DOI:
10.1039/C6QO00163G
(Research Article)
Org. Chem. Front., 2016,
3, 865-869
Generation of (2-oxoindolin-3-yl)methanesulfonohydrazides via a photo-induced reaction of N-(2-iodoaryl)acrylamide, DABSO, and hydrazine†
Received
16th April 2016
, Accepted 12th May 2016
First published on 13th May 2016
Abstract
A photo-induced catalyst-free three-component reaction of N-(2-iodoaryl)acrylamide, sulfur dioxide, and hydrazine is reported. Under ultraviolet irradiation, diverse (2-oxoindolin-3-yl)methanesulfonohydrazides are generated in moderate to good yields. During the process, the sulfonyl group can be easily incorporated via insertion of sulfur dioxide in the absence of any metals or photo-redox catalysts under mild conditions. A plausible mechanism is proposed, which involves 5-exo radical cyclization and subsequent insertion of sulfur dioxide.
Introduction
In the past decade, photo-induced organic transformations using photocatalysis have rapidly developed.1,2 In most cases, reactive radical species as intermediates are involved during the process. The presence of photocatalysis usually assists electron transfer to promote the completion of the reaction. Recently, interesting results under catalyst-free conditions enabled by photoenergy were reported.3 Under ultraviolet irradiation, aryl/alkyl halides would afford the corresponding aryl/alkyl radicals in the absence of photocatalysis during the reaction process. For example, Li and co-workers described a metal-free coupling of aryl alkynes with alkyl iodides in water under ultraviolet irradiation.3a They also developed a photo-induced halogen exchange in aryl or vinyl halides under metal-free conditions (Scheme 1).3b It was found that these reactions proceeded through alkyl or aryl radicals, generated from the corresponding alkyl/aryl halides enabled by photoenergy. Thus, a new route to the coupling of aryl/alkyl halides would be expected, since these reactions promoted by ultraviolet irradiation could be performed under metal-free conditions. This approach is promising, especially in the drug discovery process. Consequently, it will avoid contamination of metal salts in the final products.
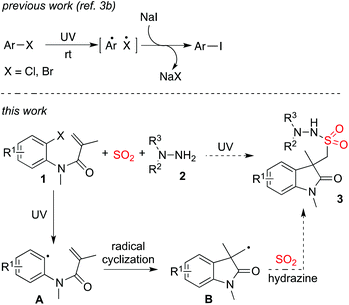 |
| Scheme 1 A proposed three-component reaction of N-(2-bromoaryl)acrylamide 1, sulfur dioxide, and hydrazine 2. | |
Recently, continuous attention has been given to the fixation of sulfur dioxide into small molecules.4–7 A sulfonyl group can be easily introduced by using sulfur dioxide as the starting material. The importance of the sulfonyl group is well recognized, due to its wide presence in pharmaceutical and agrochemical molecules.8,9 So far, transition-metal-catalyzed or metal-free aminosulfonylation of aryl electrophiles, sulfur dioxide, and hydrazines has been developed.4 For instance, Willis and co-workers described a palladium-catalyzed aminosulfonylation of aryl iodides, sulfur dioxide, and hydrazines.4a During the transformation, an innoxious and bench-stable DABCO·(SO2)2 (DABSO) was used as the SO2 source.7 In the meantime, we reported an efficient route to aryl N-aminosulfonamides through a coupling of aryldiazonium tetrafluoroborates, sulfur dioxide, and hydrazines under metal-free conditions.4j It was demonstrated that this transformation proceeded through a radical process. Additionally, the strategy via insertion of sulfur dioxide has been applied effectively in the generation of sulfones.5,6
As part of a program in our laboratory for the construction of nitrogen-containing heterocycles in the studies of chemical genetics,10 we are interested in methodology development for the synthesis of sulfonyl-substituted 2-oxoindoline derivatives. As a privileged scaffold, the 2-oxoindoline core can be found in many natural products and pharmaceuticals with remarkable biological activities.11 It would be attractive if the fixation of sulfur dioxide could be incorporated in the generation of sulfonyl-substituted 2-oxoindoline compounds. As mentioned above, aminosulfonylation of aryldiazonium tetrafluoroborates, sulfur dioxide, and hydrazines proceeded through a radical process.4j We also discovered that aryl halides could be used in the insertion of sulfur dioxide with hydrazines enabled by photoenergy.4n Prompted by these results and the advancement of metal-free coupling of aryl/alkyl halides under ultraviolet irradiation,3 we envisioned that N-(2-haloaryl)acrylamide 1 could be utilized as the starting material in the reaction of sulfur dioxide and hydrazine for the synthesis of sulfonyl-substituted 2-oxoindolines (Scheme 1). During the transformation, tandem 5-exo radical cyclization and insertion of sulfur dioxide were designed for the final outcome although a competitive pathway involving the direct aminosulfonylation of aryl halides seemed to be inevitable. We reasoned that under ultraviolet irradiation, the C–X bond of N-(2-haloaryl)acrylamide 1 would be broken to produce radical A. Consequently, an intramolecular 5-exo cyclization would happen to afford an alkyl radical B, which was believed to be the key intermediate during the tandem process. We expected that the subsequent insertion of sulfur dioxide via the radical process would provide the desired (2-oxoindolin-3-yl)methanesulfonohydrazide 3. To verify the feasibility of this hypothesis as shown in Scheme 1, we therefore started to explore the three-component reaction of N-(2-haloaryl)acrylamide 1, sulfur dioxide, and hydrazine 2.
Results and discussion
At the outset, the reaction of N-(2-iodophenyl)-N-methylmethacrylamide 1a, DABCO·(SO2)2, and morpholin-4-amine 2a was selected as the model for method development (Table 1). The reaction was performed at 25 °C under ultraviolet irradiation by using a standard photo-reactor (see the ESI†). Initially, the reaction was performed in DMF (Table 1, entry 1). However, no reaction occurred under the conditions. We postulated that the solubility of DABSO in the solvent might retard the transformation. Thus, TBAI (tetrabutylammonium iodide) was added to make DABSO more soluble, and the reaction showed a trace amount of the product (Table 1, entry 2). A similar result was observed when the solvent was changed to 1,4-dioxane (Table 1, entry 3). No reaction took place when dichloromethane or 1,2-dichloroethane was employed as the solvent (Table 1, entries 4 and 5). To our delight, the reaction worked efficiently in acetonitrile, leading to the corresponding product 3a in 71% yield (Table 1, entry 6). A controlled experiment showed that the presence of TBAI was essential in the reaction, since the corresponding product 3a could not be isolated without the addition of TBAI (Table 1, entry 7). To our surprise, the reaction failed to provide the desired product 3a when TBAI was replaced by TBAB or TBAC (Table 1, entries 8 and 9). The yield was lower when the amount of TBAI was decreased (Table 1, entry 10).
Table 1 Initial studies of the photo-induced catalyst-free reaction of N-(2-iodophenyl)-N-methylmethacrylamide 1a, DABCO·(SO2)2 and morpholin-4-amine 2a
a
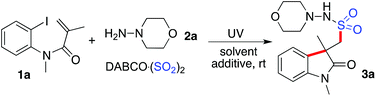
|
Entry |
Solvent |
Additive |
Yieldb (%) |
Reaction conditions: N-(2-iodophenyl)-N-methylmethacrylamide 1a (0.2 mmol), DABCO·(SO2)2 (0.16 mmol), morpholin-4-amine 2a (0.3 mmol), additive (0.3 mmol), solvent (4.0 mL), rt, 10 h.
Isolated yield based on N-(2-iodophenyl)-N-methylmethacrylamide 1a.
The reaction was performed in the presence of TBAI (1.0 equiv.).
|
1 |
DMF |
TBAI |
nr |
2 |
DMF |
TBAI |
Trace |
3 |
1,4-Dioxane |
TBAI |
Trace |
4 |
CH2Cl2 |
TBAI |
nr |
5 |
ClCH2CH2Cl |
TBAI |
nr |
6 |
MeCN |
TBAI |
71 |
7 |
MeCN |
— |
Trace |
8 |
MeCN |
TBAB |
Trace |
9 |
MeCN |
TBAC |
Trace |
10c |
MeCN |
TBAI |
68 |
We next explored the scope of the photo-induced catalyst-free three-component reaction of N-(2-iodoaryl)acrylamides 1, sulfur dioxide, and hydrazines 2 under the above optimized reaction conditions. The result is shown in Table 2. Various N-(2-iodoaryl)acrylamides 1 reacted with DABCO·(SO2)2 and hydrazines 2 efficiently, leading to the corresponding (2-oxoindolin-3-yl)methanesulfonohydrazides 3 in moderate to good yields. At the beginning, a range of N-(2-iodoaryl)acrylamides 1 were examined in the reaction of sulfur dioxide and morpholin-4-amine 2a. It seemed that the reactions of N-(2-iodoaryl)acrylamides 1 with electron-donating groups on the aromatic ring gave rise to better results. For instance, methyl-substituted (2-oxoindolin-3-yl)methanesulfonohydrazide 3b was obtained in 75% yield under the standard conditions, while nitro-substituted (2-oxoindolin-3-yl)methanesulfonohydrazide 3d was produced in 40% yield. The structure of compound 3b was identified via X-ray crystallography analysis (Fig. 1, also see the ESI†). Other hydrazines were examined subsequently, which were proven to be compatible under the standard conditions. Aryl bromide was applicable in the reaction as well. For instance, compound 3q was obtained in 39% yield when the corresponding N-(2-bromo-4-(trifluoromethoxy)phenyl)-N-methylmethacrylamide was employed under the standard conditions.
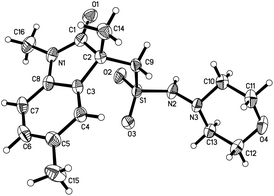 |
| Fig. 1 ORTEP illustration of compound 3b (30% probability ellipsoids). | |
Table 2 Scope investigation of the photo-induced catalyst-free three-component reaction of N-(2-iodoaryl)acrylamide 1, sulfur dioxide, and hydrazine 2
a
Isolated yield based on 2-alkynylbenzenesulfonamide 1.
|
|
We postulated that the reaction proceeded through a radical process. Thus, 2,2,6,6-tetramethyl-1-piperidinyloxy (TEMPO) was added to the reaction of N-(2-iodophenyl)-N-methylmethacrylamide 1a, DABCO·(SO2)2, and morpholin-4-amine 2a. As expected, the desired product 3a was not detected. According to this experimental observation and our previous reports,4j,n we proposed a plausible mechanism as shown in Scheme 2. We reasoned that aryl radical A and an iodo radical would be afforded when N-(2-iodoaryl)acrylamide 1 was treated under ultraviolet irradiation. Then intramolecular 5-exo radical cyclization would occur to provide alkyl radical B. In the meantime, an exchange of hydrazine 2 with DABCO·(SO2)2 would happen to produce intermediate C, which would react with alkyl radical B to generate intermediate D. The subsequent electron transfer would lead to the formation of sulfone radical E and hydrazine 2. The presence of the iodo radical would promote the generation of radical G, which would be trapped by sulfone radical E to afford the final product 3.
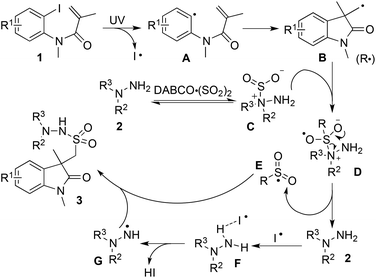 |
| Scheme 2 A proposed mechanism for the photo-induced three-component reaction of N-(2-iodoaryl)acrylamide 1, sulfur dioxide, and hydrazine 2. | |
Conclusions
In conclusion, we have described a catalyst-free photo-induced three-component reaction of N-(2-iodoaryl)acrylamide, sulfur dioxide, and hydrazine. Under ultraviolet irradiation, diverse (2-oxoindolin-3-yl)methanesulfonohydrazides are generated in moderate to good yields. During the process, the sulfonyl group can be easily incorporated via insertion of sulfur dioxide in the absence of any metals or photo-redox catalysts under mild conditions. A plausible mechanism is proposed, which involves 5-exo radical cyclization and the subsequent insertion of sulfur dioxide. The reaction scope with broad functional group tolerance is demonstrated. We believe that the photo-induced catalyst-free conditions would broaden the reaction types of insertion of sulfur dioxide, thus providing a new route for the fixation of sulfur dioxide.
Acknowledgements
Financial support from the National Natural Science Foundation of China (No. 21372046 and 21532001) is gratefully acknowledged.
Notes and references
- For reviews, see:
(a) T. P. Yoon, M. A. Ischay and J. Du, Nat. Chem., 2010, 2, 527 CrossRef CAS PubMed;
(b) D. M. Schultz and T. P. Yoon, Science, 2014, 343, 1239176 CrossRef PubMed;
(c) J. M. R. Narayanam and C. R. J. Stephenson, Chem. Soc. Rev., 2011, 40, 102 RSC;
(d) J. Xuan and W.-J. Xiao, Angew. Chem., Int. Ed., 2012, 51, 6828 CrossRef CAS PubMed;
(e) L. Shi and W. Xia, Chem. Soc. Rev., 2012, 41, 7687 RSC;
(f) Y.-M. Xi, H. Yi and A.-W. Lei, Org. Biomol. Chem., 2013, 11, 2387 RSC;
(g) C. K. Prier, D. A. Rankic and D. W. C. MacMillan, Chem. Rev., 2013, 113, 5322 CrossRef CAS PubMed;
(h) D. M. Schultz and T. P. Yoon, Science, 2014, 343, 985 CrossRef CAS PubMed;
(i) M. N. Hopkinson, B. Sahoo, J.-L. Li and F. Glorius, Chem. – Eur. J., 2014, 20, 3874 CrossRef CAS PubMed;
(j) J. Xuan, Z.-G. Zhang and W.-J. Xiao, Angew. Chem., Int. Ed., 2015, 54, 15632 CrossRef CAS PubMed;
(k) J.-R. Chen, X.-Q. Hu, L.-Q. Lu and W.-J. Xiao, Chem. Soc. Rev., 2016, 45, 2044 RSC;
(l) S. Protti, M. Fagnoni and D. Ravelli, ChemCatChem, 2015, 7, 1516 CrossRef CAS;
(m) M. D. Karkas, B. S. Matsuura and C. R. J. Stephenson, Science, 2015, 349, 1285 CrossRef CAS PubMed;
(n) R. Brimioulle, D. Lenhart, M. M. Maturi and T. Bach, Angew. Chem., Int. Ed., 2015, 54, 3872 CrossRef CAS PubMed;
(o) D. Ravelli, M. Fagnoni and A. Albini, Chem. Soc. Rev., 2013, 42, 97 RSC;
(p) T. P. Yoon, ACS Catal., 2013, 3, 895 CrossRef CAS PubMed;
(q) M. A. Ischay and T. P. Yoon, Eur. J. Org. Chem., 2012, 3359 CrossRef CAS;
(r) N. Hoffmann, ChemSusChem, 2012, 5, 352 CrossRef CAS PubMed;
(s) J. W. Tucker and C. R. J. Stephenson, J. Org. Chem., 2012, 77, 1617 CrossRef CAS PubMed.
- For selected examples, see:
(a) C. Uyeda, Y. Tan, G. C. Fu and J. C. Peters, J. Am. Chem. Soc., 2013, 135, 9548 CrossRef CAS PubMed;
(b) S. E. Creutz, K. J. Lotito, G. C. Fu and J. C. Peters, Science, 2012, 338, 647 CrossRef CAS PubMed;
(c) D. T. Ziegler, J. Choi, J. M. Muñoz-Molina, A. C. Bissember, J. C. Peters and G. C. Fu, J. Am. Chem. Soc., 2013, 135, 13107 CrossRef CAS PubMed;
(d) J. A. Terrett, J. D. Cuthbertson, V. W. Shurtleff and D. W. C. MacMillan, Nature, 2015, 524, 330 CrossRef CAS PubMed;
(e) C. C. Nawrat, C. R. Jamison, Y. Slutskyy, D. W. C. MacMillan and L. E. Overman, J. Am. Chem. Soc., 2015, 137, 11270 CrossRef CAS PubMed;
(f) J. Jin and D. W. C. MacMillan, Nature, 2015, 525, 87 CrossRef CAS PubMed;
(g) X.-Q. Hu, J.-R. Chen, Q. Wei, F.-L. Liu, Q.-H. Deng, A. M. Beauchemin and W.-J. Xiao, Angew. Chem., Int. Ed., 2014, 53, 12163 CrossRef CAS;
(h) Y. Masuda, N. Ishida and M. Murakami, J. Am. Chem. Soc., 2015, 137, 14063 CrossRef CAS PubMed;
(i) M. El Khatib, R. A. M. Serafim and G. A. Molander, Angew. Chem., Int. Ed., 2016, 55, 254 CrossRef CAS PubMed;
(j) J. C. Tellis, D. N. Primer and G. A. Molander, Science, 2014, 345, 433 CrossRef CAS PubMed;
(k) H.-Q. Do, S. Bachman, A. C. Bissember, J. C. Peters and G. C. Fu, J. Am. Chem. Soc., 2014, 136, 2162 CrossRef CAS PubMed;
(l) A. C. Bissember, R. J. Lundgren, S. E. Creutz, J. C. Peters and G. C. Fu, Angew. Chem., Int. Ed., 2013, 52, 5129 CrossRef CAS PubMed.
- For selected examples, see:
(a) W. Liu, L. Li and C.-J. Li, Nat. Commun., 2015, 6, 6526 CrossRef CAS PubMed;
(b) L. Li, W. Liu, H. Zeng, X. Mu, G. Cosa, Z. Mi and C.-J. Li, J. Am. Chem. Soc., 2015, 137, 8328 CrossRef CAS PubMed;
(c) Y. Masuda, N. Ishida and M. Murakami, J. Am. Chem. Soc., 2015, 137, 14063 CrossRef CAS PubMed;
(d) J. Ruch, A. Aubin, G. Erbland, A. Fortunato and J.-P. Goddard, Chem. Commun., 2016, 52, 2326 RSC;
(e) A. M. Mfuh, J. D. Doyle, B. Chhetri, H. D. Arman and O. V. Larionov, J. Am. Chem. Soc., 2016, 138, 2985 CrossRef CAS PubMed;
(f) I. Ghosh, T. Ghosh, J. I. Bardagi and B. König, Science, 2014, 346, 725 CrossRef CAS PubMed;
(g) B. Guizzardi, M. Mella, M. Fagnoni and A. Albini, Chem. – Eur. J., 2003, 9, 1549 CrossRef CAS PubMed;
(h) X. Zheng, L. Yang, W. Du, A. Ding and H. Guo, Chem. – Asian J., 2014, 9, 439 CrossRef CAS PubMed;
(i) T. Kawamoto, A. Sato and I. Ryu, Chem. – Eur. J., 2015, 21, 14764 CrossRef CAS PubMed;
(j) S. Cai, Z. Xiao, J. Ou, Y. Shi and S. Gao, Org. Chem. Front., 2016, 3, 354 RSC;
(k) J. Kan, S. Huang, H. Zhao, J. Lin and W. Su, Sci. China: Chem., 2015, 58, 1329 CrossRef CAS.
-
(a) B. Nguyen, E. J. Emmet and M. C. Willis, J. Am. Chem. Soc., 2010, 132, 16372 CrossRef CAS PubMed;
(b) E. J. Emmet, C. S. Garcia-Rubia, B. Nguyen, A. B. Garcia-Rubia, R. Hayter and M. C. Willis, Org. Biomol. Chem., 2012, 10, 4007 RSC;
(c) L. Martial, Synlett, 2013, 1595 Search PubMed;
(d) W. Li, H. Li, P. Langer, M. Beller and X.-F. Wu, Eur. J. Org. Chem., 2014, 3101 CrossRef;
(e) W. Li, M. Beller and X.-F. Wu, Chem. Commun., 2014, 50, 9513 RSC;
(f) X. Wang, L. Xue and Z. Wang, Org. Lett., 2014, 16, 4056 CrossRef CAS PubMed;
(g) A. S. Deeming, C. J. Russell and M. C. Willis, Angew. Chem., Int. Ed., 2015, 54, 1168 CrossRef CAS PubMed;
(h) S. Ye and J. Wu, Chem. Commun., 2012, 48, 7753 RSC;
(i) S. Ye and J. Wu, Chem. Commun., 2012, 48, 10037 RSC;
(j) D. Zheng, Y. An, Z. Li and J. Wu, Angew. Chem., Int. Ed., 2014, 53, 2451 CrossRef CAS PubMed;
(k) S. Ye, H. Wang, Q. Xiao, Q. Ding and J. Wu, Adv. Synth. Catal., 2014, 356, 3225 CrossRef CAS;
(l) Y. An, D. Zheng and J. Wu, Chem. Commun., 2014, 50, 11746 RSC;
(m) D. Zheng, Y. Li, Y. An and J. Wu, Chem. Commun., 2014, 50, 8886 RSC;
(n) Y. Li, D. Zheng, Z. Li and J. Wu, Org. Chem. Front., 2016, 3, 574 RSC.
-
(a) A. Shavnya, K. D. Hesp, V. Mascitti and A. C. Smith, Angew. Chem., Int. Ed., 2015, 54, 13571 CrossRef CAS PubMed;
(b) A. S. Deeming, C. J. Russell and M. C. Willis, Angew. Chem., Int. Ed., 2016, 55, 747 CrossRef CAS PubMed;
(c) C. C. Chen and J. Waser, Org. Lett., 2015, 17, 736 CrossRef CAS PubMed;
(d) A. S. Deeming, C. J. Rusell, A. J. Hennessy and M. C. Willis, Org. Lett., 2014, 16, 150 CrossRef CAS PubMed;
(e) B. N. Rocke, K. B. Bahnck, M. Herr, S. Lavergne, V. Mascitti, C. Perreault, J. Polivkova and A. Shavnya, Org. Lett., 2014, 16, 154 CrossRef CAS PubMed;
(f) E. J. Emmett, B. R. Hayter and M. C. Willis, Angew. Chem., Int. Ed., 2013, 52, 12679 CrossRef CAS PubMed.
-
(a) E. J. Emmett, B. R. Hayter and M. C. Willis, Angew. Chem., Int. Ed., 2014, 53, 10204 CrossRef CAS PubMed;
(b) A. Shavnya, S. B. Coffey, A. C. Smith and V. Mascitti, Org. Lett., 2013, 15, 6226 CrossRef CAS PubMed;
(c) M. W. Johnson, S. W. Bagley, N. P. Mankad, R. G. Bergman, V. Mascitti and F. D. Toste, Angew. Chem., Int. Ed., 2014, 53, 4404 CrossRef CAS PubMed;
(d) Y. Luo, X. Pan, C. Chen, L. Yao and J. Wu, Chem. Commun., 2015, 51, 180 RSC;
(e) D. Zheng, R. Mao, Z. Li and J. Wu, Org. Chem. Front., 2016, 3, 359 RSC;
(f) A. S. Tsai, J. M. Curto, B. N. Rocke, A. R. Dechert-Schmitt, G. K. Ingle and V. Mascitti, Org. Lett., 2016, 18, 508 CrossRef CAS PubMed;
(g) W. Zhang and M. Luo, Chem. Commun., 2016, 52, 2980 RSC.
- For reviews:
(a) G. Liu, C. Fan and J. Wu, Org. Biomol. Chem., 2015, 13, 1592 RSC;
(b) P. Bisseret and N. Blanchard, Org. Biomol. Chem., 2013, 11, 5393 RSC;
(c) A. S. Deeming, E. J. Emmett, C. S. Richards-Taylor and M. C. Willis, Synthesis, 2014, 2701 Search PubMed.
-
(a)
M. Bartholow, Top 200 Drugs of 2011. Pharmacy Times. http://www.pharmacytimes.com/publications/issue/2012/July2012/Top-200-Drugs-of-2011, accessed on Jan 9, 2013;
(b) For a list of top drugs by year, see: http://cbc.arizona.edu/njardarson/group/top-pharmaceuticals-poster, accessed on Jan 9, 2013;
(c) J. Drews, Science, 2000, 287, 1960 CrossRef CAS PubMed.
-
(a) P. J. Crowley, J. Fawcett, B. M. Kariuki, A. C. Moralee, J. M. Percy and V. Salafia, Org. Lett., 2002, 4, 4125 CrossRef CAS PubMed;
(b)
N. S. Simpkins, Sulfones in Organic Synthesis, Pergamon Press, Oxford, 1993 Search PubMed.
- For selected reviews, see:
(a) H. Wang, Y. Kuang and J. Wu, Asian J. Org. Chem., 2012, 1, 302 CrossRef CAS;
(b) L. He, H. Nie, G. Qiu, Y. Gao and J. Wu, Org. Biomol. Chem., 2014, 12, 9045 RSC;
(c) G. Qiu, Q. Ding and J. Wu, Chem. Soc. Rev., 2013, 42, 5257 RSC;
(d) G. Qiu, Y. Kuang and J. Wu, Adv. Synth. Catal., 2014, 356, 3483 CrossRef CAS;
(e) G. Qiu and J. Wu, Chem. Rec., 2016, 16, 19 CrossRef CAS PubMed.
- For selected examples, see:
(a) P. R. Sebahar and R. M. Williams, J. Am. Chem. Soc., 2000, 122, 5666 CrossRef CAS;
(b) A. Fensome, R. Bender, J. Cohen, M. A. Collins, V. A. Mackner, L. L. Miller, J. W. Ullrich, R. Winneker, J. Wrobel, P. Zhang, Z. Zhang and Y. Zhu, Bioorg. Med. Chem. Lett., 2002, 12, 3487 CrossRef CAS PubMed;
(c) Z. Bian, C. C. Marvin, M. Pettersson and S. F. Martin, J. Am. Chem. Soc., 2014, 136, 14184 CrossRef CAS PubMed;
(d) C. R. Prakash, P. Theivendren and S. Raja, Pharmacol. Pharm., 2012, 3, 62 CrossRef CAS;
(e) L. Sun, N. Tran, F. Tang, H. App, P. Hirth, G. McMahon and C. Tang, J. Med. Chem., 1998, 41, 2588 CrossRef CAS PubMed.
Footnote |
† Electronic supplementary information (ESI) available: Experimental procedure and related data. CCDC 1443052. For ESI and crystallographic data in CIF or other electronic format see DOI: 10.1039/c6qo00163g |
|
This journal is © the Partner Organisations 2016 |
Click here to see how this site uses Cookies. View our privacy policy here.