DOI:
10.1039/C5QO00301F
(Research Article)
Org. Chem. Front., 2016,
3, 77-81
N-Heterocyclic carbene-catalyzed [4 + 2] annulation of α,β-unsaturated carboxylic acids: enantioselective synthesis of dihydropyridinones and spirocyclic oxindolodihydropyridinones†
Received
30th September 2015
, Accepted 14th November 2015
First published on 16th November 2015
Abstract
The N-heterocyclic carbene-catalyzed generation of dienoate from α,β-unsaturated carboxylic acid via the in situ formed mixed anhydride, and the following [4 + 2] annulation with hydrazones and isatin-derived imines was developed, affording the corresponding dihydropyridinones and spirocyclic oxindolodihydropyridinones, respectively, in moderate to good yields with good to excellent enantioselectivities.
Introduction
Dihydropyridinones and their derivatives are privileged motifs in numerous natural products1 and pharmacologically active compounds.2 Over the last few decades, many approaches for the preparation of enantioenriched dihydropyridinones have been developed via aza-Diels–Alder reactions by employing various chiral catalysts, such as Lewis acids,3 Brønsted acids,4 proline derivatives5 and isothiourea derivatives.6 Even so, the development of new methodologies for dihydropyridinones, especially those using stable and readily available starting materials, is still highly desired.
Over the past few decades, N-heterocyclic carbenes (NHCs) have emerged as remarkable organocatalysts for various reactions.7 The NHC-catalyzed synthesis of dihydropyridinones has been developed by the [2 + 4] annulation of aldehydes,8 enals,9 chloroaldehydes,10 ketenes11 and esters12 with azadienes, by the [3 + 3] annulation of enals,13 bromoenals,14 and α,β-unsaturated esters15 with enamines, and by the [4 + 2] annulation of α,β-unsaturated esters,16 cyclobutenones17 and γ-aryloxylenals18 with hydrazones or imines. In seeking stable and readily available starting materials for NHC catalysis, we developed the NHC-catalyzed generation of α,β-unsaturated acyl azolium from α,β-unsaturated carboxylic acids and its following [3 + 2] and [3 + 3] annulation with aminoketones and enamines (Scheme 1, reaction (a)).19 The NHC-catalyzed generation of enolate20 and homoenolate21 from carboxylic acids has also been established recently (reactions (b) and (c)). In this paper, we report the NHC-catalyzed generation of dienolates from α,β-unsaturated carboxylic acids, and their following [4 + 2] annulation with the C
N bond to give dihydropyridinones (reaction (d)).
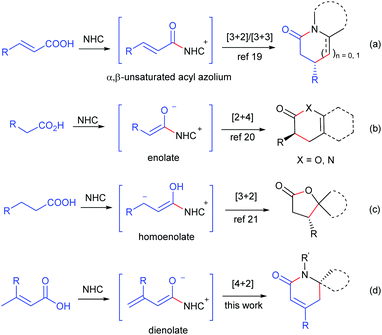 |
| Scheme 1 NHC-catalyzed generation of α,β-unsaturated acyl azolium, enolate, homoenolate and dienolate from carboxylic acids. | |
Results and discussion
Initially, α,β-unsaturated carboxylic acid 1a was treated with pivaloyl chloride to generate the mixed anhydride, which then reacted with hydrazone 2a under NHC catalysis (Table 1). We were encouraged to find that the desired dihydropyridinone 3a was obtained in 33% yield with 95% ee for the reaction catalyzed by 20 mol% of aminoindanol-derived preNHC A1 (entry 1). The preNHC B1 could also catalyze the reaction but resulted in very low yield albeit with high enantioselectivity (entry 2). Interestingly, the aminoindanol-derived preNHC A2 with the N-mesityl group led to a dramatic improvement of the yield (73%) with 97% ee (entry 3). Unexpectedly, the reaction using preNHC A3 with the N-pentafluorophenyl group gave only a trace of the desired product (entry 4). Several bases, such as DBU, TBD, DIPEA and Cs2CO3, were then screened but showed inferior performance than K2CO3 in terms of yield (entries 4–8). The reaction in THF gave better yield but with some loss of enantioselectivity (entry 9). Decreasing the loading of the NHC catalyst to 10 mol% or 5 mol% resulted in the loss of the yield but with high enantioselectivity maintained (entries 10 and 11).
Table 1 Condition optimization of the reaction with hydrazone 2a
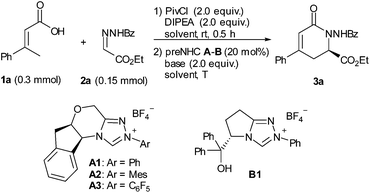
|
Entry |
NHC |
Base |
Solvent |
T
|
Yielda/% |
eeb/% |
Isolated yield.
Determined by HPLC on chiral columns.
Using 10 mol% A2 as the catalyst.
Using 5 mol% A2 as the catalyst. PivCl = pivaloyl chloride. TBD: 1,5,7-triazabicyclo[4.4.0]dec-5-ene. DBU: 1,8-diazabicyclo[5.4.0]undec-7-ene.
|
1 |
A1
|
K2CO3 |
DCM |
Reflux |
33 |
95 |
2 |
B1
|
K2CO3 |
DCM |
Reflux |
15 |
−91 |
3 |
A2
|
K2CO3 |
DCM |
Reflux |
73 |
97 |
4 |
A3
|
K2CO3 |
DCM |
Reflux |
Trace |
— |
5 |
A2
|
DBU |
DCM |
Reflux |
57 |
96 |
6 |
A2
|
TBD |
DCM |
Reflux |
34 |
97 |
7 |
A2
|
DIPEA |
DCM |
Reflux |
39 |
97 |
8 |
A2
|
Cs2CO3 |
DCM |
Reflux |
37 |
87 |
9 |
A2
|
K2CO3 |
THF |
45 °C |
88 |
95 |
10c |
A2
|
K2CO3 |
DCM |
Reflux |
50 |
95 |
11d |
A2
|
K2CO3 |
DCM |
Reflux |
35 |
95 |
With the optimized reaction conditions in hand, the substrate scope was briefly investigated (Table 2). It was found that both β-aryl-α,β-unsaturated acids with electron-donating (R = 4-MeC6H4 and 4-MeOC6H4) and electron-withdrawing groups (R = 4-ClC6H4 and 4-BrC6H4) worked well to give the desired products (3b–3e) in good to high yields with excellent enantioselectivities. Both bulky aryl groups (R = 2-ClC6H4, 2-naphthyl) and heteroaryl groups (R = 2-furyl, 2-thienyl) were also tolerated, affording dihydropyridinones 3f–3i in good yields with high enantioselectivities. The reaction of β-alkyl-α,β-unsaturated acids (R = methyl) also worked but in very low yield (23%) with 53% ee (3j). Replacement of the methyl group with the ethyl group of the unsaturated acid gave the dihydropyridinone 3k in 46% yield with 96% ee. The reaction with N-arylcarbonyl (Ar′ = 4-MeC6H4, 4-ClC6H4) or N-2-furylcarbonyl hydrazones also proceeded well, giving the corresponding dihydropyridinones 3l–3n in good yields with high enantioselectivities.
Table 2 NHC-catalyzed synthesis of dihydropyridinones
After establishing the reaction with hydrazones, we are interested in developing the reaction with oxindole-derived imines to give spirocyclic oxindoles, which are the key structural motifs found in many natural products and clinical pharmaceuticals.22 The model reaction of α,β-unsaturated carboxylic acid 1a with isatin-derived ketimine 4a was investigated under NHC catalysis (Table 3). Unfortunately, the desired spirocyclic oxindolodihydropyridinone 5a was isolated in low yield with only 7% ee when the previous conditions for hydrazone 2 were applied (entry 1). Considering the possible cooperative effort of the Lewis acid for the reaction,23 several additives were then investigated for the reaction. We were happy to find that the yield and enantioselectivity were dramatically improved when Sc(OTf)3 was added to the reaction (entry 2). A slightly better enantioselectivity was observed when the N-isopropylphenyl NHC precursor A4 was used instead of N-mesityl one A2 (entry 3). The L-pyroglutamic acid-derived NHC precursors B2 and B3 could also catalyze the reaction but with opposite enantioselectivities (entries 4 and 5). Further improvement of enantioselectivity was observed when Sn(OTf)2 or La(OTf)3 was used instead of Sc(OTf)3 (entries 6 and 7). The reaction performed worse in DCM or toluene than in THF (entries 7 vs. 8 and 9). Lowering the reaction temperature to room temperature or 0 °C resulted in a slight increase of enantioselectivity (entries 10 and 11).
Table 3 Condition optimization of the reaction with isatin-derived imines
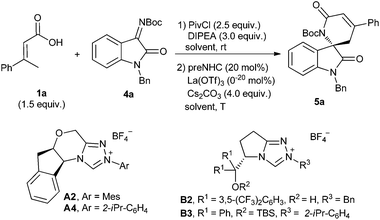
|
Entry |
Cat. |
Lewis acid |
Solvent |
T
|
Yielda/% |
eeb/% |
Isolated yield.
Determined by HPLC on chiral columns.
|
1 |
A2
|
— |
THF |
40 °C |
29 |
7 |
2 |
A2
|
Sc(OTf)3 |
THF |
40 °C |
78 |
71 |
3 |
A4
|
Sc(OTf)3 |
THF |
40 °C |
76 |
75 |
4 |
B2
|
Sc(OTf)3 |
THF |
40 °C |
76 |
−55 |
5 |
B3
|
Sc(OTf)3 |
THF |
40 °C |
75 |
−73 |
6 |
A4
|
Sn(OTf)2 |
THF |
40 °C |
68 |
84 |
7 |
A4
|
La(OTf)3 |
THF |
40 °C |
73 |
85 |
8 |
A4
|
La(OTf)3 |
DCM |
40 °C |
34 |
54 |
9 |
A4
|
La(OTf)3 |
Toluene |
40 °C |
45 |
65 |
10 |
A4
|
La(OTf)3 |
THF |
rt |
72 |
87 |
11 |
A4
|
La(OTf)3 |
THF |
0 |
70 |
92 |
With optimized reaction conditions in hand, various α,β-unsaturated carboxylic acids and isatin-derived imines were then evaluated (Table 4). It was found that the α,β-unsaturated carboxylic acid with electron-donating substituents (R = 4-MeC6H4, 3-MeC6H4) worked better than that with electron-withdrawing substituents (R = 4-BrC6H4, 3-ClC6H4) (5bvs.5c, and 5dvs.5e). The α,β-unsaturated carboxylic acid with a sterically hindered 1-naphthyl group also worked for the reaction, giving the corresponding product 5f in 58% yield with 83% ee. The reaction of β,β-dialkyl-α,β-unsaturated carboxylic acid (R = Me) gave the desired product 5g in very low yield and enantioselectivity. The isatin-derived ketimines with 5-chloro or N-Me instead of N-benzyl gave the corresponding products in moderate to good yields and enantioselectivities (5h–5i).
Table 4 Synthesis of spiro[oxindole-3,2′-dihydropyridinones]
A plausible catalytic cycle for the reaction is depicted in Fig. 1. The addition of NHC to the mixed anhydride 1′, generated in situ from the carboxylic acid, gives the α,β-unsaturated acyl azolium intermediate I. The deprotonation of the γ-C–H of the α,β-unsaturated acyl azolium intermediate I affords the dienolate intermediate II, which reacts with hydrazones 2 or imines 4 in a possible [4 + 2] cycloaddition manner to give adduct III. The fragmentation of adduct III furnishes the final dihydropyridinones 3 or 5 and regenerates the NHC catalyst.
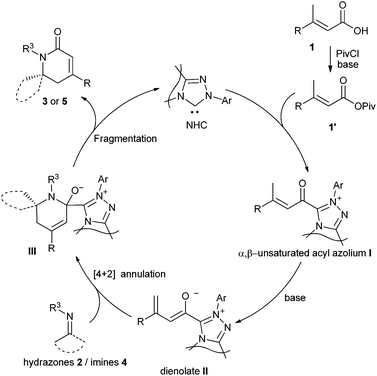 |
| Fig. 1 Plausible catalytic cycle. | |
The plausible stereochemical modes are depicted in Fig. 2. The bulky aryl group in triazolium may help to establish the configuration of the dienolate. The [4 + 2] cycloaddition of the electron-deficient C
N bond in hydrazones (TS A) or isatin imines (TS B) with the dienes from the less sterically hindered face results in the observed enantioselectivity.
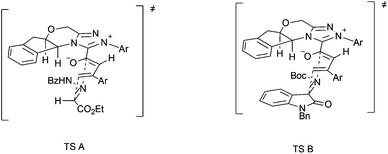 |
| Fig. 2 Stereochemical modes. | |
Conclusions
In summary, the N-heterocyclic carbene-catalyzed [4 + 2] annulation of α,β-unsaturated carboxylic acid was developed. The dienolate intermediate, which is generated from α,β-unsaturated carboxylic acids via in situ formed mixed anhydrides in the presence of N-heterocyclic carbene, reacted with hydrazones and imines to afford the dihydropyridinones and spirocylic oxindolodihydropyridinones, respectively, in moderate to good yields with good to excellent enantioselectivities. Further development of the NHC-catalyzed reactions of α,β-unsaturated carboxylic acids is underway in our laboratory.
Acknowledgements
Financial support from the National Science Foundation of China (no. 21425207 and 21272237), and the Chinese Academy of Sciences is greatly acknowledged.
Notes and references
-
(a) J. R. Lewis, Nat. Prod. Rep., 1994, 11, 329 RSC;
(b) G. R. Pettit, G. R. Pettit, G. Groszek, R. A. Backhaus, D. L. Doubek, R. J. Barr and A. W. Meerow, J. Nat. Prod., 1995, 58, 756 CrossRef CAS PubMed;
(c) S. Son, S.-K. Ko, M. Jang, J. K. Lee, I.-J. Ryoo, J.-S. Lee, K. H. Lee, N.-K. Soung, H. Oh, Y.-S. Hong, B. Y. Kim, J.-H. Jang and J. S. Ahn, Org. Lett., 2015, 17, 4046 CrossRef CAS PubMed.
-
(a) R. Kikumoto, Y. Tamao, T. Tezuka, S. Tonomura, H. Hara, K. Ninomiya, A. Hijikata and S. Okamoto, Biochemistry, 1984, 23, 85 CrossRef CAS PubMed;
(b) K. Hilpert, J. Ackermann, D. W. Banner, A. Gast, K. Gubernator, P. Hadvary, L. Labler, K. Mueller and G. Schmid, J. Med. Chem., 1994, 37, 3889 CrossRef CAS PubMed;
(c) C. Flexner, N. Engl. J. Med., 1998, 338, 1281 CrossRef CAS PubMed;
(d) P. L. Beaulieu, P. C. Anderson, D. R. Cameron, G. Croteau, V. Gorys, C. Grand-Maître, D. Lamarre, F. Liard, W. Paris, L. Plamondon, F. Soucy, D. Thibeault, D. Wernic, C. Yoakim, S. Pav and L. Tong, J. Med. Chem., 2000, 43, 1094 CrossRef CAS PubMed;
(e) M. A. Letavic, M. Z. Axt, J. T. Barberia, T. J. Carty, D. E. Danley, K. F. Geoghegan, N. S. Halim, L. R. Hoth, A. V. Kamath, E. R. Laird, L. L. Lopresti-Morrow, K. F. McClure, P. G. Mitchell, V. Natarajan, M. C. Noe, J. Pandit, L. Reeves, G. K. Schulte, S. L. Snow, F. J. Sweeney, D. H. Tan and C. H. Yu, Bioorg. Med. Chem. Lett., 2002, 12, 1387 CrossRef CAS PubMed.
-
(a) K. Hattori and H. Yamamoto, J. Org. Chem., 1992, 57, 3264 CrossRef CAS;
(b) S. Yao, M. Johannsen, R. G. Hazell and K. A. Jørgensen, Angew. Chem., Int. Ed., 1998, 37, 3121 CrossRef CAS;
(c) S. Kobayashi, K.-i. Kusakabe and H. Ishitani, Org. Lett., 2000, 2, 1225 CrossRef CAS PubMed;
(d) N. S. Josephsohn, M. L. Snapper and A. H. Hoveyda, J. Am. Chem. Soc., 2003, 125, 4018 CrossRef CAS PubMed;
(e) O. G. Mancheño, R. G. Arrayás and J. C. Carretero, J. Am. Chem. Soc., 2004, 126, 456 CrossRef PubMed;
(f) C. A. Newman, J. C. Antilla, P. Chen, A. V. Predeus, L. Fielding and W. D. Wulff, J. Am. Chem. Soc., 2007, 129, 7216 CrossRef CAS PubMed;
(g) H. Mandai, K. Mandai, M. L. Snapper and A. H. Hoveyda, J. Am. Chem. Soc., 2008, 130, 17961 CrossRef CAS PubMed.
- J. Itoh, K. Fuchibe and T. Akiyama, Angew. Chem., Int. Ed., 2006, 45, 4796 CrossRef CAS PubMed.
-
(a) H. Sundén, I. Ibrahem, L. Eriksson and A. Córdova, Angew. Chem., Int. Ed., 2005, 44, 4877 CrossRef PubMed;
(b) T. Itoh, M. Yokoya, K. Miyauchi, K. Nagata and A. Ohsawa, Org. Lett., 2003, 5, 4301 CrossRef CAS PubMed;
(c) T. Itoh, M. Yokoya, K. Miyauchi, K. Nagata and A. Ohsawa, Org. Lett., 2006, 8, 1533 CrossRef CAS PubMed.
- C. Simal, T. Lebl, A. M. Z. Slawin and A. D. Smith, Angew. Chem., Int. Ed., 2012, 51, 3653 CrossRef CAS PubMed.
-
(a) X. Bugaut and F. Glorius, Chem. Soc. Rev., 2012, 41, 3511 RSC;
(b) A. Grossmann and D. Enders, Angew. Chem., Int. Ed., 2012, 51, 314 CrossRef CAS PubMed;
(c) J. Izquierdo, G. E. Hutson, D. T. Cohen and K. A. Scheidt, Angew. Chem., Int. Ed., 2012, 51, 11686 CrossRef CAS PubMed;
(d) H. U. Vora, P. Wheeler and T. Rovis, Adv. Synth. Catal., 2012, 354, 1617 CrossRef CAS PubMed;
(e) J. W. Bode, Nat. Chem., 2013, 5, 813 CrossRef CAS PubMed;
(f) S. J. Ryan, L. Candish and D. W. Lupton, Chem. Soc. Rev., 2013, 42, 4906 RSC;
(g) S. Ye and X.-Y. Chen, Synlett, 2013, 1614 CrossRef;
(h) M. N. Hopkinson, C. Richter, M. Schedler and F. Glorius, Nature, 2014, 510, 485 CrossRef CAS PubMed;
(i) J. Mahatthananchai and J. W. Bode, Acc. Chem. Res., 2014, 47, 696 CrossRef CAS PubMed;
(j) D. M. Flanigan, F. Romanov-Michailidis, N. A. White and T. Rovis, Chem. Rev., 2015, 115, 9307 CrossRef CAS PubMed;
(k) K. Hirano, I. Piel and F. Glorius, Chem. Lett., 2011, 40, 786 CrossRef CAS;
(l) J.-L. Li, B. Sahoo, C.-G. Daniliuc and F. Glorius, Angew. Chem., Int. Ed., 2014, 53, 10515 CrossRef CAS PubMed.
- X. Zhao, K. E. Ruhl and T. Rovis, Angew. Chem., Int. Ed., 2012, 51, 12330 CrossRef CAS PubMed.
- M. He, J. R. Struble and J. W. Bode, J. Am. Chem. Soc., 2006, 128, 8418 CrossRef CAS PubMed.
-
(a) T.-Y. Jian, L.-H. Sun and S. Ye, Chem. Commun., 2012, 48, 10907 RSC;
(b) D.-L. Wang, Z.-Q. Liang, K.-Q. Chen, D.-Q. Sun and S. Ye, J. Org. Chem., 2015, 80, 5900 CrossRef CAS PubMed.
-
(a) T.-Y. Jian, P.-L. Shao and S. Ye, Chem. Commun., 2011, 47, 2381 RSC;
(b) L. Sun, Z. Liang and S. Ye, Acta Chim. Sin., 2014, 72, 841 CrossRef CAS.
- L. Hao, S. Chen, J. Xu, B. Tiwari, Z. Fu, T. Li, J. Lim and Y. R. Chi, Org. Lett., 2013, 15, 4956 CrossRef CAS PubMed.
-
(a) B. Wanner, J. Mahatthananchai and J. W. Bode, Org. Lett., 2011, 13, 5378 CrossRef CAS PubMed;
(b) A. G. Kravina, J. Mahatthananchai and J. W. Bode, Angew. Chem., Int. Ed., 2012, 51, 9433 CrossRef CAS PubMed.
-
(a) H.-M. Zhang, W.-Q. Jia, Z.-Q. Liang and S. Ye, Asian J. Org. Chem., 2014, 3, 462 CrossRef CAS;
(b) Z.-H. Gao, X.-Y. Chen, H.-M. Zhang and S. Ye, Chem. Commun., 2015, 51, 12040 RSC.
- J. Cheng, Z. Huang and Y. R. Chi, Angew. Chem., Int. Ed., 2013, 52, 8592 CrossRef CAS PubMed.
- J. Xu, Z. Jin and Y. R. Chi, Org. Lett., 2013, 15, 5028 CrossRef CAS PubMed.
- B. S. Li, Y. Wang, Z. Jin, P. Zheng, R. Ganguly and Y. R. Chi, Nat. Commun., 2015, 6, 6207 CrossRef CAS PubMed.
- Y. Xiao, J. Wang, W. Xia, S. Shu, S. Jiao, Y. Zhou and H. Liu, Org. Lett., 2015, 17, 3850 CrossRef CAS PubMed.
- X.-Y. Chen, Z.-H. Gao, C.-Y. Song, C.-L. Zhang, Z.-X. Wang and S. Ye, Angew. Chem., Int. Ed., 2014, 53, 11611 CrossRef CAS PubMed.
-
(a) A. Lee, A. Younai, C. K. Price, J. Izquierdo, R. K. Mishra and K. A. Scheidt, J. Am. Chem. Soc., 2014, 136, 10589 CrossRef CAS PubMed;
(b) J.-T. Cheng, X.-Y. Chen and S. Ye, Org. Biomol. Chem., 2015, 13, 1313 RSC.
-
(a) Y. Xie, C. Yu, T. Li, S. Tu and C. Yao, Chem. – Eur. J., 2015, 21, 5355 CrossRef CAS PubMed;
(b) Z. Jin, K. Jiang, Z. Fu, J. Torres, P. Zheng, S. Yang, B.-A. Song and Y. R. Chi, Chem. – Eur. J., 2015, 21, 9360 CrossRef CAS PubMed.
-
(a) C. Marti and E. M. Carreira, Eur. J. Org. Chem., 2003, 2209 CrossRef CAS;
(b) R. M. Williams and R. J. Cox, Acc. Chem. Res., 2003, 36, 127 CrossRef CAS PubMed;
(c) C. V. Galliford and K. A. Scheidt, Angew. Chem., Int. Ed., 2007, 46, 8748 CrossRef CAS PubMed;
(d) N. R. Ball-Jones, J. J. Badillo and A. K. Franz, Org. Biomol. Chem., 2012, 10, 5165 RSC;
(e) R. Dalpozzo, G. Bartoli and G. Bencivenni, Chem. Soc. Rev., 2012, 41, 7247 RSC.
-
(a) B. Cardinal-David, D. E. A. Raup and K. A. Scheidt, J. Am. Chem. Soc., 2010, 132, 5345 CrossRef CAS PubMed;
(b) D. E. A. Raup, B. Cardinal-David, D. Holte and K. A. Scheidt, Nat. Chem., 2010, 2, 766 CrossRef CAS PubMed;
(c) J. Dugal-Tessier, E. A. O'Bryan, T. B. H. Schroeder, D. T. Cohen and K. A. Scheidt, Angew. Chem., Int. Ed., 2012, 51, 4963 CrossRef CAS PubMed;
(d) A. Lee and K. A. Scheidt, Angew. Chem., Int. Ed., 2014, 53, 7594 CrossRef CAS PubMed;
(e) Z. Q. Rong, M. Q. Jia and S. L. You, Org. Lett., 2011, 13, 4080 CrossRef CAS PubMed;
(f) J. Mo, X. Chen and Y. R. Chi, J. Am. Chem. Soc., 2012, 134, 8810 CrossRef CAS PubMed;
(g) J. Mo, L. Shen and Y. R. Chi, Angew. Chem., Int. Ed., 2013, 52, 8588 CrossRef CAS PubMed;
(h) Z. X. Xiao, C. X. Yu, T. J. Li, X. S. Wang and C. S. Yao, Org. Lett., 2014, 16, 3632 CrossRef CAS PubMed;
(i) J.-T. Cheng, X.-Y. Chen, Z.-H. Gao and S. Ye, Eur. J. Org. Chem., 2015, 1047 CrossRef CAS;
(j) Z. Wu, F. Li and J. Wang, Angew. Chem., Int. Ed., 2015, 54, 1629 CrossRef CAS PubMed.
Footnotes |
† Electronic supplementary information (ESI) available: Experimental details, and 1H and 13C NMR spectra. See DOI: 10.1039/c5qo00301f |
‡ These authors contributed equally to this work. |
|
This journal is © the Partner Organisations 2016 |
Click here to see how this site uses Cookies. View our privacy policy here.