DOI:
10.1039/C5QO00329F
(Research Article)
Org. Chem. Front., 2016,
3, 165-169
Copper-mediated radical alkylarylation of unactivated alkenes with acetonitrile leading to fluorenes and pyrroloindoles†
Received
27th October 2015
, Accepted 6th December 2015
First published on 17th December 2015
Abstract
A Cu-mediated/catalyzed selective oxidative dual C–H bond cleavage of an arene and alkylnitrile or acetone is reported. This method provides a novel approach to highly functionalized fluorene and pyrroloindole derivatives, which are useful in pharmaceutical and photoelectronic areas. In this reaction, two new C(sp3)–C(sp3) and C(Ar)–C(sp3) bonds, a quaternary center and a five-membered ring are simultaneously formed.
Polycyclic aromatic hydrocarbons (PAHs) have been playing increasingly important roles in organic, medicinal and materials chemistry over the past few decades.1 Representative examples are fluorene and pyrroloindole derivatives (Fig. 1, I and II), which have excellent anti-inflammatory (III),2 antitumor (VII),3 and anticancer (VIII) activities4 and were confirmed as the cholesterol acyltransferase (ACAT) inhibitor (IV),5 cyclophilin A inhibitor (V)6 or S1P1 receptor agonist (VI),7etc. In addition, while these structural motifs are privileged in naturally occurring and biological active molecules, they also exhibit unique electrical and optical properties.8 As such, the development of practical and efficient methods for the synthesis of functionalized PAHs is urgently demanded.
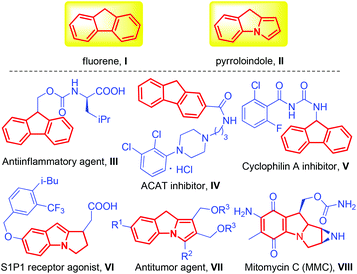 |
| Fig. 1 Representative bioactive related compounds exhibiting the fluorene or pyrroloindole motifs. | |
Several synthetic procedures to build fused cyclic compounds which suffered from limitations, such as tedious multistep synthesis, harsh reaction conditions, lack of regioselectivity, and poor functional group tolerance, were reported.9,10 Recently, extensive research attention has been paid to transition-metal-mediated cyclizations utilizing C–H bond activation (Scheme 1, A)11 or a direct dehydrogenative aryl–aryl coupling strategy (Scheme 1, B).12 Although intermolecular catalytic reactions were also reported (Scheme 1, C),13 the fast assembly of diverse fluorenes commencing from suitably functionalized starting materials is still an exciting goal because of its simple operation and atom economy.14 In this regard we report: (1) an autotandem radical process for the construction of polycyclic aromatic compounds through an oxidative C–C/C–C cross-coupling sequence with the participation of unactivated alkenes (it should be noted that the documented intermolecular dicarbonation is still restricted to oxindoles15 and related heterocycles16 using activated substrates); (2) an inexpensive Cu-mediated/catalyzed17 selective oxidative C–H bond cleavage of an arene and alkylnitrile; (3) two new C–C bonds, a quaternary center and a five-membered ring are simultaneously formed in one reaction; (4) an operationally simple and air benign catalyst system allowing access to various condensed carbo- or heterocycles as a promising scaffold for synthetic intermediates, pharmacophores, and organic photoelectronic materials (Scheme 1, D).
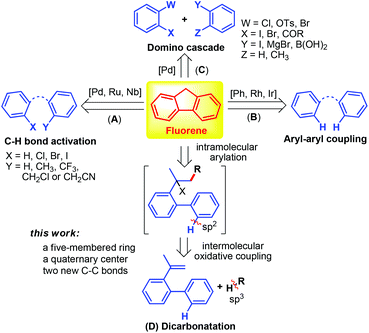 |
| Scheme 1 Metal-mediated routes to fluorenes. | |
The sequence of domino oxidative coupling of alkene 1a with acetonitrile (2a) and subsequent aryl intramolecular incorporation was established after conducting a variety of reactions (for detailed information on the optimization of the reaction conditions, see Table S1, ESI†). It was found that the presence of the nitrogen-containing ligand (1,10-phenanthroline) was critical to obtaining high yields of the target product. A reasonable explanation is that the cyano group of acetonitrile might coordinate strongly to a metal leading to catalyst poisoning.18 Screening of other parameters (ligand, base, catalyst, oxidant, etc.) substantially increased the yield of 3aa to 78%. In addition, this one-pot reaction can be scaled up to 2 mmol (70% yield) under an air atmosphere (Table 1, product 3aa).
Table 1 Rapid assembly of fluorenes from alkenes 1 and acetonitrile 2a
a
Reaction conditions: alkene 1 (0.2 mmol), 2a (2 mL), Cu(OTf)2 (0.1 mmol), 1,10-phenanthroline (0.2 mmol), K2CO3 (0.6 mmol) and di-tert-butyl peroxide (0.5 mmol) at 140 °C under air for 19 h; yields of the isolated products.
2 mmol scale.
Determined by 1H NMR analysis of the isolated products; only the major products are shown.
|
|
Encouraged by these results, next we set out to investigate the scope of various alkenes 1 in acetonitrile (Tables 1 and 2). As shown in Table 1, the substrates bearing electron-withdrawing or electron-donating groups always afforded the desired products in moderate to good yields (3aa–3na). The functionalities such as halogen, cyano, and ester groups at the p-(3aa–3ha), m-(3ia–3ka), or o-positions (3la–3na) of the aryl rings have no significant influence on the efficiency, thereby facilitating a chance for further modifications of the embedded functional groups. Notably, the use of substrates containing meta-substituents gave a mixture of two regioselective products (3ja/3ja′ and 3ka/3ka′). Moreover, substrates bearing heterocyclic rings including pyridine (1o), pyrimidine (1p) and thiophene (1q) were proven to be appropriate candidates, delivering the corresponding products 3oa–3qa in 37–68% yields. To our delight, when the benzene ring of 1 was changed to naphthalene (1r), phenanthrene (1s), dibenzo[b,d]furan (1t), dibenzo[b,d]thiophene (1u), triphenylamine (1v), and 9-phenyl-9H-carbazole (1w), the substrates were also successfully converted into the alkylarylated products 3ra–3wa. This methodology provides a concise entry to methylene-disubstituted fluorenes which are not easily accessed by the reported methods. More importantly, these new members of the family of π-conjugated polycyclic derivatives could be used as potential optoelectronic materials.1h,8d
Table 2 Substrate scope for the reaction of various alkenes 1 with 2a
a
Reaction conditions: alkene 1 (0.2 mmol), 2a (2 mL), Cu(OTf)2 (0.1 mmol), 1,10-phenanthroline (0.2 mmol), K2CO3 (0.6 mmol) and di-tert-butyl peroxide (0.5 mmol) at 140 °C under air for 19 h; yields of the isolated products.
Diastereomeric ratio was determined by 1H NMR spectroscopy of the isolated products.
30 mol% of Cu(OTf)2 was used.
|
|
The synthesis of fluorene 4aa with an ethyl group at the methylene moiety proceeded smoothly under optimal conditions, whereas simple styrene failed to afford the product 4ba (Table 2, 4aa–4ba). In addition, it was found that 2-vinylbiphenyls containing halogen atoms or derived from 1-(biphenyl-2-yl)propan-1-one were also compatible with the reaction conditions, leading to the tricyclic products 4ca and 4da in 66% and 57%, respectively. Interestingly, pyrroles and indoles could be facilely incorporated into these skeletons, which greatly streamlined the access to such fused pyrroloindoles (as electroluminescence materials8b and pharmaceutically relevant molecules3,4,7). Unfortunately, a series of other types of alkenes having an aliphatic chain (6–7) or double bonds (8) as the tether were not suitable. Apart from acetonitrile 2a, acetone 2b could also act as an effective substrate in this system with alkenes 1 (Table 3).19 The aryl-substituted alkenes 1 also worked well and mainly offered the desired products 5ab, 5db and 5sb in medium yield. Gratifyingly, the reaction was applicable to 5-(2-(prop-1-en-2-yl)phenyl)pyrimidine (1s), furnishing the product 5pb in 47% yield.
Table 3 Rapid assembly of fluorenes from alkenes 1 and acetone 2b
a
Reaction conditions: alkene 1 (0.3 mmol), 2a (2 mL), Cu(OTf)2 (0.03 mmol), 1,10-phenanthroline (0.06 mmol), Na3PO4 (0.3 mmol) and di-tert-butyl peroxide (0.75 mmol) at 120 °C under air for 24 h; yields of the isolated products.
|
|
It is noteworthy that the cyanomethylated products were widely applied in synthetic transformations (Scheme 2).20 For instance, an unexpected product of 9H-fluoren-9-ol 10 was synthesized from 3aa with the reduction of lithium aluminium hydride (Scheme 2, a). In addition, the cyano group can be easily hydrolyzed to amide in the KOH/tBuOH system (Scheme 2, b). Moreover, the photophysical properties of the prepared polycyclics were analysed by UV-Vis absorption photoluminescence measurements at room temperature in CH2Cl2 (Fig. 2).
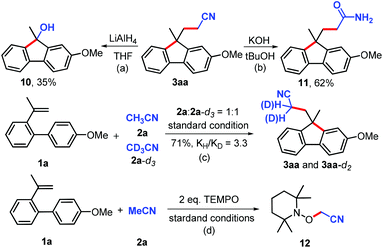 |
| Scheme 2 Further synthetic transformations and mechanism studies. | |
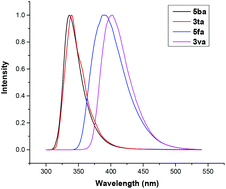 |
| Fig. 2 The fluorescence spectra of the prepared polycyclic compounds. | |
To get insight into the mechanism, a kinetic isotopic effect (KIE) study was conducted. The intermolecular kH/kD (3.3) indicated that the C(sp3)–H bond cleavage of acetonitrile should be the rate-determining step (Scheme 2, c). Furthermore, the reactions were completely inhibited in the presence of a radical scavenger 2,2,6,6-tetramethylpiperidine N-oxide (TEMPO) (Scheme 2, d), implying that a radical process might be involved in this transformation (for detailed information, see Fig. S2 and S3, ESI†). Initial mechanistic studies suggested that a free radical pathway (Scheme 3, path b) was more likely than a process involving the formation of an organocopper species (path a).17 Thus, initially, acetonitrile radical B (SOMO-π delocalization)17g which is thermodynamically stable, adds to alkene 1 to form a free radical D. Subsequently, an intramolecular cyclization from active alkyl radical D to intermediate E occurs; the former is kinetically competitive with TEMPO trapping, thus the TEMPO adduct of D was not observed.21 Finally, radical E was oxidized and followed by deprotonation to furnish fluorene 3.15,16
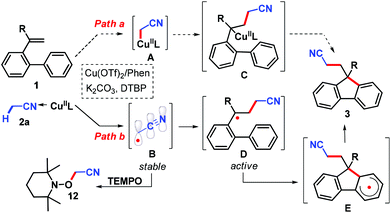 |
| Scheme 3 Proposed reaction mechanism. | |
In conclusion, we have developed a copper-mediated/catalyzed selective oxidative C–H bond functionalization reaction for a rapid assembly of fluorene and pyrroloindole derivatives from unactivated alkenes and acetonitrile. The reaction is general and practical because of its high reaction efficiency, broad substrate scope, the use of an inexpensive Cu-catalyst, and simple operation under air. More importantly, this methodology provides chemists an alternative method for designing new pharmaceutical frameworks and photoelectronic devices. Further studies to elucidate the detailed reaction mechanism and its application to the synthesis of complex products are ongoing in our group and will be disclosed in the near future.
We gratefully acknowledge the Natural Science Foundation of China (no. 21172162, 21372174), the Young National Natural Science Foundation of China (no. 21202113), the Ph.D. Programs Foundation of Ministry of Education of China (2013201130004), the Research Grant from the Innovation Project for Graduate Student of Jiangsu Province (KYZZ15_0322), PAPD, State and Local Joint Engineering Laboratory for Novel Functional Polymeric Materials and Soochow University for financial support.
Notes and references
-
(a)
R. G. Harvey, Polycyclic Aromatic Hydrocarbons, Wiley-VCH, Weinheim, 1997 Search PubMed;
(b) M. Toyota and N. Ihara, Nat. Prod. Rep., 1998, 15, 327 RSC;
(c) M. Bendikov, F. Wudl and D. F. Perepichka, Chem. Rev., 2004, 104, 4891 CrossRef CAS PubMed;
(d) D. Pérez and E. Guitián, Chem. Soc. Rev., 2004, 33, 274 RSC;
(e) J. E. Anthony, Chem. Rev., 2006, 106, 5028 CrossRef CAS PubMed;
(f)
Carbon-Rich Compounds, ed. M. M. Haley and R. R. Tykwinski, Wiley-VCH, Weinheim, 2006 Search PubMed;
(g) S. Sergeyev, W. Pisula and Y. H. Geerts, Chem. Soc. Rev., 2007, 36, 1902 RSC;
(h) C. Wang, H. Dong, W. Hu, Y. Liu and D. Zhu, Chem. Rev., 2012, 112, 2208 CrossRef CAS PubMed;
(i) J. R. Fulton, M. W. Bouwkamp and R. G. Bergman, J. Am. Chem. Soc., 2000, 122, 8799 CrossRef CAS PubMed;
(j) C. A. Fleckenstein and H. Plenio, Chem. – Eur. J., 2007, 13, 2701 CrossRef CAS PubMed.
- R. M. Burch, M. Weitzberg, N. Blok, R. Muhlhauser, D. Martin, S. G. Farmer, J. M. Bator, J. R. Connor, M. Green and C. Ko, Proc. Natl. Acad. Sci. U. S. A., 1991, 88, 355 CrossRef CAS.
- R. Kakadiya, H. Dong, P.-C. Lee, N. Kapuriya, X. Zhang, T.-C. Chou, T.-C. Lee, K. Kapuriya, A. Shah and T.-L. Su, Bioorg. Med. Chem., 2009, 17, 5614 CrossRef CAS PubMed.
-
(a) M. Tomasz and Y. Palom, Pharmacol. Ther., 1997, 76, 73 CrossRef CAS PubMed;
(b) W. T. Bradner, Cancer Treat. Rev., 2001, 27, 35 CrossRef CAS PubMed.
- A. K. Banala, B. A. Levy, S. S. Khatri, C. A. Furman, R. A. Roof, Y. Mishra, S. A. Griffin, D. R. Sibley, R. R. Luedtke and A. H. Newman, J. Med. Chem., 2011, 54, 3581 CrossRef CAS PubMed.
- S. Ni, Y. Yuan, J. Huang, X. Mao, M. Lv, J. Zhu, X. Shen, J. Pei, L. Lai, H. Jiang and J. Li, J. Med. Chem., 2009, 52, 5295 CrossRef CAS PubMed.
-
R. M. Jones, D. J. Buzard, A. M. Kawasaki, H. S. Kim, L. Thoresen, J. Lehmann and X. Zhu, WO2010027431, 2010 Search PubMed.
- For selected examples, see:
(a) U. Scherf and E. J. W. List, Adv. Mater., 2002, 14, 477 CrossRef CAS;
(b) T. Yoshihara, S. I. Druzhinin and K. A. Zachariasse, J. Am. Chem. Soc., 2004, 126, 8535 CrossRef CAS PubMed;
(c) R. Rathore, V. J. Chebny and S. H. Abdelwahed, J. Am. Chem. Soc., 2005, 127, 8012 CrossRef CAS PubMed;
(d) Y.-X. Xu, C.-C. Chueh, H.-L. Yip, F.-Z. Ding, Y.-X. Li, C.-Z. Li, X. Li, W.-C. Chen and A. K.-Y. Jen, Adv. Mater., 2012, 24, 6356 CrossRef CAS PubMed;
(e) M. J. Ingleson, D. Crossley, I. A. Cade, E. Clark, A. Escande, M. Humphries, S. King, I. Vitorica-Yrzebal and M. Turner, Chem. Sci., 2015, 6, 5144 RSC;
(f) G.-F. Zhang, T. Chen, Z.-Q. Chen, M. P. Aldred, X. Meng and M.-Q. Zhu, Chin. J. Chem., 2015, 33, 939 CrossRef CAS.
- For selected examples for the synthesis of fluorenes, see:
(a) S. Merlet, M. Birau and Z. Y. Wang, Org. Lett., 2002, 4, 2157 CrossRef CAS PubMed;
(b) D. L. J. Clive and R. Sunasee, Org. Lett., 2007, 9, 2677 CrossRef CAS PubMed;
(c) D. J. Gorin, I. D. G. Watson and F. D. Toste, J. Am. Chem. Soc., 2008, 130, 3736 CrossRef CAS PubMed;
(d) L. Xu, W. Yang, L. Zhang, M. Miao, Z. Yang, X. Xu and H. Ren, J. Org. Chem., 2014, 79, 9206 CrossRef CAS PubMed;
(e) Y. Wang, P. R. McGonigal, B. Herlé, M. Besora and A. M. Echavarren, J. Am. Chem. Soc., 2014, 136, 801 CrossRef CAS PubMed;
(f) X. Qin, X. Li, Q. Huang, H. Liu, D. Wu, Q. Guo, J. Lan, R. Wang and J. You, Angew. Chem., Int. Ed., 2015, 54, 7167 CrossRef CAS PubMed.
- For selected examples for the synthesis of pyrroloindoles, see:
(a) G. Abbiati, A. Casoni, V. Canevari, D. Nava and E. Rossi, Org. Lett., 2006, 8, 4839 CrossRef CAS PubMed;
(b) M. Tanaka, M. Ubukata, T. Matsuo, K. Yasue, K. Matsumoto, Y. Kajimoto, T. Ogo and T. Inaba, Org. Lett., 2007, 9, 3331 CrossRef CAS PubMed;
(c) S. J. Hwang, S. H. Cho and S. Chang, J. Am. Chem. Soc., 2008, 130, 16158 CrossRef CAS PubMed;
(d) L. Hao, Y. Pan, T. Wang, M. Lin, L. Chen and Z.-P. Zhan, Adv. Synth. Catal., 2010, 352, 3215 CrossRef CAS;
(e) N. Kern, M. Hoffmann, A. Blanc, J.-M. Weibel and P. Pale, Org. Lett., 2013, 15, 836 CrossRef CAS PubMed.
-
(a) L. C. Campeau, M. Parisien, A. Jean and K. Fagnou, J. Am. Chem. Soc., 2006, 128, 581 CrossRef CAS PubMed;
(b) S. J. Hwang, H. J. Kim and S. Chang, Org. Lett., 2009, 11, 4588 CrossRef CAS PubMed;
(c) M. Tobisu, Y. Kita, Y. Ano and N. Chatani, J. Am. Chem. Soc., 2008, 130, 15982 CrossRef CAS PubMed;
(d) K. Fuchibe and T. Akiyama, J. Am. Chem. Soc., 2006, 128, 1434 CrossRef CAS PubMed.
-
(a) C. S. Yeung and V. M. Dong, Chem. Rev., 2011, 111, 1215 CrossRef CAS PubMed;
(b) M. Itoh, K. Hirano, T. Satoh, Y. Shibata, K. Tanaka and M. Miura, J. Org. Chem., 2013, 78, 1365 CrossRef CAS PubMed.
-
(a) T.-P. Liu, C.-H. Xing and Q.-S. Hu, Angew. Chem., Int. Ed., 2010, 49, 2909 CrossRef CAS PubMed;
(b) Y.-B. Zhao, B. Mariampillai, D. A. Candito, B. Laleu, M. Li and M. Lautens, Angew. Chem., Int. Ed., 2009, 48, 1849 CrossRef CAS PubMed;
(c) C.-G. Dong and Q.-S. Hu, Angew. Chem., Int. Ed., 2006, 45, 2289 CrossRef CAS PubMed;
(d) C.-G. Dong and Q.-S. Hu, Org. Lett., 2006, 8, 5057 CrossRef CAS PubMed;
(e) R. Barroso, R. A. Valencia, M.-P. Cabal and C. Valdes, Org. Lett., 2014, 16, 2264 CrossRef CAS PubMed.
-
(a) A.-H. Zhou, F. Pan, C. Zhu and L.-W. Ye, Chem. – Eur. J., 2015, 21, 10278 CrossRef CAS PubMed;
(b) Z. Liu, H. Tan, L. Wang, T. Fu, Y. Xia, Y. Zhang and J. Wang, Angew. Chem., Int. Ed., 2015, 54, 3056 CrossRef CAS PubMed.
- For reviews, see:
(a) W. Mai, J. Wang, L. Yang, J. Yuan, P. Mao, Y. Xiao and L. Qu, Chin. J. Org. Chem., 2014, 34, 1958 CrossRef CAS;
(b) J.-R. Chen, X.-Y. Yu and W.-J. Xiao, Synthesis, 2015, 604 Search PubMed. For selected examples, see:
(c) S. Jaegli, J. Dufour, H. Wei, T. Piou, X. H. Duan, J. P. Vors, L. Neuville and J. Zhu, Org. Lett., 2010, 12, 4498 CrossRef CAS PubMed;
(d) T. Wu, X. Mu and G. Liu, Angew. Chem., Int. Ed., 2011, 50, 12578 CrossRef CAS PubMed;
(e) X. Mu, T. Wu, H. Wang, Y. Guo and G. Liu, J. Am. Chem. Soc., 2012, 134, 878 CrossRef CAS PubMed;
(f) W.-T. Wei, M.-B. Zhou, J.-H. Fan, W. Liu, R.-J. Song, Y. Liu, M. Hu, P. Xie and J.-H. Li, Angew. Chem., Int. Ed., 2013, 52, 3638 CrossRef CAS PubMed;
(g) S. L. Zhou, L. N. Guo, H. Wang and X. H. Duan, Chem. – Eur. J., 2013, 19, 12970 CrossRef CAS PubMed;
(h) H. Wang, L. N. Guo and X. H. Duan, Chem. Commun., 2013, 49, 10370 RSC;
(i) X. Xu, Y. Tang, X. Li, G. Hong, M. Fang and X. Du, J. Org. Chem., 2014, 79, 446 CrossRef CAS PubMed;
(j) J.-H. Fan, W.-T. Wei, M.-B. Zhou, R.-J. Song and J.-H. Li, Angew. Chem., Int. Ed., 2014, 53, 6650 CrossRef CAS PubMed;
(k) M.-Z. Lu and T.-P. Loh, Org. Lett., 2014, 16, 4698 CrossRef CAS PubMed;
(l) Y. Liu, J.-L. Zhang, R.-J. Song and J.-H. Li, Org. Chem. Front., 2014, 1, 1289 RSC;
(m) H. Zhang, P. Chen and G. Liu, Synlett, 2012, 2749 CAS.
-
(a) B. Zhang and A. Studer, Chem. Soc. Rev., 2015, 44, 3505 RSC;
(b) U. Wille, Chem. Rev., 2013, 113, 813 CrossRef CAS PubMed;
(c) K. C. Majumdar, P. K. Basu and P. P. Mukhopadhyay, Tetrahedron, 2004, 60, 6239 CrossRef CAS;
(d) X. Dong, R. Sang, Q. Wang, X.-Y. Tang and M. Shi, Chem. – Eur. J., 2013, 19, 16910 CrossRef CAS PubMed;
(e) S.-L. Zhou, L.-N. Guo, S. Wang and X. H. Duan, Chem. Commun., 2014, 50, 3589 RSC;
(f) Y. Li, Y. Lu, G. Qiu and Q. Ding, Org. Lett., 2014, 16, 4240 CrossRef CAS PubMed;
(g) G. Han, Y. Liu and Q. Wang, Org. Lett., 2014, 16, 3188 CrossRef CAS PubMed;
(h) H. Wang, Y. Yu, X. Hong and B. Xu, Chem. Commun., 2014, 50, 13485 RSC;
(i) T. F. Jamison, Angew. Chem., Int. Ed., 2014, 53, 14451 CrossRef PubMed;
(j) B. Zhang and A. Studer, Org. Lett., 2014, 16, 1216 CrossRef CAS PubMed;
(k) L. Lv, S. Lu, Q. X. Guo and Z. Li, J. Org. Chem., 2015, 80, 698 CrossRef CAS PubMed;
(l) N. Fuentes, W. Kong, L. Fernández-Sánchez, E. Merino and C. Nevado, J. Am. Chem. Soc., 2015, 137, 964 CrossRef CAS PubMed;
(m) Q.-H. Deng, J.-R. Chen, Q. Wei, Q.-Q. Zhao, L.-Q. Lu and W.-J. Xiao, Chem. Commun., 2015, 51, 3537 RSC;
(n) X.-Q. Chu, H. Meng, Y. Zi, X.-P. Xu and S.-J. Ji, Org. Chem. Front., 2015, 2, 216 RSC;
(o) W.-T. Wei, R.-J. Song, X.-H. Ouyang, Y. Li, H.-B. Li and J.-H. Li, Org. Chem. Front., 2014, 1, 484 RSC.
- For recent Cu-catalyzed selective oxidative C–H bond cleavages, see:
(a) Y. Zhu and Y. Wei, Chem. Sci., 2014, 5, 2379 RSC;
(b) A. K. Yadav and L. S. Yadav, Org. Biomol. Chem., 2015, 13, 2606 RSC;
(c) B. D. Barve, Y.-C. Wu, M. El-Shazly, M. Korinek, Y.-B. Cheng, J.-J. Wang and F.-R. Chang, Tetrahedron, 2015, 71, 2290 CrossRef CAS;
(d) S. Manna and A. P. Antonchick, Angew. Chem., Int. Ed., 2015, 54, 14845 CAS;
(e) W.-T. Wei, H.-B. Li, R.-J. Song and J.-H. Li, Chem. Commun., 2015, 51, 11325 RSC;
(f) A. Bunescu, Q. Wang and J. Zhu, Org. Lett., 2015, 17, 1890 CrossRef CAS PubMed;
(g) Z. Li, Y. Xiao and Z.-Q. Liu, Chem. Commun., 2015, 51, 9969 RSC;
(h) C. Chatalova-Sazepin, Q. Wang, G. M. Sammis and J. Zhu, Angew. Chem., Int. Ed., 2015, 54, 5443 CrossRef CAS PubMed.
-
(a) S.-I. Murahashi, T. Naota, H. Taki, M. Mizuno, H. Takaya, S. Komiya, Y. Mizuho, N. Oyasato, M. Hiraoka, M. Hirano and A. Fukuoka, J. Am. Chem. Soc., 1995, 117, 12436 CrossRef CAS;
(b) D. M. Tellers, J. C. M. Ritter and R. G. Bergman, Inorg. Chem., 1999, 38, 4810 CrossRef CAS PubMed;
(c) T. Naota, A. Tannna and S.-I. Murahashi, J. Am. Chem. Soc., 2000, 122, 2960 CrossRef CAS;
(d) T. Naota, A. Tannna and S.-I. Murahashi, Chem. Commun., 2001, 63 RSC;
(e) M. Kujime, S. Hikichi and M. Akita, Organometallics, 2001, 20, 4049 CrossRef CAS;
(f) M. Purzycki, W. Liu, G. Hilmersson and F. F. Fleming, Chem. Commun., 2013, 49, 4700 RSC.
- X.-W. Lan, N.-X. Wang, W. Zhang, J.-L. Wen, C.-B. Bai, Y. Xing and Y.-H. Li, Org. Lett., 2015, 17, 4460 CrossRef CAS PubMed.
-
(a)
K. Friedrich and K. Wallenfels, The Chemistry of the Cyano Group, Wiley-Interscience, New York, 1970 Search PubMed;
(b) R. J. H. Gregory, Chem. Rev., 1999, 99, 3649 CrossRef CAS PubMed.
- Trace amounts of the final products were observed suggesting that the acetonitrile radical is not completely consumed by TEMPO before the active alkyl radical D is generated.
Footnote |
† Electronic supplementary information (ESI) available. See DOI: 10.1039/c5qo00329f |
|
This journal is © the Partner Organisations 2016 |
Click here to see how this site uses Cookies. View our privacy policy here.