DOI:
10.1039/C5QO00378D
(Research Article)
Org. Chem. Front., 2016,
3, 475-479
Ruthenium-catalyzed selective imine synthesis from nitriles and secondary alcohols under hydrogen acceptor- and base-free conditions†
Received
18th November 2015
, Accepted 1st February 2016
First published on 11th February 2016
Abstract
We report a method for the selective synthesis of imines from nitriles and secondary alcohols using a hydrogen-transfer strategy. The imine bond is efficiently formed between the nitrogen atom of nitrile and the α-carbon of secondary alcohol, catalyzed by a ruthenium dihydride complex with pyridine as a stabilizing ligand.
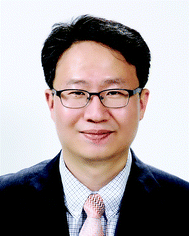 Soon Hyeok Hong | Soon Hyeok Hong received his BS, and MS from Seoul National University, South Korea. He then joined the Department of Chemistry at the Korea Air Force Academy as a full-time instructor to fulfill his military service. After completion of the service, he continued his studies at California Institute of Technology, where he obtained his PhD (2007), under the guidance of Professor Robert H. Grubbs. After having postdoctoral research experience with Professor J. Fraser Stoddart at the University of California, Los Angeles, he gained industrial experience at Materia, Inc. He began his independent career in academia as a Nanyang Assistant Professor at the Nanyang Technological University with a joint appointment as a National Research Foundation Fellow of the Singapore government in 2008. In 2011, he moved back to the Seoul National University, where he has been working on organometallic catalysis in developing efficient, practical, and sustainable synthetic methods. |
Introduction
Imines are an important functional group in organic chemistry because their versatility and high reactivity facilitate various transformations in laboratory and industrial synthetic processes.1 Conventionally, imines are synthesized from the condensation of an aldehyde or ketone with a primary amine in the presence of an acid catalyst.2 Moreover, imines have been prepared by oxidation of secondary amines,3 self-condensation of primary amines,3j,l–n,4 the aza-Wittig reaction,5 and the dehydrogenative coupling reaction between amines and alcohols.3m,n,6
Catalytic methods to convert nitriles to imines have been relatively less explored in organic synthesis. Symmetrical and unsymmetrical imines have been synthesized by coupling nitriles with the corresponding reduced amines or additional amines under hydrogen pressure with liberation of NH3.7 Recently, Nikonov and co-workers reported the reaction of nitriles to imines using a cationic Ru complex; however, the alcohol scope was limited to only 2-propanol and strong basic conditions were required to generate the active catalyst.8
We have been working on the development of atom-, step-, and redox-economical C–N bond formation methods for the synthesis of amides, formamides, and imides from nitriles and alcohols through a hydrogen-transfer strategy (Scheme 1).9 Further, Beller and co-workers reported N-monoalkylation of nitriles using 2-propanol as both the hydrogen source and the coupling partner.10 As an expansion of these studies, we herein report a selective, catalytic imine synthetic method from nitriles and secondary alcohols under base-free and hydrogen acceptor-free conditions.
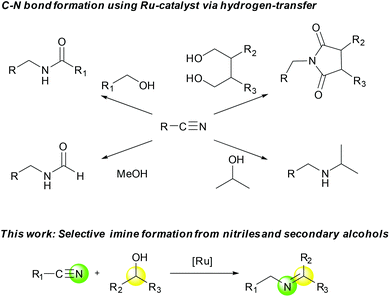 |
| Scheme 1 Imine synthesis directly from nitriles and secondary alcohols. | |
Results and discussion
Initially, the reaction between benzonitrile and 2-propanol was selected as a model reaction to investigate the catalytic conditions (Table 1 and ESI†). Molecular sieves (MS) were added to enable the continuous removal of water during the reaction. We first tested several Ru precatalysts that were known to be active for C–N bond formation from dehydrogenation of alcohols. Milstein catalyst and (NHC)Ru-based dehydrogenation catalytic systems yielded none of the desired imine products; however, N-benzylidenebenzylamine was obtained as a major product (Table 1 and entries 1 and 2).6k,7b When the RuCl2(PPh3)3-based catalytic system, which was reported as efficient for the N-alkylation of nitriles using 2-propanol under basic conditions, was applied without any base, it yielded only trace amounts of 4aa (2%, entry 3).10 We then found that the Ru(II) dihydride complex RuH2(CO)(PPh3)3 (1) showed significant activity for the imine synthesis (61%, entry 4). Previously, we reported N-heterocyclic carbene (NHC) coordinated Ru(II) dihydride complexes as efficient catalysts for the dehydrogenative amidation of nitriles with primary alcohols.9 A well-defined Ru dihydride complex, RuH2(CO)(PPh3)2(IiPr),11 gave 4aa in 60% yield (entry 5). After extensive screening (ESI†), an increased loading of the readily available precatalyst 1 (10 mol%) accompanied with pyridine as a stabilizing ligand was identified as the most efficient method for the imine synthesis (entry 6).
Table 1 Catalyst screeninga
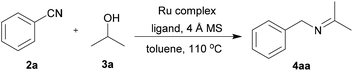
|
Entry |
Ru complex |
Ligand |
Time (h) |
Yieldb (%) |
Reaction conditions: 2a (0.5 mmol, 1.0 equiv.), 3a (5 equiv.), Ru complex (5 mol%), ligand (20 mol%), 4 Å molecular sieves (200 mg), toluene (0.6 mL), and 110 °C.
Determined by GC using dodecane as the internal standard.
Milstein catalyst: carbonylhydrido[6-(di-tert-butylphosphinomethylene)-2-(N,N-diethylamino methyl)-1,6-dihydropyridine]ruthenium(II).
RuCl2(IiPr)(p-cymene) (5 mol%), and 1,4-diazabicyclo[2,2,2]octane (DABCO, 10 mol%).
IiPr = 1,3-diisopropylimidazolidene.
10 mol% of 1 was used.
|
1 |
Milstein catalystc |
|
18 |
0 |
2 |
RuCl2(IiPr)(p-cymene)d |
|
18 |
0 |
3 |
RuCl2(PPh3)3 |
|
18 |
2 |
4 |
RuH2(CO)(PPh3)3 (1) |
|
18 |
61 |
5 |
RuH2(CO)(PPh3)2(IiPr)e |
|
18 |
60 |
6 |
RuH2(CO)(PPh3)3 (1)f |
Pyridine |
1 |
84 |
With the optimized conditions in hand, the substrate scope was then investigated. First, different nitriles were tested with 2-propanol as the coupling partner (Table 2). Various aromatic and aliphatic nitriles afforded the corresponding imines in moderate to good yields (entries 1–10). o-Tolunitrile showed a slightly decreased reactivity and required a longer reaction time than m-, and p-tolunitrile, presumably because of increased steric hindrance near the reaction center (entry 2). Electron-rich benzonitriles exhibited high reactivity (entries 2, 3 and 4), whereas electron-deficient nitriles gave diminished yields (entries 5 and 6). Aliphatic nitriles, which are less prone to reduction than aryl nitriles, also gave good yields (entries 7 and 8). However, propionitrile showed poor yield (entry 9). We think that the linear alkyl nitrile might coordinate to the catalytically active Ru complex stronger than other nitriles resulting in reduced catalytic activity (Table S1,† entry 22).12 Furthermore, a nitrile with a pyridine ring (2l) reacted smoothly under the reaction conditions (entry 10).
Table 2 Imine synthesis from 2-propanol and nitrilesa
Next, the reactions between benzonitrile and various secondary alcohols were investigated (Table 3). Various aliphatic secondary alcohols generated the corresponding imines in moderate to good yields (entries 1–6). Relatively sterically congested secondary alcohols such as 3-pentanol and cyclooctanol could also be used (entries 3 and 6). In the case of 1-phenylethanols, electron-donating 1-(4-methylphenyl)ethanol and 1-(3-methylphenyl)ethanol gave good yields but sterically congested 1-(2-methylphenyl)ethanol exhibited reduced activity (entry 8).
Table 3 Imine synthesis from benzonitrile and alcoholsa

|
Entry |
Alcohol |
Product |
Yieldb (%) |
Reaction conditions: 2a (0.5 mmol, 1.0 equiv.), alcohol (5.0 equiv.), 1 (10 mol%), pyridine (20 mol%), 4 Å molecular sieves (200 mg), toluene (0.6 mL), 110 °C, and 1 h reaction time.
Determined by 1H NMR with 1,2-dimethoxybenzene as the internal standard.
6 h reaction time.
12 h reaction time.
Determined by 1H NMR with nitrobenzene as the internal standard.
|
1 |
|
|
81c |
2 |
|
|
71c |
3 |
|
|
66c |
4 |
|
|
84 |
5 |
|
|
85 |
6 |
|
|
89c |
7 |
|
|
75d,e |
8 |
|
|
76d,e |
73d,e |
17d,e |
To investigate the reaction mechanism, a kinetic study was conducted by monitoring the progress of the reaction between 2a and 3a (Fig. 1). We found that 2a was rapidly consumed in 30 min, and benzylamine (5) was observed as a major intermediate. The concentration of 5 decreased whereas that of the imine (4aa) gradually increased and became saturated within 1 h. A small amount of N-benzylidenebenzylamine (7) was formed from the coupling reaction between 2a and 5. Interestingly, we observed very low trace amounts of N-isopropylbenzylamine (6), which is a further hydrogenated form of 4aa. We verified that our catalytic system is not very active for hydrogenation of the N-alkyl imine 4aa, which leads to the selective formation of imine (Scheme 2). The results suggest that 1 can hydrogenate nitriles and N-protonated imine intermediates, but not N-alkylated imines under the reaction conditions used.
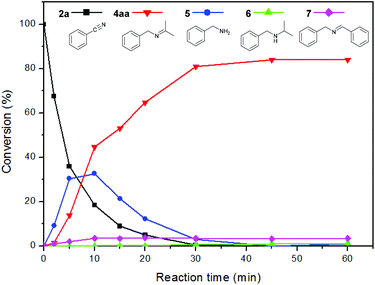 |
| Fig. 1 Reaction profiles for the imine synthesis showing the relative amounts of substrates and products. Reaction conditions: 2a (0.5 mmol, 1.0 equiv.), 3a (2.5 mmol, 5.0 equiv.), 1 (10 mol%), pyridine (20 mol%), 4 Å molecular sieves (200 mg), toluene (0.6 mL), and 110 °C. The reaction's progress was monitored by GC analysis using dodecane as the internal standard. | |
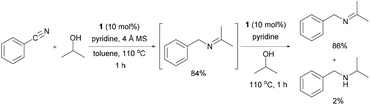 |
| Scheme 2 Attempted imine hydrogenation. Reaction conditions: (1) 2a (0.5 mmol, 1.0 equiv.), 3a (2.5 mmol, 5.0 equiv.), 1 (10 mol%), pyridine (20 mol%), 4 Å molecular sieves (200 mg), toluene (0.6 mL), 110 °C, 1 h. (2) 3a (2.5 mmol, 5.0 equiv.), 1 (10 mol%), pyridine (20 mol%), 110 °C, and 1 h reaction time. | |
A plausible mechanism is proposed on the basis of experimental observations (Scheme 3). At the initiation stage, hydrogen transfer from alcohol to nitrile affords the corresponding amine with simultaneous generation of ketone. Then, the generated amine undergoes coupling with ketone to produce the hemiaminal intermediate, which is further dehydrated to imine.
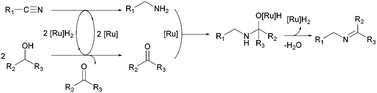 |
| Scheme 3 Proposed mechanism. | |
Conclusions
We have developed a Ru dihydride-complex-based catalytic system that works without any external base or hydrogen acceptor for the synthesis of imines from nitriles and secondary alcohols. The developed catalytic system can selectively control the pathway to the corresponding imine. This reaction offers a simple, convenient, and environmentally benign synthetic method for the synthesis of versatile imines by applying a hydrogen-transfer strategy.
Acknowledgements
This research was supported by the National Research Foundation of Korea (NRF-2015M1A8A1048891; NRF-2015M3D3A1A01065480; NRF-2014R1A5A1011165), funded by the Korean Government.
Notes and references
-
(a) J. P. Adams, J. Chem. Soc., Perkin Trans. 1, 2000, 125 RSC;
(b) G. Alvaro and D. Savoia, Synlett, 2002, 1180 Search PubMed;
(c) J. A. Ma, Chem. Soc. Rev., 2006, 35, 630 RSC;
(d) A. Erkkila, I. Majander and P. M. Pihko, Chem. Rev., 2007, 107, 5416 CrossRef PubMed;
(e) G. K. Friestad and A. K. Mathies, Tetrahedron, 2007, 63, 2541 CrossRef CAS;
(f) S. Kobayashi, Y. Mori, J. S. Fossey and M. M. Salter, Chem. Rev., 2011, 111, 2626 CrossRef CAS PubMed;
(g) J. H. Xie, S. F. Zhu and Q. L. Zhou, Chem. Rev., 2011, 111, 1713 CrossRef CAS PubMed.
-
(a) M. M. Sprung, Chem. Rev., 1940, 26, 297 CrossRef CAS;
(b) R. W. Layer, Chem. Rev., 1963, 63, 489 CrossRef CAS.
-
(a) S. I. Murahashi, T. Naota and H. Taki, J. Chem. Soc., Chem. Commun., 1985, 613 RSC;
(b) P. Muller and D. M. Gilabert, Tetrahedron, 1988, 44, 7171 CrossRef;
(c) A. H. Ell, J. S. M. Samec, C. Brasse and J. E. Backvall, Chem. Commun., 2002, 1144 RSC;
(d) K. C. Nicolaou, C. J. N. Mathison and T. Montagnon, Angew. Chem., Int. Ed., 2003, 42, 4077 CrossRef CAS PubMed;
(e) J. S. M. Samec, A. H. Ell and J. E. Backvall, Chem. – Eur. J., 2005, 11, 2327 CrossRef CAS PubMed;
(f) H. Choi and M. P. Doyle, Chem. Commun., 2007, 745 RSC;
(g) S. I. Murahashi, Y. Okano, H. Sato, T. Nakae and N. Komiya, Synlett, 2007, 1675 CrossRef CAS;
(h) G. Jiang, J. Chen, J. S. Huang and C. M. Che, Org. Lett., 2009, 11, 4568 CrossRef CAS PubMed;
(i) L. Aschwanden, T. Mallat, M. Maciejewski, F. Krumeich and A. Baiker, ChemCatChem, 2010, 2, 666 CrossRef CAS;
(j) R. D. Patil and S. Adimurthy, Adv. Synth. Catal., 2011, 353, 1695 CrossRef CAS;
(k) T. Sonobe, K. Oisaki and M. Kanai, Chem. Sci., 2012, 3, 3249 RSC;
(l) B. Huang, H. Tian, S. Lin, M. Xie, X. Yu and Q. Xu, Tetrahedron Lett., 2013, 54, 2861 CrossRef CAS;
(m) E. Zhang, H. Tian, S. Xu, X. Yu and Q. Xu, Org. Lett., 2013, 15, 2704 CrossRef CAS PubMed;
(n) B. Chen, L. Wang and S. Gao, ACS Catal., 2015, 5, 5851 CrossRef CAS.
-
(a) G. Chu and C. Li, Org. Biomol. Chem., 2010, 8, 4716 RSC;
(b) Q. L. Yuan, X. T. Zhou and H. B. Ji, Catal. Commun., 2010, 12, 202 CrossRef CAS;
(c) X. Lang, H. Ji, C. Chen, W. Ma and J. Zhao, Angew. Chem., Int. Ed., 2011, 50, 3934 CrossRef CAS PubMed;
(d) L. Liu, S. Zhang, X. Fu and C.-H. Yan, Chem. Commun., 2011, 47, 10148 RSC;
(e) A. Prades, E. Peris and M. Albrecht, Organometallics, 2011, 30, 1162 CrossRef CAS;
(f) Z. Hu and F. M. Kerton, Org. Biomol. Chem., 2012, 10, 1618 RSC;
(g) M. Largeron and M.-B. Fleury, Angew. Chem., Int. Ed., 2012, 51, 5409 CrossRef CAS PubMed;
(h) A. E. Wendlandt and S. S. Stahl, Org. Lett., 2012, 14, 2850 CrossRef CAS PubMed;
(i) M. Largeron and M. B. Fleury, Science, 2013, 339, 43 CrossRef PubMed;
(j) X. Qiu, C. Len, R. Luque and Y. Li, ChemSusChem, 2014, 7, 1684 CrossRef CAS PubMed;
(k) J. Wang, S. Lu, X. Cao and H. Gu, Chem. Commun., 2014, 50, 5637 RSC;
(l) B. Chen, L. Wang, W. Dai, S. Shang, Y. Lv and S. Gao, ACS Catal., 2015, 5, 2788 CrossRef CAS.
-
(a) P. M. Fresneda and P. Molina, Synlett, 2004, 1 CrossRef CAS;
(b) F. Palacios, C. Alonso, D. Aparicio, G. Rubiales and J. M. de los Santos, Tetrahedron, 2007, 63, 523 CrossRef CAS.
-
(a) L. Blackburn and R. J. K. Taylor, Org. Lett., 2001, 3, 1637 CrossRef CAS PubMed;
(b) J. W. Kim, J. He, K. Yamaguchi and N. Mizuno, Chem. Lett., 2009, 38, 920 CrossRef CAS;
(c) M. S. Kwon, S. Kim, S. Park, W. Bosco, R. K. Chidrala and J. Park, J. Org. Chem., 2009, 74, 2877 CrossRef CAS PubMed;
(d) H. Sun, F.-Z. Su, J. Ni, Y. Cao, H.-Y. He and K.-N. Fan, Angew. Chem., Int. Ed., 2009, 48, 4390 CrossRef CAS PubMed;
(e) B. Gnanaprakasam, J. Zhang and D. Milstein, Angew. Chem., Int. Ed., 2010, 49, 1468 CrossRef CAS PubMed;
(f) R. Cano, D. J. Ramon and M. Yus, J. Org. Chem., 2011, 76, 5547 CrossRef CAS PubMed;
(g) M. A. Esteruelas, N. Honczek, M. Olivan, E. Onate and M. Valencia, Organometallics, 2011, 30, 2468 CrossRef CAS;
(h) L. Jiang, L. Jin, H. Tian, X. Yuan, X. Yu and Q. Xu, Chem. Commun., 2011, 47, 10833 RSC;
(i) R. R. Donthiri, R. D. Patil and S. Adimurthy, Eur. J. Org. Chem., 2012, 4457 CrossRef CAS;
(j) Q. Kang and Y. Zhang, Green Chem., 2012, 14, 1016 RSC;
(k) A. Maggi and R. Madsen, Organometallics, 2012, 31, 451 CrossRef CAS;
(l) H. W. Tian, X. C. Yu, Q. Li, J. X. Wang and Q. Xu, Adv. Synth. Catal., 2012, 354, 2671 CrossRef CAS;
(m) J. Xu, R. Zhuang, L. Bao, G. Tang and Y. Zhao, Green Chem., 2012, 14, 2384 RSC;
(n) J.-F. Soule, H. Miyamura and S. Kobayashi, Chem. Commun., 2013, 49, 355 RSC;
(o) G. Zhang and S. K. Hanson, Org. Lett., 2013, 15, 650 CrossRef CAS PubMed;
(p) N. J. Oldenhuis, V. M. Dong and Z. Guan, Tetrahedron, 2014, 70, 4213 CrossRef CAS PubMed;
(q) B. Saha, S. M. W. Rahaman, P. Daw, G. Sengupta and J. K. Bera, Chem. – Eur. J., 2014, 20, 6542 CrossRef CAS PubMed;
(r) L. Wang, B. Chen, L. Ren, H. Zhang, Y. Lü and S. Gao, Chin.
J. Catal., 2015, 36, 19 CAS.
-
(a) R. Reguillo, M. Grellier, N. Vautravers, L. Vendier and S. Sabo-Etienne, J. Am. Chem. Soc., 2010, 132, 7854 CrossRef CAS PubMed;
(b) D. Srimani, M. Feller, Y. Ben-David and D. Milstein, Chem. Commun., 2012, 48, 11853 RSC;
(c) S. Chakraborty and H. Berke, ACS Catal., 2014, 4, 2191 CrossRef CAS;
(d) J. Long, B. Yin, Y. Li and L. Zhang, AIChE J., 2014, 60, 3565 CrossRef CAS;
(e) D. B. Bagal and B. M. Bhanage, Adv. Synth. Catal., 2015, 357, 883 CrossRef CAS;
(f) J.-H. Choi and M. H. G. Prechtl, ChemCatChem, 2015, 7, 1023 CrossRef CAS.
- S.-H. Lee and G. I. Nikonov, ChemCatChem, 2015, 7, 107 CrossRef CAS.
-
(a) B. Kang, Z. Fu and S. H. Hong, J. Am. Chem. Soc., 2013, 135, 11704 CrossRef CAS PubMed;
(b) J. Kim and S. H. Hong, Org. Lett., 2014, 16, 4404 CrossRef CAS PubMed;
(c) B. Kang and S. H. Hong, Adv. Synth. Catal., 2015, 357, 834 CrossRef CAS.
- S. Werkmeister, C. Bornschein, K. Junge and M. Beller, Eur. J. Org. Chem., 2013, 3671 CrossRef CAS.
- K. Kim, B. Kang and S. H. Hong, Tetrahedron, 2015, 71, 4565 CrossRef CAS.
- S. C. Ghosh, S. Muthaiah, Y. Zhang, X. Xu and S. H. Hong, Adv. Synth. Catal., 2009, 351, 2643 CrossRef CAS.
Footnote |
† Electronic supplementary information (ESI) available. See DOI: 10.1039/c5qo00378d |
|
This journal is © the Partner Organisations 2016 |