DOI:
10.1039/C5QO00313J
(Research Article)
Org. Chem. Front., 2016,
3, 82-86
Copper-mediated C(sp3)–H amination in a multiple C–N bond-forming strategy for the synthesis of N-heterocycles†
Received
13th October 2015
, Accepted 18th November 2015
First published on 20th November 2015
Abstract
An efficient construction of imidazo[1,5-a]pyridines, through a three-component reaction involving benzyl substituted pyridines, aldehydes, and TMSN3, has been developed. Three C–N bonds were formed in one pot. Copper-promoted amination of the benzylic C(sp3)–H bond is a key step of this multiple C–N bond-forming sequence.
Carbon–nitrogen bond formation is one of the major topics of organic synthesis due to the great importance of nitrogen-containing compounds in natural products, pharmaceuticals, and agrochemicals as well as functional chemicals.1 In recent years, transition-metal-catalyzed amination/amidation of C–H bonds has emerged as an atom-economical and efficient alternative to traditional C–N bond-forming methods using prefunctionalized substrates.2–4 Organic azides have been utilized increasingly as amino sources recently, since the method normally requires no extra oxidant and only N2 gas is released as a by-product.5 Additionally, structurally diversified organic azides are readily available. As a result, a variety of transition-metal-based catalytic systems have successfully been exploited in directed intermolecular C–H amination/amidation with organic azides.6–11 Although great success has been achieved in this field, some challenges still exist: (1) a process involving multiple C–N bond formation is uncommon; (2) the use of a directing group limits the application of this strategy; (3) intermolecular amidation/amination of the C(sp3)–H bond is still rare.12 Recently, a cascade intermolecular C(sp2)–H amidation and intramolecular N–N bond-forming strategy using organic azides was applied in the synthesis of indazoles.13,14 Herein, we report a new synthesis of imidazo[1,5-a]pyridines via amination of a sp3 hybridized C–H bond as a key C–N bond-forming step with TMSN3 as an amino source promoted by Cu(II) (Scheme 1). In this three-component reaction involving benzyl substituted N-heterocycles, aldehydes, and TMSN3, imidazo[1,5-a]pyridines and related more complicated fused heterocycles were constructed with sequential three C–N bonds formation. Besides, the heterocyclic nitrogen acted as both a directing group and an intramolecular nucleophile in this process.
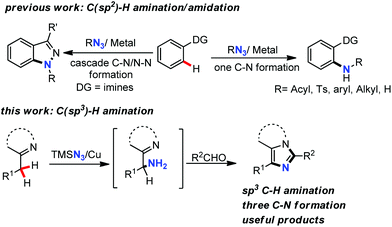 |
| Scheme 1 C–H amination/amidation for the construction of N-heterocycles. | |
The scaffold of imidazo[1,5-a]pyridine15 exists in many natural products and pharmaceutical agents with broad biological activities, such as antimicrobial, anti-neoplastic, anti-anxiety, and anti-inflammatory and consequently, much attention has been paid to its synthesis. Methods for the synthesis of imidazo[1,5-a]pyridines are mainly limited to 1,2,3-triazolo[1,5-a]pyridine-derived carbene insertion into nitriles, and Vilsmeier-type dehydrative cyclization of N-2-pyridylmethylamides and oxidative cyclization of 2-pyridinyl imine or amine derivatives.16 However, most of the starting materials applied in these methods require multiple-step preparations. The general procedure reported here starts from readily available substrates with C(sp3)–H amination as a key step.
We initiated the study with a reaction between 2-benzylpyridine 1a, p-chlorobenzaldehyde 2a, and TMSN3 under the reaction conditions we reported recently.10a The desired product, 3-(4-chlorophenyl)-1-phenylimidazo[1,5-a]pyridine 3a, was isolated in 14% yield. Then, different acids in place of PivOH were tested. PivOH was proved to be the additive of choice (entries 1–5, Table 1). DCB (o-dichlorobenzene) was a better solvent than the others screened (entries 6–8). Investigation of copper salts indicated that Cu(TFA)2·H2O was optimal. When the reaction was subjected to a catalytic amount of Cu(TFA)2·H2O (0.2 equiv.) with oxidants such as TBHP and dioxygen, the corresponding oxidation by-product, phenyl(pyridin-2-yl)methanone, was obtained and only a trace amount of the desired product 3a was detected. During the optimization, we found that 2-benzylpyridine was partially oxidized to the corresponding ketone. The yield of 3a was improved to 28% when the amount of 1a was doubled (entry 11). Addition of TMSN3 in two portions and increasing the loading of Cu(TFA)2 to 1.2 equiv. were helpful for product formation (entries 12 and 13). Finally, the yield was maximized to 72% when the reaction was performed in a higher concentration and a total of 4.0 equivalents of TMSN3 (3 + 1) were applied (entries 14 and 15).
Table 1 Optimization of the reaction conditionsa
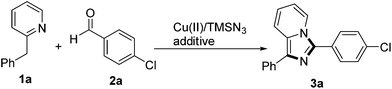
|
Entry |
Copper (equiv.) |
Additive (1 equiv.) |
Solvent (mL) |
Yield (%) |
Reaction conditions: 1a (0.2 mmol), 2a (0.2 mmol), copper salt, additive (1.0 equiv.), TMSN3 (3.0 equiv.), solvent, in Ar, 110 °C.
1a (0.4 mmol) and 2a (0.2 mmol).
Addition of TMSN3 (2.0 equiv.) at the beginning of the reaction, and the second portion of TMSN3 (1.0 equiv.) was added after 8 h.
Addition of TMSN3 (3.0 equiv.) at the beginning of the reaction, and the second portion of TMSN3 (1.0 equiv.) was added after 8 h. DCB = o-dichlorobenzene, PivOH = pivalic acid, TFA = trifluoroacetic acid, TsOH = p-toluenesulfonic acid, and HOTf = trifluoromethanesulfonic acid.
|
1 |
Cu(TFA)2 (1.0) |
PivOH |
DCB (2) |
14 |
2 |
Cu(TFA)2 (1.0) |
TFA |
DCB (2) |
n.d. |
3 |
Cu(TFA)2 (1.0) |
TsOH |
DCB (2) |
Trace |
4 |
Cu(TFA)2 (1.0) |
HOTf |
DCB (2) |
n.d. |
5 |
Cu(TFA)2 (1.0) |
— |
DCB (2) |
10 |
6 |
Cu(TFA)2 (1.0) |
PivOH |
Toluene (2) |
Trace |
7 |
Cu(TFA)2 (1.0) |
PivOH |
DMF (2) |
Trace |
8 |
Cu(TFA)2 (1.0) |
PivOH |
DCE (2) |
Trace |
9 |
Cu(OAc)2 (1.0) |
PivOH |
DCB (2) |
<5 |
10 |
CuCl2 (1.0) |
PivOH |
DCB (2) |
<5 |
11b |
Cu(TFA)2 (1.0) |
PivOH |
DCB (2) |
28 |
12b,c |
Cu(TFA)2 (1.0) |
PivOH |
DCB (2) |
32 |
13b,c |
Cu(TFA)2 (1.2) |
PivOH |
DCB (2) |
47 |
14b,c |
Cu(TFA)2 (1.2) |
PivOH |
DCB (0.3) |
60 |
15
,
|
Cu(TFA)
2
(1.2)
|
PivOH
|
DCB (0.3)
|
72
|
With the optimal conditions in hand, we started to examine the generality of aldehydes applicable to this three-component reaction (Scheme 2). Benzaldehydes substituted at the para position with either electron-donating OMe, Me or electron-withdrawing NO2 groups reacted smoothly with 1a and TMSN3 to give the corresponding products in 62% to 69% yield (3c–3e), demonstrating that the electronic density of the aromatic aldehydes had little effect on the product formation. Benzaldehydes bearing ortho substituents (Me, F) also gave the desired products (3f, 3g) in comparable yields. Furthermore, thiophene-2-carbaldehyde and furyl aldehyde afforded the corresponding heterocycle substituted products (3i, 3j) in 83% and 53% yield, respectively. Unfortunately, the aliphatic aldehydes failed to deliver the corresponding products with the method. It was notable that in these cases, no by-product, derived from either the Schmidt reaction17 or Jiao's reaction18 through C–C bond cleavage, was detected.
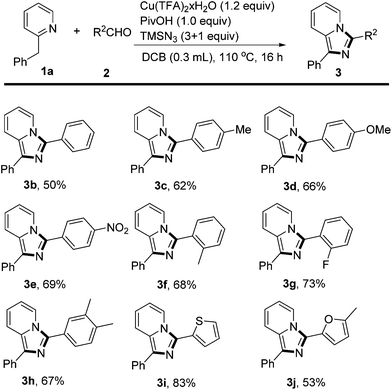 |
| Scheme 2 The scope of aldehydes. Reaction conditions: 1a (0.4 mmol, 2.0 equiv.), 2 (0.2 mmol, 1.0 equiv.), PivOH (0.2 mmol, 1.0 equiv.), Cu(TFA)2·H2O (0.24 mmol, 1.2 equiv.), DCB (0.3 mL), TMSN3 (0.6 mmol, 3.0 equiv., 8 h, then 0.2 mmol, 1.0 equiv., 8 h), 110 °C, in Ar. | |
Then, the scope of substituents on the phenyl ring of 2-benzylpyridine was studied (Scheme 3). Electron-rich 2-benzylpyridines afforded the corresponding products in higher yields than those substituted with electron-withdrawing CF3 or F groups in reactions with p-chlorobenzaldehyde 2a (3m and 3nvs. 3k and 3l). Besides pyridine, other N-heterocycles could also be used to build fused heterocyclic systems. For example, 2-benzylbenzothiazole and 2-benzylbenzoxazole reacted smoothly with 2a to afford the more complicated tricyclic scaffolds 3o and 3p in good yields. Isoquinoline is an excellent directing group in this highly efficient C–N bond-forming reaction, delivering 3q in 90% yield. Substituted imidazo[1,5-a]pyrimidine derivative 3r could also be obtained in 60% yield by applying this method.
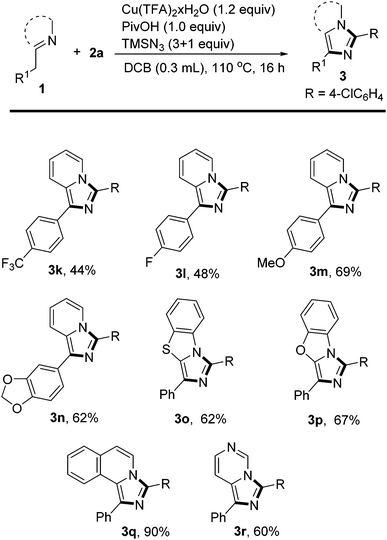 |
| Scheme 3 The scope of N-heterocycles. Reaction conditions: 1 (0.4 mmol, 2.0 equiv.), 2a (0.2 mmol, 1.0 equiv.), PivOH (0.2 mmol, 1.0 equiv.), Cu(TFA)2·H2O (0.24 mmol, 1.2 equiv.), DCB (0.3 mL), TMSN3 (0.6 mmol, 3.0 equiv., 8 h, then 0.2 mmol, 1.0 equiv., 8 h), 110 °C, in Ar. | |
In order to gain insights into the reaction mechanism, some possible reaction intermediates derived from benzaldehyde, such as benzonitrile, benzoic acid and benzamide, reacted with 1a and TMN3 under the standard conditions. No trace amount of the desired product was detected in these cases. Phenyl(pyridin-2-yl)methanone, the oxidation by-product of 1a, also failed to give 3a in a reaction with 2a and TMSN3. Then, azidation or amination of one of the benzylic C–H bonds was proposed as the initial step of this multiple C–N forming sequence. Therefore, the corresponding amino and azido substituted derivatives 4 and 5 were synthesized and tested (Scheme 4). Both 4 and 5 could react with 2a in the absence of TMSN3 to give the desired product 3a in 95% and 16% yield, respectively. However, the amino intermediate was not detected even in the absence of an aldehyde, probably due to its instability under the reaction conditions. We proposed that amination of the C(sp3)–H bond in 1a rather than azidation was likely the first step triggering the following processes.
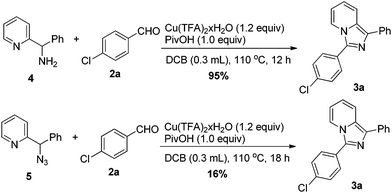 |
| Scheme 4 Reactions of possible intermediates. | |
Although the exact mechanism of this reaction is not completely clear at this stage, a plausible mechanism was proposed (Scheme 5).10a,12b Initially, coordination of Cu(II) with the pyridinyl nitrogen facilitates the deprotonation by the pivaloate anion to give intermediate A. Subsequently, the azido anion derived from HOPiv and TMSN3 may replace TFA in A to form intermediate B. The carbon–copper bond may be formed in intermediate Cvia 1,3-migration. With the assistance of acids, the first C–N bond was formed with the release of N2 as a driving force, delivering the aminated intermediate 5via intermediates D and E.19 Then, condensation of 5 with aldehydes followed by cycloaddition generates intermediate G. Finally, oxidative aromatization by Cu(II) gives the desired product 3.16d
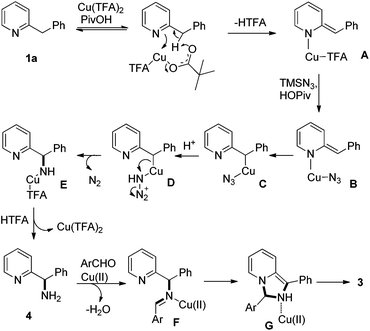 |
| Scheme 5 Proposed mechanism. | |
Conclusions
In conclusion, we have developed an efficient method for the synthesis of fused heterocycles containing the imidazole moiety starting from benzyl substituted N-heterocycles and aldehydes using TMSN3 as a nitrogen source. Copper-mediated amination of the sp3 hybridized C–H bond is the initial step for this multiple C–N bond-forming sequence. This methodology not only provides a useful approach for synthesis of imidazo[1,5-a]pyridines and related fused heterocycles, but also offers a new strategy for retro-synthetic analysis of certain N-heterocycles, in which multiple C–N bonds could be disconnected around aromatic nitrogen to yield simple and readily available feedstock chemicals as synthetic starting materials.
Acknowledgements
We are grateful for the financial support of the National Science Foundation of China (21272233, 21472190).
Notes and references
-
(a) R. Hili and A. K. Yudin, Nat. Chem. Biol., 2006, 2, 284 CrossRef CAS PubMed;
(b)
Amino Group Chemistry: From Synthesis to the Life Sciences, ed. A. Ricci, Wiley-VCH, Weinheim, Germany, 2007 Search PubMed;
(c) P. A. Gale, Acc. Chem. Res., 2006, 39, 465 CrossRef CAS PubMed.
-
(a)
Metal-Catalyzed Cross-Coupling Reactions, ed. A. Meijere and F. Diederich, Wiley-VCH, Weinheim, 2004 Search PubMed;
(b) S. V. Ley and A. W. Thomas, Angew. Chem., Int. Ed., 2003, 42, 5400 CrossRef CAS PubMed;
(c) J. P. Corbet and G. Mignani, Chem. Rev., 2006, 106, 2651 CrossRef CAS PubMed;
(d) E. M. Beccalli, G. Broggini, M. Martinelli and S. Sottocornola, Chem. Rev., 2007, 107, 5318 CrossRef CAS PubMed;
(e) J. F. Hartwig, Acc. Chem. Res., 2008, 41, 1534 CrossRef CAS PubMed;
(f) F. Monnier and M. Taillefer, Angew. Chem., Int. Ed., 2008, 47, 3096 CrossRef CAS PubMed;
(g) D. S. Surry and S. L. Buchwald, Angew. Chem., Int. Ed., 2008, 47, 6338 CrossRef CAS PubMed;
(h) F. Monnier and M. Taillefer, Angew. Chem., Int. Ed., 2009, 48, 6954 CrossRef CAS PubMed.
-
(a) S. H. Cho, J. Y. Kim, J. Kwak and S. Chang, Chem. Soc. Rev., 2011, 40, 5068 RSC;
(b) T. R. M. Rauws and B. U. W. Maes, Chem. Soc. Rev., 2012, 41, 2463 RSC;
(c) Z. Shi, C. Zhang, C. Tang and N. Jiao, Chem. Soc. Rev., 2012, 41, 3381 RSC;
(d) C. Zhang, C. Tang and N. Jiao, Chem. Soc. Rev., 2012, 41, 3464 RSC;
(e) G. Song, F. Wang and X. Li, Chem. Soc. Rev., 2012, 41, 3651 RSC;
(f) J. Bariwal and E. Van der Eycken, Chem. Soc. Rev., 2013, 42, 9283 RSC;
(g)
D. N. Zalatan and J. Du Bois, in Topics in Current Chemistry, ed. J. Q. Yu and Z. Shi, Springer, Berlin, 2010, vol. 292, pp. 347–378 Search PubMed.
-
(a) M. L. Louillat and F. W. Patureau, Chem. Soc. Rev., 2014, 43, 901 RSC;
(b) K. Takamatsu, K. Hirano, T. Satoh and M. Miura, Org. Lett., 2014, 16, 2892 CrossRef CAS PubMed;
(c) H. Xu, X. Qiao, S. Yang and Z. Shen, J. Org. Chem., 2014, 79, 4414 CrossRef CAS PubMed;
(d) I. Sokolovs, D. Lubriks and E. Suna, J. Am. Chem. Soc., 2014, 136, 6920 CrossRef CAS PubMed;
(e) J. Huang, T. Mao and Q. Zhu, Eur. J. Org. Chem., 2014, 2878 CrossRef CAS;
(f) C. Zhu, M. Yi, D. Wei, X. Chen, Y. Wu and X. Cui, Org. Lett., 2014, 16, 1840 CrossRef CAS PubMed;
(g) B. L. Tran, B. Li, M. Driess and J. F. Hartwig, J. Am. Chem. Soc., 2014, 136, 2555 CrossRef CAS PubMed;
(h) M. Shang, S. Sun, H. X. Dai and J. Q. Yu, J. Am. Chem. Soc., 2014, 136, 3354 CrossRef CAS PubMed.
-
(a) S. Cenini, E. Gallo, A. Caselli, F. Ragaini, S. Fantauzi and C. Piangiolino, Coord. Chem. Rev., 2006, 250, 1234 CrossRef CAS;
(b) S. Brase, C. Gil, K. Knepper and V. Zimmerman, Angew. Chem., Int. Ed., 2005, 44, 5188 CrossRef CAS PubMed;
(c) S. Lang and J. A. Murphy, Chem. Soc. Rev., 2006, 35, 146 RSC;
(d) K. Shin, H. Kim and S. Chang, Acc. Chem. Res., 2015, 48, 1040 CrossRef CAS PubMed.
- For selected Rh-catalyzed C–H amidation using organo azide:
(a) J. Y. Kim, S. H. Park, J. Ryu, S. H. Cho, S. H. Kim and S. Chang, J. Am. Chem. Soc., 2012, 134, 9110 CrossRef CAS PubMed;
(b) J. Ryu, K. Shin, S. H. Park, J. Y. Kim and S. Chang, Angew. Chem., Int. Ed., 2012, 51, 9904 CrossRef CAS PubMed;
(c) K. Shin, Y. Baek and S. Chang, Angew. Chem., Int. Ed., 2013, 52, 8031 CrossRef CAS PubMed;
(d) J. Jeong, P. Patel, H. Hwang and S. Chang, Org. Lett., 2014, 16, 4598 CrossRef CAS PubMed;
(e) H. Hwang, J. Kim, J. Jeong and S. Chang, J. Am. Chem. Soc., 2014, 136, 10770 CrossRef CAS PubMed.
- For selected Ir-catalyzed C–H amidation using organo azides:
(a) J. Kim, J. Kim and S. Chang, Chem. – Eur. J., 2013, 19, 7328 CrossRef CAS PubMed;
(b) V. S. Thirunavukkarasu, K. Raghuvanshi and L. Ackermann, Org. Lett., 2013, 15, 3286 CrossRef CAS PubMed;
(c) M. Bhanuchandra, M. R. Yadav, R. K. Rit, M. R. Kuram and A. K. Sahoo, Chem. Commun., 2013, 49, 5225 RSC;
(d) M. R. Yadav, R. K. Rit and A. K. Sahoo, Org. Lett., 2013, 15, 1638 CrossRef CAS PubMed;
(e) Q. Z. Zheng, Y. F. Liang, C. Qin and N. Jiao, Chem. Commun., 2013, 49, 5654 RSC;
(f) K. Shin, J. Ryu and S. Chang, Org. Lett., 2014, 16, 2022 CrossRef CAS PubMed;
(g) E. Brachet, T. Ghosh, I. Ghosh and B. Konig, Chem. Sci., 2015, 6, 987 RSC.
- For selected Ru-catalyzed C–H amidation using organo azides:
(a) J. Ryu, J. Kwak, K. Shin, D. Lee and S. Chang, J. Am. Chem. Soc., 2013, 135, 12861 CrossRef CAS PubMed;
(b) J. Kim and S. Chang, Angew. Chem., Int. Ed., 2014, 53, 2203 CrossRef CAS PubMed;
(c) T. Kang, Y. Kim, D. Kee, Z. Wang and S. Chang, J. Am. Chem. Soc., 2014, 136, 4141 CrossRef CAS PubMed;
(d) K. Shin and S. Chang, J. Org. Chem., 2014, 79, 12197 CrossRef CAS PubMed;
(e) H. Kim, J. Park, J. G. Kim and S. Chang, Org. Lett., 2014, 16, 5466 CrossRef CAS PubMed.
- For selected Co-catalyzed C–H amidation using organo azides:
(a) B. Sun, T. Yoshino, S. Matsunaga and M. Kanai, Adv. Synth. Catal., 2014, 356, 1491 CrossRef CAS;
(b) B. Sun, T. Yoshino, S. Matsunaga and M. Kanai, Chem. Commun., 2015, 51, 4649 Search PubMed.
- For selected Cu-catalyzed C–H amination/amidation using organo azides:
(a) J. Peng, M. Chen, Z. Xie, S. Luo and Q. Zhu, Org. Chem. Front., 2014, 1, 777 RSC;
(b) R. R. Donthiri, V. Pappula, N. N. K. Reddy, D. Bairagi and S. Adimurthy, J. Org. Chem., 2014, 79, 11277 CrossRef CAS PubMed;
(c) Y. M. Badiei, A. Dinescu, X. Dai, R. M. Palomino, F. W. Heinemann, T. R. Cundari and T. Warren, Angew. Chem., Int. Ed., 2008, 47, 9961 CrossRef CAS PubMed.
- Z. Hu, S. Luo and Q. Zhu, Sci. China: Chem., 2015, 58, 1349 CrossRef CAS.
- For selected recent papers on sp3 C–H amidation:
(a) X. Huang, Y. Wang, J. Lan and J. You, Angew. Chem., Int. Ed., 2015, 54, 9404 CrossRef CAS PubMed;
(b) H. M. L. Davies and J. R. Manning, Nature, 2008, 451, 417 CrossRef CAS PubMed;
(c) J. L. Roizen, M. E. Harvey and J. Du Bios, Acc. Chem. Res., 2012, 45, 911 CrossRef CAS PubMed;
(d) F. Collet, R. G. Dodd and P. Dauban, Chem. Commun., 2009, 5061 RSC;
(e) J. L. Jeffrey and R. Sarpong, Chem. Sci., 2013, 4, 4092 RSC;
(f) H. Wang, G. Tang and X. Li, Angew. Chem., Int. Ed., 2015, 54, 13049 CrossRef CAS PubMed.
-
(a) D. G. Yu, M. Suri and F. Glorius, J. Am. Chem. Soc., 2013, 135, 8802 CrossRef CAS PubMed;
(b) J. Peng, Z. Xie, S. Luo and Q. Zhu, Org. Lett., 2014, 16, 4702 CrossRef CAS PubMed.
- Selected papers on C–H azidation for the synthesis of heterocycles:
(a) Q. Z. Zheng, P. Feng, Y. F. Liang and N. Jiao, Org. Lett., 2013, 15, 4262 CrossRef CAS PubMed;
(b) F. Xie, Z. S. Qi and X. W. Li, Angew. Chem., Int. Ed., 2013, 52, 11862 CrossRef CAS PubMed;
(c) C. Tang and N. Jiao, J. Am. Chem. Soc., 2012, 134, 18924 CrossRef CAS PubMed;
(d) Y. Peng, W. Wan, G. Ma, W. Gao, H. Jiang, S. Zhu and J. Hao, Chem. Commun., 2014, 50, 5733 RSC;
(e) M. Devulapally, S. Pradeep and P. Tharmalingam, J. Org. Chem., 2015, 80, 1644 CrossRef PubMed.
- For selected recent biological applications, see:
(a) B. W. Trotter, Bioorg. Med. Chem. Lett., 2011, 21, 2354 CrossRef CAS PubMed;
(b) A. Kamalaaa, G. Ramakrishna and P. S. Ramakrishna, Eur. J. Med. Chem., 2011, 46, 2427 CrossRef PubMed;
(c) G. R. Pettit, J. C. Collins, J. C. Knight, D. L. Herald, R. A. Nieman, M. D. Williams and R. K. Pettit, J. Nat. Prod., 2003, 66, 544 CrossRef CAS PubMed;
(d) A. A. Kalinin, A. D. Voloshina, N. V. Kulik, V. V. Zobov and V. A. Mamedov, Eur. J. Med. Chem., 2013, 66, 345 CrossRef CAS PubMed.
-
(a) S. Chuprakov, F. Hwang and V. Gevorgyan, Angew. Chem., Int. Ed., 2007, 46, 4757 CrossRef CAS PubMed;
(b) Y. Shi, A. V. Gulevich and V. Gevorgyan, Angew. Chem., Int. Ed., 2014, 53, 14191 CrossRef CAS PubMed;
(c) G. Pelletier and A. B. Charette, Org. Lett., 2013, 15, 2290 CrossRef CAS PubMed;
(d) M. E. Bluhm, M. Ciesielski, H. Görls and M. Döring, Angew. Chem., Int. Ed., 2002, 41, 2962 CrossRef CAS;
(e) S. Fumitoshi, S. Rie, Y. Eiji, K. Asumi and M. Toshiaki, J. Org. Chem., 2009, 74, 3566 CrossRef PubMed;
(f) M. Li, Y. Xie, Y. Ye, Y. Zou, H. Jiang and W. Zeng, Org. Lett., 2014, 16, 6232 CrossRef CAS PubMed;
(g) Y. Yan, Y. Zhang, Z. Zha and Z. Wang, Org. Lett., 2013, 15, 2274 CrossRef CAS PubMed;
(h) S. Fumitoshi, K. Asumi, Y. Eiji and M. Toshiaki, Org. Lett., 2006, 8, 5621 CrossRef PubMed;
(i) D. S. Roman, V. Poiret, G. Pelletier and A. B. Charette, Eur. J. Org. Chem., 2015, 67 CrossRef.
-
Named Organic Reactions, ed. T. Laue and A. Plagens, John Wiley & Sons, Chichester, England, New York, 2005, vol. 2, p. 320 Search PubMed.
-
(a) F. Chen, T. Wang and N. Jiao, Chem. Rev., 2014, 114, 8613 CrossRef CAS PubMed;
(b) T. Wang and N. Jiao, Acc. Chem. Res., 2014, 47, 1137 CrossRef CAS PubMed;
(c) C. Tang and N. Jiao, Angew. Chem., Int. Ed., 2014, 53, 6528 CrossRef CAS PubMed;
(d) P. Feng, X. Sun, Y. Su, X. Li, L. H. Zhang, X. Shi and N. Jiao, Org. Lett., 2014, 16, 3388 CrossRef CAS PubMed;
(e) Y. Liang, Y. F. Liang and N. Jiao, Org. Chem. Front., 2015, 2, 403 RSC.
-
(a) S. B. Han, S. Park, K. Kim and S. Chang, J. Org. Chem., 2008, 73, 2862 CrossRef PubMed;
(b) K. M. Gillespie, E. J. Crust, R. J. Deeth and P. Scott, Chem. Commun., 2001, 785 RSC.
Footnote |
† Electronic supplementary information (ESI) available. See DOI: 10.1039/c5qo00313j |
|
This journal is © the Partner Organisations 2016 |
Click here to see how this site uses Cookies. View our privacy policy here.