Nikishin, Org. Chem., 1985, 125 Search PubMed.
- B. V. Burger and W. G. B. Petersen, J. Chem. Ecol., 2002, 28, 501 CrossRef CAS PubMed.
- A. C. Bonaparte, M. P. Betush, B. M. Panseri, D. J. Mastarone, R. K. Murphy and S. S. Murphree, Org. Lett., 2011, 13, 1447 CrossRef CAS PubMed.
Footnote |
† Electronic supplementary information (ESI) available: Experimental procedures, characterization data, and NMR spectra. See DOI: 10.1039/c6qo00187d |
|
This journal is © the Partner Organisations 2016 |
Click here to see how this site uses Cookies. View our privacy policy here.