DOI:
10.1039/C5QI00241A
(Research Article)
Inorg. Chem. Front., 2016,
3, 406-416
3,5-Bis((4′-carboxylbenzyl)oxy)benzoilate-based coordination polymers: their synthesis, structural characterization, and sensing properties†
Received
6th November 2015
, Accepted 26th December 2015
First published on 28th December 2015
Abstract
With the assistance of various organic bases, hydrothermal reactions of transition-metal salts with a kind of tripodal tricarboxylic acid, 3,5-bis((4′-carboxylbenzyl)oxy)benzoic acid (H3(bcbob)), at different pH values were carried out, producing four new bcbob-based 3-D coordination polymers: [Ni2(Hbcbob)2(bimb)2] (bimb = 1,4-bis(imidazol-1-yl-methyl)benzene) 1, [Zn3(bcbob)2(bpe)2] (bpe = 1,2-bis(4-pyridyl)ethene) 2, [H2(bpp)][Mn2(bcbob)2] (bpp = 1,2-bis(4-piperidyl)propane) 3, and [Cd2(OH)(bcbob)(bpe)0.5]·H2O 4. X-ray single-crystal diffraction analysis reveals that (i) in 1, the bcbob molecules link the Ni2+ centers into a 2-D layer with a (6,3) net, and then the bimb molecule serves as the pillar linker; (ii) in 2, the bpe molecule is only used to stabilize the 3-D network. The bcbob molecules link two types of tetrahedral Zn2+ ions into a 3-D network with a pcu topology; and (iii) the 3-D networks of 3 and 4 are both built up from carboxylate-bridged rod-shaped secondary building units (SBUs) by the bcbob molecules (acting as a 3-connected node), and three types of 1-D channels are observed. However, since the rod-shaped SBUs contain different structures, the side walls of the channels exhibit different arrangements. In particular, the side wall in 4 adopts a helical arrangement. The bcbob molecules act as the side wall of the channel. In 3, the H2(bpp)2+ molecules occupy the space of the largest channels, while in 4, the bpe molecules occlude one type of smaller channels by coordinating to the Cd2+ ions on the side wall. The photoluminescence analysis reveals that 2 and 4 can serve as chemosensors to detect the ppm-grade nitrobenzene (250 ppm for 2, 150 ppm for 4).
Introduction
Considerable attention has been paid to the design and synthesis of novel metal-multicarboxylate coordination polymers (CPs), owing to their structural diversity1 and potential applications in some fields.2 For example, (i) due to the stronger ability to form clusters or 1-D chains for the metal ions with carboxylate groups, some metal-multicarboxylate CPs possess stable 3-D networks with porous structures, which have been confirmed to be potential candidates for H2 storage and CO2 capture;3 (ii) due to the electron deficient nature of nitro-aromatics, it can change the transfer path of the excited electron for metal-multicarboxylate CPs, leading to photoluminescence quenching. So ligand-based photoluminescence metal-multicarboxylate CPs can act as sensors to detect trace nitro-explosives;4 and (iii) due to the linkage of carboxylate groups, strong M⋯M interactions may be observed in metal-multicarboxylate CPs, which makes these materials exhibit interesting magnetic properties.5
Ever since a highly porous CP [Cu3(tma)2(H2O)3] (tma = benzene-1,3,5-tricarboxylate) was reported,6 some tripodal tricarboxylic acids have been designed, constructing a series of novel metal CP materials over the last fifteen years.7 However, the semi-rigid 3,5-bis((4′-carboxylbenzyl)oxy)benzoilate (bcbob)-based metal CPs remain less investigated.8Scheme 1 plots the molecular structure of the H3(bcbob) molecule. So-called semi-rigidity means that two benzene rings at the 3- and 5-positions can freely rotate around the –O–CH2– groups. Due to the existence of two non-metallic atoms, the dihedral angle between two neighboring benzene rings can span a wider range from 0° to 90°, compared with those multi-carboxylic molecules without any non-metallic atom (dihedral angle: 0° or a slight rotation) or with only one non-metallic atom (dihedral angle: 90° or a slight rotation). Furthermore, the C6H5–O–CH2–H5C6 moiety may show different shapes: Z or V shape (see Scheme S1†). Hence, the framework structure of the as-synthesized bcbob-extended CP is more unpredictable. In addition, the N,N′-donor organic bases were mixed into the metal–bcbob reactive system, aiming at (i) extending the metal–bcbob framework and (ii) stabilizing the metal–bcbob framework structure. In this article, a series of hydrothermal reactions of transition-metal salts and H3bcbob with the assistance of various N,N′-donor organic bases at different pH values were performed, producing finally four new bcbob-based 3-D coordination polymers: [Ni2(Hbcbob)2(bimb)2] (bimb = 1,4-bis(imidazol-1-yl-methyl)benzene) 1, [Zn3(bcbob)2(bpe)2] (bpe = 1,2-bis(4-pyridyl)ethene) 2, [H2(bpp)][Mn2(bcbob)2] (bpp = 1,2-bis(4-piperidyl)propane) 3, and [Cd2(OH)(bcbob)(bpe)0.5]·H2O 4. In order to estimate the properties of the as-synthesized CP materials, the sensing ability of compounds 2 and 4 on the nitrobenzene (NB) molecule was investigated.
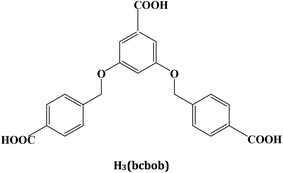 |
| Scheme 1 Molecular structure of H3(bcbob). | |
Experimental
General
All chemicals were of reagent grade quality, obtained from commercial sources without further purification. Elemental analysis (C, H and N) was performed on a Perkin-Elmer 2400LS II elemental analyzer. Infrared (IR) spectrum was recorded on a Perkin-Elmer Spectrum 1 spectrophotometer in the 4000–400 cm−1 region using a powdered sample on a KBr plate. Powder X-ray diffraction (XRD) data were collected on a Rigaku/max-2550 diffractometer with Cu-Kα radiation (λ = 1.5418 Å). Thermogravimetric (TG) behavior was investigated on a Perkin-Elmer TGA-7 instrument with a heating rate of 10 °C min−1 in air. Fluorescence spectra were recorded on a LS 55 florescence/phosphorescence spectrophotometer at room temperature.
Syntheses of coordination polymers
[Ni2(Hbcbob)2(bimb)2] 1.
A mixture of Ni(NO3)2·6H2O (0.2 mmol, 58 mg), H3(bcbob) (0.1 mmol, 42 mg), bimb (0.1 mmol, 24 mg) and water (10 mL) (acidified to pH = 5 by dilute HNO3) was heated to 150 °C in a Teflon-lined stainless steel vessel under autogenous pressure for 4 days, and subsequently cooled to room temperature. The green block crystals of 1 were collected, washed with water, and dried in air. Yield: ca. 25% based on Ni(II). Anal. calcd for C74H60N8O16Ni21: C, 61.95; H, 4.21; N, 7.81. Found: C, 61.69; H, 3.97; N, 7.66%. IR (cm−1): 1647 (m), 1593 (m), 1532 (m), 1423 (s), 1294 (w), 1241 (w), 1161 (s), 1109 (w), 1061 (w), 769 (m), 663 (w).
[Zn3(bcbob)2(bpe)2] 2.
A mixture of Zn(OAc)2·4H2O (0.2 mmol, 44 mg), H3(bcbob) (0.1 mmol, 42 mg), bpp (0.1 mmol, 21 mg) and water (10 mL) (neutralized to pH = 7 by dilute NaOH) was heated to 150 °C in a Teflon-lined stainless steel vessel under autogenous pressure for 4 days, and subsequently cooled to room temperature. The colorless block crystals of 2 were collected, washed with water, and dried in air. Yield: ca. 20% based on Zn(II). Anal. calcd for C70H54N4O16Zn32: C, 59.91; H, 3.88; N, 3.99. Found: C, 59.76; H, 3.68; N, 4.12%. IR (cm−1): 1614 (s), 1568 (s), 1507 (w), 1422 (m), 1343 (m), 1151 (s), 1049 (m), 987 (w), 835 (w), 778 (s), 711 (w), 558 (m).
[H2(bpp)][Mn2(bcbob)2] 3.
A mixture of MnCl2·4H2O (0.16 mmol, 32 mg), H3(bcbob) (0.08 mmol, 34 mg), bpp (0.08 mmol, 17 mg) and water (10 mL) (neutralized to pH = 6 by dilute NaOH) was heated to 150 °C in a Teflon-lined stainless steel vessel under autogenous pressure for 4 days, and subsequently cooled to room temperature. The colorless block crystals of 3 were collected, washed with water, and dried in air. Yield: ca. 20% based on Mn(II). Anal. calcd for C59H60N8O16Ni23: C, 61.95; H, 4.21; N, 7.81. Found: C, 61.69; H, 3.97; N, 7.66%. IR (cm−1): 1591 (s), 1557 (s), 1399 (s), 1373 (s), 1300 (m), 1139 (s), 1041 (m), 854 (w), 821 (w), 775 (m), 756 (w), 708 (w), 629 (w).
[Cd2(OH)(bcbob)(bpe)0.5]·H2O 4.
A mixture of Cd(OAc)2·2H2O (0.2 mmol, 53 mg), H3(bcbob) (0.1 mmol, 42 mg), bpe (0.1 mmol, 21 mg) and water (10 mL) (neutralized to pH = 7 by dilute NaOH) was heated to 150 °C in a Teflon-lined stainless steel vessel under autogenous pressure for 4 days, and subsequently cooled to room temperature. The colorless block crystals of 4 were collected, washed with water, and dried in air. Yield: ca. 18% based on Cd(II). Anal. calcd for C29H23NO10Cd24: C, 45.21; H, 3.01; N, 1.82. Found: C, 45.69; H, 3.08; N, 2.16%. IR (cm−1): 1617 (w), 1593 (w), 1507 (w), 1545 (s), 1407 (s), 1367 (s), 1151 (s), 1048 (m), 1020 (w), 855 (w), 825 (w), 767 (m), 550 (m).
X-ray crystallography
The data were collected with Mo-Kα radiation (λ = 0.71073 Å) on a Rigaku R-AXIS RAPID IP diffractometer for 1 and 3, and on a Siemens SMART CCD diffractometer for 2 and 4. With the SHELXTL program, the structures of all were solved using direct methods.9 The non-hydrogen atoms were assigned anisotropic displacement parameters in the refinement, and the other hydrogen atoms were treated using a riding model. The hydrogen atoms on C30 in 3 and on the hydroxyl group in 4 were not located. The hydrogen atom on the carboxyl group in 1 was not located. The lattice water molecule in 4 was confirmed by the elemental analysis and the TG analysis. This method has been extensively employed in the related reports.10 The structures were then refined on F2 using SHELXL-97.9 CCDC numbers are 1059704–1059706 for 1–3 and 1426333 for 4, respectively. The crystallographic data for 1–4 are summarized in Table 1.
Table 1 Crystal data of 1–4
|
1
|
2
|
3
|
4
|
Formula |
C74H60N8O16Ni2 |
C70H50N4O16Zn3 |
C59H58N2O16Mn2 |
C29H23NO10Cd2 |
M
|
1434.72 |
1399.25 |
1160.95 |
770.30 |
T (K) |
293(2) |
293(2) |
293(2) |
293(2) |
Crystal system |
Triclinic |
Monoclinic |
Monoclinic |
Monoclinic |
Space group |
P![[1 with combining macron]](https://www.rsc.org/images/entities/char_0031_0304.gif) |
C2/c |
C2/c |
C2/c |
a (Å) |
9.1609(18) |
45.925(2) |
15.338(3) |
31.512(3) |
b (Å) |
12.811(3) |
6.5022(3) |
22.100(4) |
7.0593(4) |
c (Å) |
14.754(3) |
21.3486(15) |
16.425(3) |
27.1251(19) |
α (°) |
87.65(3) |
90 |
90 |
90 |
β (°) |
85.10(3) |
105.346(6) |
102.64(3) |
106.230(4) |
γ (°) |
89.56(3) |
90 |
90 |
90 |
V (Å3) |
1723.7(6) |
6147.2(6) |
5432.6(19) |
5793.6(8) |
Z
|
1 |
4 |
4 |
8 |
D
c (g cm−3) |
1.382 |
1.512 |
1.419 |
1.766 |
μ (mm−1) |
0.621 |
1.237 |
0.539 |
1.526 |
Reflections collected |
16 778 |
16 552 |
22 151 |
15 895 |
Unique reflections |
7711 |
5397 |
6199 |
5106 |
R
int
|
0.0242 |
0.0416 |
0.0512 |
0.0360 |
Gof |
1.102 |
1.052 |
1.050 |
1.179 |
R
1, I > 2σ(I) |
0.0409 |
0.0493 |
0.0560 |
0.0407 |
wR2, all data |
0.1176 |
0.1689 |
0.1674 |
0.1331 |
Results and discussion
Synthetic analysis
All of the reactions were carried out under hydrothermal conditions. Reactions of transition-metal salts, H3(bcbob) and organic bases in a molar ratio of 2
:
1
:
1 at different pH levels were performed, creating the title compounds 1–4. During the reactions, the pH value of the reactive mixture plays a key role. On the one hand, it determines the degree of deprotonation reaction for H3(bcbob). At pH ≥ 6, the H3(bcbob) molecule can be completely deprotonated, and exists in the form of bcbob3−, based on the reactions of compounds 2, 3 and 4, while at pH = 5, the H3(bcbob) molecule is partly protonated and exists in the form of H(bcbob)2−, based on the reaction of compound 1. On the other hand, it controls the existing form of the organic base molecule. As observed in compound 3, bpp exists in a diprotonated form at pH = 6, whereas as observed in compounds 2 and 4, bpe exists as a neutral molecule at pH = 7. But the bimb molecule is difficult to protonate in a weakly acidic environment such as pH = 5, as was found when preparing compound 1.
Structural description of 1
Compound 1 is a 3-D Ni2+ coordination polymer with a mixed ligand of bcbob and bimb. The asymmetric unit of compound 1 is found to be composed of one Ni2+ ion (Ni1), one bcbob molecule and one bimb molecule. The organic acidic molecule should exist in the form of H(bcbob)2− in order to balance the systematic charge. The 1-position carboxyl group should be deprotonated, which is revealed by the two equivalent C–O distances, while for the other two carboxylate groups, the two C–O distances for each are different, suggesting that this H+ ion is related to these two carboxylate groups. The bcbob molecule exhibits a triple-bridged coordination mode. As shown in Scheme 2, the 1-position carboxylate group and the carboxylate group on the 3-position benzyloxy group monodentately bond respectively to one Ni2+ center, while the carboxylate group on the 5-position benzyloxyl group chelates to one Ni2+ center. As shown in Fig. 1b, the η1:η0:η1:η0:η1:η1:μ3-mode H(bcbob)2− molecules link the Ni2+ centers into a 2-D layer network. Based on the topological viewpoint, both the Ni2+ center and the bcbob molecule can be regarded as a 3-connected node, so this 2-D layer network possesses a simple (6,3) topology. The bimb molecule serves as the pillar linker, extending the 2-D Ni(Hbcbob) layers into a 3-D network, as shown in Fig. 1a. Synchronously, the 1-D channels form in the 3-D network of compound 1. However, the 3-fold interpenetration is observed in the packing structure of compound 1, occluding these channels. The Ni1 center in compound 1 is involved in an octahedral site with four carboxylate O atoms (O2, O4b, O5a, O6a; Ni1–O = 2.0621(16)–2.1466(16) Å) occupying the equatorial plane, and two bimb N atoms (N1, N3; Ni1–N = 2.061(2)–2.062(2) Å) occupying the axial positions (see Fig. S1†).
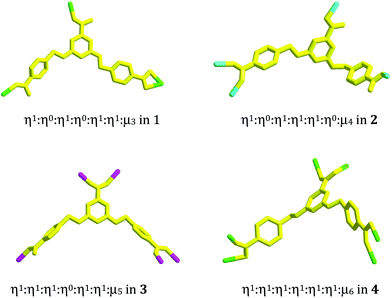 |
| Scheme 2 Coordination modes of bcbob in 1–4. | |
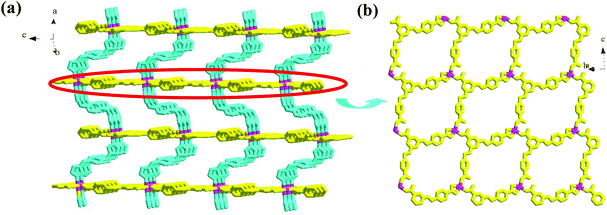 |
| Fig. 1 3-D network (a) and 2-D Ni–H(bcbob)2− layer (b) in 1. | |
Structural description of 2
Compound 2 is a 3-D Zn2+ coordination polymer with a mixed ligand of bcbob and bpe. The asymmetric unit of compound 2 is found to be composed of two types of Zn2+ ions (Zn1, Zn2; occupancy ratio: 1 for Zn1, 0.5 for Zn2), one bcbob molecule and one bpe molecule. Zn1 and Zn2 are both involved in a tetrahedral site, but the detailed coordination environments are different (see Fig. S2†). Zn1 is coordinated with three carboxylate O atoms (O2a, O3b, O4) and one bpe N atom (N1), whereas Zn2 is completed by two carboxylate O atoms (O6d, O6e) and two bpe N atoms (N2, N2f). As shown in Scheme 2, the bcbob molecule adopts a tetra-bridged coordination mode. Two carboxylate groups interact respectively with one Zn2+ ion, whereas the third carboxylate group bridges bidentately to two Zn2+ ions. In compound 2, the bpe molecule plays two roles. One is to complete the tetrahedral coordination of two Zn2+ centers, and the other is to stabilize the 3-D network of compound 2. The bcbob molecules link two types of Zn2+ ions to form a 3-D network of compound 2, as shown in Fig. 2a. The bcbob molecules first utilize two carboxylate groups to serve as donors, linking the Zn1 ions into a 2-D layer network (see Fig. 2b). This 2-D layer is based on a kind of carboxylate-bridged dimer (see Fig. 2c), which is composed of two Zn1 centers and two carboxylate groups on the 5-position benzyloxyl groups. Via the interactions between the Zn1 ion and the 1-position carboxylate O atom (O2a), each dimer interacts with four adjacent dimers, forming finally this 2-D layer network. The other carboxylbenzyloxy groups protrude from the layer. Via the interactions between Zn2 and O6d/O6e, the Zn2 ions act as bridges, propagating the 2-D layer into a 3-D network of compound 2. The bpe molecule acts as the second connector to link further Zn1 and Zn2 together, stabilizing the 3-D network of compound 2 (see Fig. 2d). The Zn–O and Zn–N ranges are 1.945(4)–1.966(3) Å and 2.024(4)–2.059(4) Å, respectively.
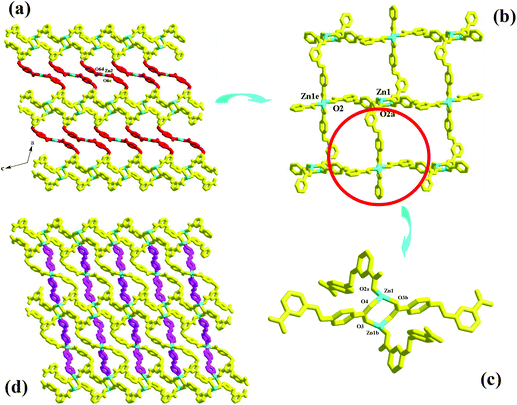 |
| Fig. 2 3-D Zn-bcbob network (a), 2-D (4,4) net (b), dimer (c) and 3-D Zn-bcbob-bpe network (d) in 2 ((a) x, −y + 4, z + 1/2; (b) −x + 1/2, −y + 5/2, −z + 2; (c) x, −y + 2, z + 1/2; (d) −x, −y + 2, −z + 1; (e) x, −y + 4, z − 1/2). | |
Structural description of 3
Compound 3 is a bcbob-extended 3-D Mn2+ coordination polymer with the occluded H2(bpp)2+ cations. The asymmetric unit of compound 3 is found to be composed of one Mn2+ ion (Mn1), one bcbob molecule and half a H2(bpp)2+ molecule. As shown in Fig. S3,† Mn1 with a trigonal bipyramidal geometry is coordinated with five carboxylate O atoms (O1, O3d, O4a, O5b, O6c; Mn1–O = 2.084(2)–2.143(2) Å). The bcbob molecule is involved in a penta-bridged coordination mode: five O atoms monodentately bond to one Mn2+ center, respectively (see Scheme 2). Templated by H2(bpp)2+, the penta-bridged bcbob molecules link the 5-coordinated Mn2+ centers into a 3-D network structure, as shown in Fig. 3a. The 3-D network is based on the rod-shaped secondary building unit (SBU), which can be described as an alternate arrangement of two types of carboxylate-bridged 8-membered dimers (dimer I, dimer II; see Fig. 3b). The carboxylate groups on 5-position benzyloxy groups doubly bridge two Mn2+ ions to construct dimer I, whereas the 1-position carboxylate groups doubly bridge two Mn2+ ions to construct dimer II. The two types of dimers show different arrangements: boat configuration for dimer I and chair configuration for dimer II. The Mn⋯Mn separations are 4.973 Å and 3.702 Å, respectively. The bcbob molecule serves as a 3-connected node, extending the rod-shaped SBUs into a 3-D network of compound 3, where the 1-D channels with the larger rhomboid window are observed (see Fig. 3c). As shown in Fig. 3d, the bcbob molecules with the carboxylate groups on 3,5-position benzyloxy groups doubly bridge two rod-shaped SBUs to form this 1-D channel with the window size of 10 × 9 Å2. The bcbob molecules act as the side wall of the channel. The arrangements of two side walls are the same (see Fig. 3d).
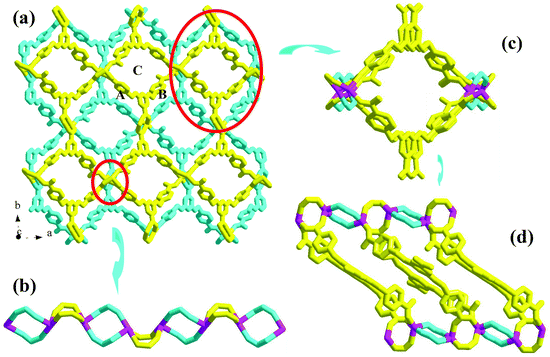 |
| Fig. 3 3-D network (a), rod-shaped SBU (b), and 1-D channel (c, d) in 3. | |
Structural description of 4
Compound 4 is a 3-D Cd2+ coordination polymer with a mixed ligand of bcbob and bpe. The asymmetric unit of compound 4 is found to be composed of two types of Cd2+ ions (Cd1, Cd2), one bcbob molecule, half a bpe molecule, and one OH− group. The triple-bridged O atom is defined as the OH− group in order to balance the systematic charge. The two types of Cd2+ ions show different geometries: Cd1 is in an octahedral site, surrounded by three carboxylate O atoms (O2, O3c, O6b), one bpe N atom (N1), and two OH− groups (OH1, OH1a), whereas Cd2 is in a tetrahedral site, completed by three carboxylate O atoms (O1, O4d, O5e) and one OH− group (OH1) (see Fig. S4†). The bcbob molecule adopts a hexa-bridged coordination mode: each carboxylate group bidentately bridges two Cd2+ centers (see Scheme 2). The OH− group adopts a trigonal pyramidal geometry with the Cd–OH1–Cd angles of 101.19(14)–107.25(14)° and a distance of 0.95 Å from the apical OH− group to the plane composed of three Cd(II) ions. As shown in Fig. 4a, compound 4 seems to have a similar 3-D network to that of compound 3, namely the bcbob molecules as a 3-connected node link the rod-shaped SBUs into a 3-D network with three types of 1-D channels (channel A, channel B, and channel C). They are indeed different. In compound 4, the rod-shaped SBU Cd2(OH)(COO)6 is based on a kind of tetranuclear cluster. As shown in Fig. 4b, two OH− groups link two Cd1 ions and two Cd2 ions to form this tetranuclear cluster. The centric Cd2O2 ring is planar, and the Cd1⋯Cd1a separation is 3.605 Å. Each of the two neighboring Cd2+ ions are further bridged by carboxylate I and carboxylate II for the bcbob molecule, stabilizing this cluster structure. Then the carboxylate III groups doubly bridge the adjacent tetranuclear clusters into a chained SBU of compound 4, extending along the b-axial direction. Since the SBU shows differences, the bcbob molecule also adopts a different arrangement in both compounds (see Fig. 4c). The arrangements on the two side walls in compound 4 are different, and a helical chain is found, as shown in Fig. 4d. As shown in Fig. S5,† the 3-D network of compound 3 can also be described as an alternate arrangement of two types of layers via sharing the Mn2+ centers. A translation of the layer I produces layer II. However, due to the existence of the helical chain in compound 4, it is difficult to find a 2-D layer in the 3-D network of compound 4. In compound 3, the H2(bpp)2+ molecules occupy the space of the largest channel C. Channel A has the same window as that of channel B, whereas in compound 4, the bpe molecules occlude the space of channel B by coordinating to the Cd2+ centers on the side walls. Although the window of channel C turns small, channel C can be activated, and the lattice water molecule occupies its space. The Cd–O range is 2.166(8)–2.414(7) Å, and the Cd–N separation is Cd1⋯Cd2 = 3.3245(10) Å.
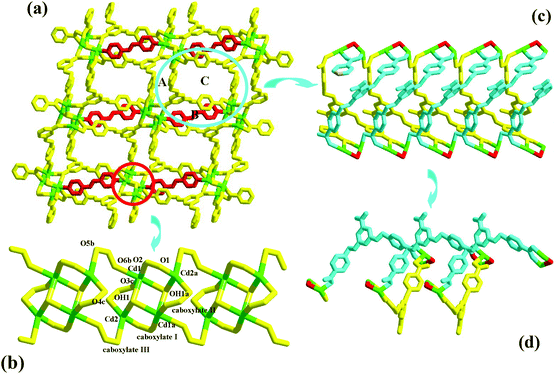 |
| Fig. 4 3-D network (a), rod-shaped SBU (b), 1-D channel (c) and helical chain (d) in 4 ((a) −x − 1/2, −y + 1/2, −z − 1; (b) −x, y − 1, −z − 1/2; (c) x, −y + 2, z − 1/2). | |
Structural discussion
The four title compounds are all new, different from the reported bcbob-based CPs.8 All possess 3-D network structures. The bcbob molecule shows its special semi-rigidity: (i) in compounds 1–4, the dihedral angles of two neighboring benzene rings span a rather wider range from 13.5° observed in compound 1 to 87.7° observed in compound 2. Note that in compound 1, two adjacent benzene rings array in a nearly parallel form and (ii) the C6H5–O–CH2–H5C6 moiety for bcbob exhibits two shapes. Generally, it adopts a Z-shaped arrangement, namely two neighboring benzene rings are distributed on both sides of the CH2–O line. In compound 2, the V-shaped C6H5–O–CH2–H5C6 moiety is observed. As found in compounds 3 and 4, the porous size of the 3-D M2+-bcbob network can be adjusted by the mixed organic base molecule, which should also be related to the diverse configuration of the C6H5–O–CH2–H5C6 moiety. The bcbob molecules can link the metal ions into a 3-D network with the channels. The channel has a larger window. Such a 3-D network is unstable. The main role of the introduced organic base molecule is to stabilize this unstable 3-D network: (i) stabilizing the 3-D network by way of decreasing the window size of the channel, namely acting as a so-called pillar to further support the channel, as observed in compounds 2 and 4; (ii) stabilizing the 3-D network by way of acting as the guest molecule, as observed in compound 3. Once the bcbob molecules only link the metal ions into an oligomer, the introduced organic base molecule can serve as the pillar linker, extending the metal–bcbob oligomers into a 3-D network, as observed in compound 1. Due to the existence of the channel with the larger window, this 3-D network is unstable, either. So a 3-fold interpenetration occurs in compound 1, in order to form a stable network. Obviously, the most important role of the incorporated organic base molecule is to directly influence the interaction between the metal ion and the bcbob molecule.
Characterization
Fig. S6† presents the experimental and simulated powder XRD patterns of 1–4. The experimental powder XRD pattern for each compound is in accord with the simulated one generated on the basis of structural data, confirming that the as-synthesized product is in pure phase.
The TG behaviors of compounds 1–4 were investigated. Fig. S7† shows the temperature vs. weight-loss curves. For the TG curve of compound 4, a minor weight loss of ca. 2.2% is observed in the temperature range of 30–300 °C, corresponding to the removal of one water molecule (calcd: 2.3%). This means that in an asymmetric unit of compound 4, there is one lattice water molecule (see Fig. S8† for a better understanding). The C, H and N analysis also supports this conclusion. For the TG curves of compounds 1–3, no weight loss is found before 300 °C, indicating that there are no neutral guest molecules in compounds 1–3. Based on the TG curves of 1–4, we also know that (i) compounds 1–4 possess a better thermal stability, because the temperatures for the onset of the collapse of the host frameworks are higher: 350 °C for 1, 400 °C for 2, 350 °C for 3, 370 °C for 4 and (ii) the final residues for all have been proved to be the metal (di)oxides: NiO for 1 (calcd: 10.4%; found: ca. 10.1%), ZnO for 2 (calcd: 17.4%; found: ca. 18.6%), MnO2 for 3 (calcd: 14.9%; found: ca. 13.9%), and CdO for 4 (calcd: 33.3%; found: ca. 33.2%), respectively. Fig. S9† plots the IR spectra of compounds 1–4.
Sensing properties
As expected, the Zn(II) compound 2 and the Cd(II) compound 4 possess photoluminescence properties (see Fig. 5). Upon excitation (λex = 363 nm for 2; λex = 350 nm for 4), both emit blue light, and the peaks are centered at 427 nm for 2, and 430 nm for 4, respectively. The emissions of both should be assigned to the bcbob-centered electronic excitation (π* → π), since the organic H3(bcbob) molecule exhibits a similar emission (λem = 425 nm) when excited at 365 nm (see Fig. S10†).
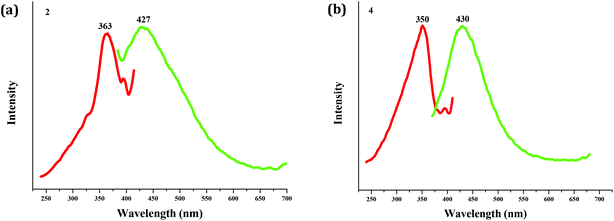 |
| Fig. 5 Photoluminescence spectra of 2 (a) and 4 (b) in the solid state. | |
In order to reveal the sensing ability of the as-synthesized CP materials, the photoluminescence behaviors of the suspension-state 2 and 4 dispersed in different organic solvents were investigated. The selected organic solvents are CH2Cl2, DMSO (dimethylsulfoxide), DMF (N,N′-dimethyl formamide), MeOH, EtOH, CH3CN, acetone, DMA (N,N′-dimethylacetamide), NMP (N-methylpyrrolidone), toluene, acetonitrile and NB. Before measurements, a series of stable emulsions were prepared as follows: a finely ground powder sample (5 mg) was immersed in an organic solvent (5.00 mL), treated by ultrasonication for 30 min, and then aged for 3 days. As shown in Fig. 6, dispersed in the other organic solvents, the suspensions of compounds 2 and 4 all emit light. They exhibit a similar emission to that of the solid-state sample. Only a minor red- or blue-shift is observed, which might be associated with the solvated effect.11 The emission intensities of all of the emulsions exhibit differences. For compound 2, CH2Cl2, DMF and DMSO have been proved to be the strongest enhancers, whereas dispersed in DMF, the suspension of 4 shows the maximum. However, the suspensions of compounds 2 and 4 exhibit a special emission behavior when dispersed in NB (nitrobenzene). The emissions are completely quenched by NB, which suggests that compounds 2 and 4 can both serve as a sensor to detect the NB molecule. After the measurements, the solid particles are filtered from the suspensions. Fig. S11† shows the XRD patterns of these solid particles. For compound 2, the experimental XRD patterns are in agreement with the simulated ones, suggesting that compound 2 is stable in organic solvents, while for compound 4, in some organic solvents, it is stable. But in some solvents, 4 is unstable, and the XRD patterns show a small difference.
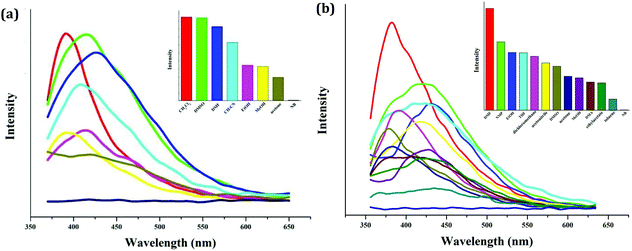 |
| Fig. 6 Photoluminescence behaviors of the suspension-state 2 (a) and 4 (b) dispersed in different organic solvents (λex = 340 nm for 2, λex = 330 nm for 4). | |
In order to investigate the quenching efficiency of the NB molecule, the emission behaviors of the suspensions of 2 and 4 with gradually increasing NB concentrations were measured. DMF was selected to act as the dispersible solvent for both. The selection of the dispersible solvent is based on two rules: (i) dispersed in this solvent, the suspension should have a stronger emission and (ii) dispersed in this solvent, the sample should be stable. As shown in Fig. 7, for both, the emission intensity of the suspensions gradually decrease with the increase of the NB concentration. When 250 ppm NB is added, the emission of compound 2 is thoroughly quenched, while with the addition of 150 ppm NB, the emission intensity of compound 4 decreases to zero. This implies that compound 2 can sense 250 ppm NB, whereas compound 4 can detect 150 ppm NB. DMSO was also selected to displace DMF to serve as the dispersible solvent of 2. But dispersed in DMF, compound 2 only detects NB with the concentration of 350 ppm. This suggests that the dispersible solvent affects the quenching efficiency.
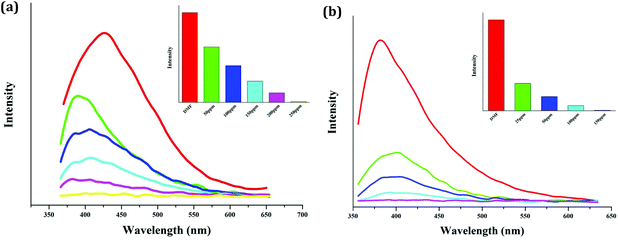 |
| Fig. 7 Photoluminescence behaviors of the suspension-state 2 (a) and 4 (b) with gradually increasing NB concentrations (dispersible solvent: DMF for both; λex = 340 nm for 2, λex = 330 nm for 4). | |
In order to investigate the origin of photoluminescence from the suspensions, solid particles in the suspensions (2/4 + dispersible solvent) are removed, and the emission behaviors of the mother solutions are measured. As shown in Fig. S12,† even though the mother solutions also possess photoluminescence emissions, the intensity is by far weaker than that of the suspensions. This implies that the photoluminescence from the suspensions is mainly derived from the as-synthesized CP.
The bcbob molecule is an electron-rich aromatic ligand (potential electron donor), while the NB molecule possesses the electron deficient nature (potential electron acceptor). The good dispersible nature of the as-synthesized CP particles in the organic solvent makes the introduced NB molecule access easily these particles and become closely adsorbed on the surface of these particles. Due to the close contact, the excited-state electron transfer path of the compound is changed. The excited-state electron would undergo a transfer to the high electron-withdrawing –NO2 group in the NB molecule, instead of returning to the ground-state energy level. So quenching is observed. Based on the above statements, the following three factors may play a key role in determining the sensing ability of the CP material on NB: (i) the size of the solid particle: smaller particles possess larger surface areas, and are easily dispersed evenly in the organic solvent; (ii) the good dispersible nature of these solid particles in the organic solvent: the larger surface area as well as the better dispersant for solid particles is helpful in the attachment of the mixed trace NB molecule; and (iii) the larger π-conjugated structure of the organic ligand in the CP framework: the molecule with the larger π-conjugated structure will give a quick photoluminescence response when encountering NB.12
Conclusion
In summary, we employed a tripodal tricarboxylic acid, namely H3(bcbob), to self-assemble four new 3-D coordination polymers with the assistance of various organic base molecules. Note that the bcbob-based coordination polymers remain less investigated. Synthetically, the pH level of the reactive system is confirmed to be a crucial factor in controlling the crystal growth. It directly affects the deprotonation reaction of the H3(bcbob) molecule. Meanwhile, it controls the existing form of the mixed organic based molecule, a protonated form or a non-protonated form. X-ray single-crystal analysis reveals that the bcbob molecules can generally link the metal centers into a 3-D network. The role of the incorporated organic base molecule is mainly to stabilize the 3-D metal–bcbob network in diverse ways: (i) acting as a guest molecule and (ii) acting as a pillar, supporting the channel. If the bcboc molecules only link the metal centers into an oligomer, the mixed organic base molecule serves as the second linker, extending the oligomers into a 3-D network. At this time, the interpenetration may occur, in order to form a 3-D stable network. A series of photoluminescence analyses of compounds 2 and 4 in the suspension state indicate that (i) compounds 2 and 4 can serve as the sensors to detect the ppm-grade NB; (ii) dispersed in the different solvent, the sensing ability for the compound may exhibit a small difference; (iii) the photoluminescence quenching may originate from three factors: the electron-rich nature of the metal-multicarboxylate CP; the electron-withdrawing nature of NB; the good dispersible nature of the metal-multicarboxylate CP particles in the organic solvent; and (iv) the additional NB molecule alters the excited-state electron transfer path, resulting in photoluminescence quenching.
Acknowledgements
This research was supported by the National Natural Science Foundation of China (No. 21271083).
References
-
(a) H. Zhou and S. Kitagawa, Chem. Soc. Rev., 2014, 43, 5415–5418 RSC;
(b) M. Eddaoudi, D. F. Eubank, K. Adil and V. Guillerm, Chem. Soc. Rev., 2015, 44, 228–249 RSC;
(c) C. K. Brozek and M. Dincă, Chem. Soc. Rev., 2014, 43, 5456–5467 RSC;
(d) Q. Zhu and Q. Xu, Chem. Soc. Rev., 2014, 43, 5468–5512 RSC;
(e) W. Lu, Z. Wei, Z. Gu, T. Liu, J. Park, J. Park, J. Tian, M. Zhang, Q. Zhang, T. Gentle III, M. Bosch and H. Zhou, Chem. Soc. Rev., 2014, 43, 5561–5593 RSC;
(f) S. Furukawa, J. Reboul, S. Diring, K. Sunida and S. Kitagawa, Chem. Soc. Rev., 2014, 43, 5700–5734 RSC;
(g) A. Schneemann, V. Bon, I. Schwedler, I. Senkovska, S. Kaskel and R. A. Fischer, Chem. Soc. Rev., 2014, 43, 6062–6096 RSC;
(h) D. Prochowicz, K. Sokolowski and J. Lewiński, Coord. Chem. Rev., 2014, 270, 112–126 CrossRef;
(i) Y. N. Wang, Q. F. Yang, G. H. Li, P. Zhang, J. H. Yu and J. Q. Xu, Dalton Trans., 2014, 43, 11646–11657 RSC;
(j) Y. N. Wang, F. Q. Bai, J. H. Yu and J. Q. Xu, Dalton Trans., 2013, 42, 16547–16555 RSC;
(k) B. Mu, F. Li, Y. Huang and K. S. Walton, J. Mater. Chem., 2012, 22, 10172–10178 RSC;
(l) T. H. Park, A. J. Hickman, K. Koh, S. Martin, A. G. Wong-Foy, M. S. Sanford and A. J. Matzger, J. Am. Chem. Soc., 2011, 133, 20138–22041 CrossRef CAS PubMed;
(m) P. Wu, C. He, J. Wang, X. Peng, X. Li, Y. An and C. Duan, J. Am. Chem. Soc., 2012, 134, 14991–14999 CrossRef CAS PubMed;
(n) L. C. Wang, J. Sun, Z. T. Huang and Q. Y. Zheng, Cryst. Growth Des., 2013, 13, 1–5 CrossRef CAS;
(o) P. V. Dau, K. K. Tanabe and S. M. Cohen, Chem. Commun., 2012, 48, 9370–9372 RSC;
(p) D. Kim, X. Liu and M. S. Lah, Inorg. Chem. Front., 2015, 2, 336–360 RSC;
(q) B. Tzeng, T. Chang, M. Tsai, Y. Lin, S. Lee and H. Sheu, ChemPlusChem, 2015, 80, 878–885 CrossRef CAS;
(r) B. Tzeng, C. Yeh and T. Chang, ChemPlusChem, 2014, 79, 387–393 CrossRef CAS.
-
(a) A. Dhakshinamoorthy, A. M. Asiri and H. Garcia, Chem. Soc. Rev., 2015, 44, 1922–1947 RSC;
(b) D. Bradshaw, S. E. Hankari and L. L. Spagnolo, Chem. Soc. Rev., 2014, 43, 5431–5443 RSC;
(c) A. Dhakshinamoorthy and H. Garcia, Chem. Soc. Rev., 2014, 43, 5750–5765 RSC;
(d) T. Zhang and W. Lin, Chem. Soc. Rev., 2014, 43, 5982–5993 RSC;
(e) A. Schwarzer, T. Saplinova and E. Kroke, Coord. Chem. Rev., 2013, 257, 2032–2062 CrossRef CAS;
(f) Y. Zhou and B. Yan, Inorg. Chem., 2014, 53, 3456–3463 CrossRef CAS PubMed;
(g) J. Heine and K. M. Buschbaum, Chem. Soc. Rev., 2013, 42, 9232–9242 RSC;
(h) Y. N. Wang, J. H. Yu and J. Q. Xu, Inorg. Chem. Front., 2014, 1, 673–681 RSC;
(i) Y. N. Wang, G. H. Li, F. Q. Bai, J. H. Yu and J. Q. Xu, Dalton Trans., 2014, 43, 15617–15627 RSC;
(j) Y. Lu and B. Yan, Chem. Commun., 2014, 50, 15443–15446 RSC;
(k) L. V. Mever, F. Schönfeld and K. M. Buschbaum, Chem. Commun., 2014, 50, 8093–8108 RSC;
(l) R. F. Mendes and F. A. A. Paz, Inorg. Chem. Front., 2015, 2, 495–509 RSC;
(m) P. Bose, L. Bai, R. Ganguly, R. Zou and Y. Zhao, ChemPlusChem, 2015, 80, 1259–1266 CrossRef CAS;
(n) L. Wang, Y. Li, F. Yang, Q. Liu, J. Ma and Y. Dong, Inorg. Chem., 2014, 53, 9087–9084 CrossRef CAS PubMed.
-
(a) E. Barea, C. Montoro and J. A. R. Navarro, Chem. Soc. Rev., 2014, 43, 5419–5430 RSC;
(b) D. Bradshaw, S. El-Hankari and L. Lupica-Spagnolo, Chem. Soc. Rev., 2014, 43, 5431–5443 RSC;
(c) J. Canivet, A. Fateeva, Y. Guo, B. Coasne and D. Farrusseng, Chem. Soc. Rev., 2014, 43, 5594–5617 RSC;
(d) B. V. Voorde, B. Bueken, J. Denayer and D. D. Vos, Chem. Soc. Rev., 2014, 43, 5766–5788 RSC;
(e) X. Wang, J. Luan, H. Lin, Q. Lu, M. Le, G. Liu and J. Shao, ChemPlusChem, 2014, 79, 1691–1702 CAS;
(f) B. Liu, Y. H. Jiang, Z. S. Li, L. Hou and B. Liu, Inorg. Chem. Front., 2015, 2, 550–557 RSC;
(g) X. L. Qi, J. W. Ye, R. B. Liu, P. Q. Liao, S. Y. Liu, C. T. He, J. P. Zhang and X. M. Chen, Inorg. Chem. Front., 2015, 2, 136–140 RSC;
(h) X. Duan, J. Yu, J. Cai, Y. He, C. Wu, W. Zhou, T. Yildirim, Z. Zhang, S. Xiang, M. O'Keeffe, B. Chen and G. Qian, Chem. Commun., 2013, 49, 2043–2045 RSC;
(i) Y. Xiong, Y. Fan, R. Yang, S. Chen, M. Pan, J. Jiang and C. Su, Chem. Commun., 2014, 50, 14631–14634 RSC;
(j) Z. Zhu, Y. Bai, L. Zhang, D. Sun, J. Fang and S. Zhu, Chem. Commun., 2014, 50, 14674–14677 RSC;
(k) Z. Niu, S. Fang, J. Ma, X. Zhang and P. Cheng, Chem. Commun., 2014, 50, 7797–7799 RSC;
(l) R. Wang, Q. Meng, L. Zhang, H. Wang, F. Dai, W. Guo, L. Zhao and D. Sun, Chem. Commun., 2014, 50, 4911–4914 RSC;
(m) Q. Yan, Y. Lin, C. Kong and L. Chen, Chem. Commun., 2013, 49, 6873–6875 RSC;
(n) Z. Zhang, Y. Zhao, Q. Gong, Z. Li and J. Li, Chem. Commun., 2013, 49, 653–661 RSC;
(o) C. H. Lee, J. Y. Wu, G. H. Lee, S. M. Peng, J. C. Jiang and K. L. Lu, Inorg. Chem. Front., 2015, 2, 477–484 RSC.
-
(a) Z. Hu, B. J. Deibert and J. Li, Chem. Soc. Rev., 2014, 43, 5815–5840 RSC;
(b) Y. Cui, B. Chen and G. Qian, Coord. Chem. Rev., 2014, 273, 76–86 CrossRef;
(c) X. Zhang, W. Wang, Z. Hu, G. Wang and K. Uvdal, Coord. Chem. Rev., 2015, 284, 206–235 CrossRef CAS;
(d) L. Qin, M. Zheng, Z. Guo, H. Zheng and Y. Xu, Chem. Commun., 2015, 51, 2447–2449 RSC;
(e) W. Shi, A. Cui and H. Kou, ChemPlusChem, 2014, 79, 310–317 CrossRef CAS;
(f) J. Hao and B. Yan, J. Mater. Chem. A, 2014, 2, 18018–18025 RSC;
(g) Y. Li, L. Wang, B. Zhou, Q. Chen and X. Bu, J. Mater. Chem. A, 2013, 1, 495–499 RSC;
(h) Z. Xiang, C. Fang, S. Leng and D. Cao, J. Mater. Chem. A, 2014, 2, 7662–7665 RSC;
(i) L. Liu, X. Chen, J. Qiu and C. Hao, Dalton Trans., 2015, 44, 2897–2906 RSC;
(j) S. Pramanik, Z. Hu, X. Zhang, C. Zheng, S. Kelly and J. Li, Chem. – Eur. J., 2013, 19, 15964–15971 CrossRef CAS PubMed;
(k) D. Banerjee, Z. Hu and J. Li, Dalton Trans., 2014, 43, 10668–10685 RSC;
(l) Z. Hu, B. J. Deibert and J. Li, Chem. Soc. Rev., 2014, 43, 5815–5840 RSC;
(m) Y. Rachuri, B. Parmar, K. K. Bisht and E. Suresh, Inorg. Chem. Front., 2015, 2, 228–236 RSC;
(n) X. Q. Zhao, P. Cui, B. Zhao, W. Shi and P. Cheng, Dalton Trans., 2011, 40, 805–819 RSC;
(o) Y. L. Hou, H. Xu, R. R. Cheng and B. Zhao, Chem. Commun., 2015, 51, 6769–6772 RSC.
-
(a) E. Coronado and G. M. Espallarqas, Chem. Soc. Rev., 2013, 42, 1525–1539 RSC;
(b) L. Cao, Y. Wei, C. Ji, M. Ma, S. Zang and T. C. W. Mark, Chem. – Asian J., 2014, 9, 3094–3098 CrossRef CAS PubMed;
(c) D. Shao, S. Zhang, X. Zhao and X. Wang, Chem. Commun., 2015, 51, 4360–4363 RSC;
(d) S. I. Vasylevs'kyy, G. A. Senchyk, A. B. Lysenko, E. B. Rusanov, A. N. Chernega, J. Jezierska, H. Krautscheid, K. V. Domasevitch and A. Ozarowski, Inorg. Chem., 2014, 53, 3642–3654 CrossRef PubMed;
(e) P. Manna, B. K. Tripuramallu, S. Bommakanti and S. K. Das, Dalton Trans., 2015, 44, 2852–2864 RSC;
(f) S. Adhikari, A. Banerjee, S. Nandi, M. Fondo, J. Sanmartίn-Matalobos and D. Das, RSC Adv., 2015, 5, 10987–10993 RSC;
(g) S. S. P. Dias, M. V. Kirillova, V. André, J. Ktak and A. M. Kirillov, Inorg. Chem. Front., 2015, 2, 525–537 RSC;
(h) J. Xie, H. Shu, H. Hu, Z. Han, S. Shen, F. Yuan, M. Yang, F. Dong and G. Xue, ChemPlusChem, 2014, 79, 985–994 CrossRef CAS.
- S. S.-Y. Chui, S. M.-F. Lo, J. P. H. Charmant, A. G. Orpen and I. D. Williams, Sciences, 1999, 283, 1148–1150 CrossRef CAS.
-
(a) S. Chen, Q. Zhai, S. Li, Y. Jiang and M. Hu, Inorg. Chem., 2015, 54, 10–12 CrossRef CAS PubMed;
(b) B. Dong, L. Chen, S. Zhang, J. Ge, L. Song, H. Tian, Y. Teng and W. Liu, Dalton Trans., 2015, 44, 1435–1440 RSC;
(c) C. Zhan, S. Ou, C. Zou, M. Zhao and C. Wu, Anal. Chem., 2014, 86, 6648–6653 CrossRef CAS PubMed;
(d) C. Zhan, C. Zou, G. Kong and C. Wu, Cryst. Growth Des., 2013, 13, 1429–1437 CrossRef CAS;
(e) A. Bajpai, P. Chandrasekhar, S. Govardhan, R. Banerjee and J. N. Moorthy, Chem. – Eur. J., 2015, 21, 2759–2765 CrossRef CAS PubMed;
(f) K. Peikert, F. Hoffmann and M. Fröba, CrystEngComm, 2015, 17, 353–360 RSC;
(g) P. Du, Y. Yang, Y. Liu, W. Kan and J. Ma, CrystEngComm, 2013, 15, 6986–7002 RSC;
(h) L. Chen, L. Zhang, S. Li, Y. Qiu, K. Shao, X. Wang and Z. Su, CrystEngComm, 2013, 15, 8214–8221 RSC;
(i) H. Fu, F. Wang and J. Zhang, Dalton Trans., 2014, 43, 4668–4673 RSC;
(j) P. Wu, C. He, J. Wang, X. Peng, X. Li, Y. An and C. Duan, J. Am. Chem. Soc., 2012, 134, 14991–14999 CrossRef CAS PubMed;
(k) W. H. Huang, X. J. Luan, X. Zhou, J. Chen and Y. Y. Wang, CrystEngComm, 2013, 15, 10389–10398 RSC;
(l) Q. Tang, S. Liu, Y. Liu, D. He, J. Miao, X. Wang, Y. Ji and Z. Zheng, Inorg. Chem., 2014, 53, 289–293 CrossRef CAS PubMed;
(m) J. Cui, Y. Li, Z. Guo and H. Zheng, Chem. Commun., 2013, 49, 555–557 RSC;
(n) K. Peikert, F. Hoffmann and M. Fröba, Chem. Commun., 2012, 48, 11196–11198 RSC;
(o) C. Janiak, Chem. Commun., 2013, 49, 6933–6937 RSC;
(p) Y. P. He, Y. X. Tan and J. Zhang, Inorg. Chem., 2012, 51, 11232–11234 CrossRef CAS PubMed;
(q) J. Park, J. R. Li, E. C. Sañudo, D. Yuan and H. C. Zhou, Chem. Commun., 2012, 48, 883–885 RSC;
(r) W. H. Huang, G. P. Yang, J. Chen, X. Chen, C. P. Zhang, Y. Y. Wang and Q. Z. Shi, Cryst. Growth Des., 2013, 13, 66–73 CrossRef CAS;
(s) H. Furukawa, M. A. Miller and O. M. Yaghi, J. Mater. Chem., 2007, 17, 3197–3204 RSC;
(t) Y. P. He, Y. X. Tan and J. Zhang, Inorg. Chem., 2013, 52, 12758–12762 CrossRef CAS PubMed;
(u) F. Gándara, H. Furukawa, S. Lee and O. M. Yaghi, J. Am. Chem. Soc., 2014, 136, 5271–5274 CrossRef PubMed;
(v) H. Furukawa, Y. B. Go, N. Ko, Y. Y. Park, F. J. Uribe-Romo, J. Kim, M. O′Keeffe and O. M. Yaghi, Inorg. Chem., 2011, 50, 9147–9152 CrossRef CAS PubMed.
-
(a) R. Patra, H. M. Titi and I. Goldberg, New J. Chem., 2013, 37, 1494–1500 RSC;
(b) X. Zhang, L. Fang, W. Song, W. Fan, L. Sun and X. Zhao, RSC Adv., 2014, 4, 30274–30281 RSC.
- G. M. Sheldrick, Acta Crystallogr., Sect. A: Found. Crystallogr., 2008, 64, 112–122 CrossRef CAS PubMed.
-
(a) J. Meng, Y. Gong, Q. Lin, M. Zhang, P. Zhang, H. Shi and J. Lin, Dalton Trans., 2015, 44, 5407–5416 RSC;
(b) L. Zhang, T. Song, J. Xu, S. Zeng, Y. Wu, Y. Fan and L. Wang, CrystEngComm, 2014, 16, 2440–2451 RSC;
(c) I. Spanopoulos, P. Xydias, C. D. Malliakas and P. N. Trikalitis, Inorg. Chem., 2013, 52, 855–862 CrossRef CAS PubMed;
(d) Q. Zheng, F. Yang, M. Deng, Y. Ling, X. Liu, Z. Chen, Y. Wang, L. Weng and Y. Zhou, Inorg. Chem., 2013, 52, 10368–10374 CrossRef CAS PubMed;
(e) Y. Gai, F. Jiang, L. Chen, Y. Bu, K. Su, S. A. Al-Thabaiti and M. Hong, Inorg. Chem., 2013, 52, 7658–7665 CrossRef CAS PubMed;
(f) F. Yi, S. Dang, W. Yang and Z. Sun, CrystEngComm, 2013, 15, 8320–8329 RSC;
(g) J. J. Henkelis, S. A. Barnett, L. P. Harding and M. J. Hardie, Inorg. Chem., 2012, 51, 10657–10674 CrossRef CAS PubMed;
(h) Y. Hu, H. Ma, B. Zheng, W. Zhang, S. Xiang, L. Zhai, L. Wang, B. Chen, X. Ren and J. Bai, Inorg. Chem., 2012, 51, 7066–7074 CrossRef CAS PubMed;
(i) D. J. Mihalcik, T. Zhang, L. Ma and W. Lin, Inorg. Chem., 2012, 51, 2503–2508 CrossRef CAS PubMed;
(j) X. Li, F. Jiang, M. Wu, L. Chen, J. Qian, K. Zhou, D. Yuan and M. Hong, Inorg. Chem., 2014, 53, 1032–1038 CrossRef CAS PubMed;
(k) Y. Gai, F. Jiang, L. Chen, M. Wu, K. Su, J. Pan, X. Wan and M. Hong, Cryst. Growth Des., 2014, 14, 1010–1017 CrossRef CAS;
(l) Y. Wei, R. Lin, P. Wang, P. Liao, C. He, W. Xue, L. Hou, W. Zhang, J. Zhang and X. Chen, CrystEngComm, 2014, 16, 6325–6330 RSC;
(m) E. Yang, Y. Zhang, Z. Liu and X. Zhao, Inorg. Chem., 2014, 53, 327–335 CrossRef CAS PubMed;
(n) Z. Lin, Y. Huang, T. Liu, X. Li and R. Cao, Inorg. Chem., 2013, 52, 3127–3132 CrossRef CAS PubMed;
(o) A. Santra, I. Senkovska, S. Kaskel and P. K. Bharadwaj, Inorg. Chem., 2013, 52, 7358–7366 CrossRef CAS PubMed.
-
(a) J. Jin, F. Q. Bai, M. J. Jia, Y. N. Wang, H. L. Jia, J. H. Yu and J. Q. Xu, Dalton Trans., 2013, 42, 8771–8780 RSC;
(b) J. Jin, F. Q. Bai, M. J. Jia, J. H. Yu and J. Q. Xu, CrystEngComm, 2013, 15, 5919–5927 RSC.
-
(a) Y. N. Wang, P. Zhang, J. H. Yu and J. Q. Xu, Dalton Trans., 2015, 44, 1655–1663 RSC;
(b) L. Zhou, J. Zhang, Y. Z. Li and H. B. Du, CrystEngComm, 2013, 15, 8989–8997 RSC;
(c) M. Guo and Z. M. Sun, J. Mater. Chem., 2012, 22, 15939–15946 RSC;
(d) H. Zhang, W. Jiang, J. Yang, Y. Y. Liu, S. Song and J. F. Ma, CrystEngComm, 2014, 16, 9939–9946 RSC;
(e) Y. C. He, H. M. Zhang, Y. Y. Liu, Q. Y. Zhai, Q. T. Shen, S. Y. Song and J. F. Ma, Cryst. Growth Des., 2014, 14, 3174–3178 CrossRef CAS;
(f) G. Y. Wang, L. L. Yang, Y. Li, H. Song, W. J. Ruan, Z. Chang and X. H. Bu, Dalton Trans., 2013, 42, 12865–12868 RSC;
(g) H. Wang, W. Yang and Z. M. Sun, Chem. – Asian. J., 2013, 8, 982–989 CrossRef CAS PubMed;
(h) D. Tian, Y. Li, R. Y. Chen, Z. Chang, G. Y. Wang and X. H. Bu, J. Mater. Chem. A, 2014, 2, 1465–1470 RSC;
(i) B. Gole, A. K. Bar and P. S. Mukherjee, Chem. – Eur. J., 2014, 20, 2276–2291 CrossRef CAS PubMed;
(j) H. Xu, F. Liu, Y. Cui, B. Chem and G. Qian, Chem. Commun., 2011, 47, 3153–3155 RSC;
(k) S. R. Zhang, D. Y. Du, J. S. Qin, S. L. Li, W. W. He, Y. Q. Lan and Z. M. Su, Inorg. Chem., 2014, 53, 8105–8113 CrossRef CAS PubMed;
(l) Y. N. Wang, J. H. Yu and J. Q. Xu, ChemPlusChem, 2015, 80, 1732–1740 CrossRef CAS.
Footnote |
† Electronic supplementary information (ESI) available. CCDC 1059704–1059706 for 1–3 and 1426333 for 4. For ESI and crystallographic data in CIF or other electronic format see DOI: 10.1039/c5qi00241a |
|
This journal is © the Partner Organisations 2016 |
Click here to see how this site uses Cookies. View our privacy policy here.