DOI:
10.1039/C6PY00162A
(Paper)
Polym. Chem., 2016,
7, 1979-1986
pH dependent thermoresponsive behavior of acrylamide–acrylonitrile UCST-type copolymers in aqueous media†
Received
28th January 2016
, Accepted 12th February 2016
First published on 12th February 2016
Abstract
There are only a few non-ionic polymers known for showing thermoresponsivity of UCST-type. Copolymers of acrylamide (AAm) and acrylonitrile (AN) represent one of such thermoresponsive polymers. The present work shows pH-dependent UCST-transitions of this copolymer system. Herein, systematic studies were carried out to show hydrolytic stability and retention of UCST of the copolymer under various conditions. Regeneration of lost UCST-type transitions under extreme pH conditions could be achieved by changing pH, and by addition of electrolytes. Reversible addition fragmentation chain transfer (RAFT) was employed as a tool to synthesize copolymers of AAm and AN. Hydrolysis reactions were carried out intentionally under acidic and alkaline conditions, in order to analyze the chemical stability of the synthesized copolymers as well as to introduce carboxylic groups into the polymer structure. The obtained results showed high tolerance of poly(AAm-co-AN) samples under acidic conditions even after long periods of storage (25 days at pH 3) or after use of pH 0 and increased temperatures (40 °C). In the case of base catalyzed hydrolysis, the thermoresponsive behavior was significantly influenced during hydrolysis in buffer solution of pH 9. Loss and regeneration of the phase transition temperature of these copolymers could be achieved by changing the pH from basic to acidic. Meanwhile, hydrolysis at pH 14 at 40 °C influenced the thermoresponsive behavior and the chemical stability of the polymer, increasing the phase transition temperature over 30 °C. Further, we observed that additives, e.g. formamide can act as a sacrificial agent for providing stable UCST-type transitions even under alkaline conditions as well as at high temperatures (85 °C).
1. Introduction
Thermoresponsive polymers are one of the most extensively used “smart” materials.1 These polymers are designed to respond to minor changes of temperature in their environment. Thus, systems with temperature-induced phase separation have been widely studied, since the temperature can be easily changed and controlled. Particularly, water-soluble thermoresponsive materials have found an important position in today's research, mostly in the field of biomedical applications.2–4 Two types of thermoresponsive behaviours are established: polymers with upper critical solution temperature (UCST) in water are the type that phase separate from solution upon cooling below the UCST. These polymers have been growing in popularity over the last few years. Nevertheless there is a vast scope to be covered in order to reach its analogous material with lower critical solution temperature (LCST), which represents the second type of thermoresponsive polymer, the phase separation occurs upon heating, above the LCST. The most known applications, e.g. bioseparation, drug delivery, sensors, membranes, etc.,5–8 have been achieved with poly(N-isopropyl acrylamide) (PNIPAAm) with phase separation of about 32 °C. Nevertheless, non-ionic UCST systems have been progressing over the last few years not only with poly(N-acryloylglycinamide) (PNAGA),9–11 but also with copolymers, e.g. AAm with additional contents of hydrophobic AN or styrene (St) units.12,13 Recently, we showed that free radical polymerization of AAm and AN with benzophenone acrylamide as a cross-linker could be used for the preparation of thermophilic films and fibers, with promising applications for wound dressings, micro-actuators and biofabrication.14 The responsivity of non-ionic UCST polymers is based on reversible hydrogen bonding, which enables stability against pH and salt changes in their environment.15 Nevertheless, in previous studies we have demonstrated that small amounts of ionic groups (1 mol%) in the polymer chain or even the sample preparation procedure can completely suppress the UCST behaviour in water.16 Thereby chemical instability in non-ionic UCST systems, which is mainly driven by hydrolysis, represents a main drawback since above the UCST (soluble polymer) the functional groups can be easily approached by water. One method to avoid the suppression of the UCST behaviour caused by ionic groups could be achieved with the addition of electrolytes to low molar mass PNAGA in aqueous solutions.17 However, chemical and long term stability in solution of UCST systems have to be improved to achieve further highly relevant applications. For example, in the pharmaceutical industry long periods of storage are needed18 as well as for implantable devices.19 In the case of PNIPAAm, chemical stability could be proved in different pH media from 3 to 10 by using pH sensitive copolymers. Nevertheless, PNIPAAm as a homopolymer is not stable under basic conditions.20 Other studies based on the chemical stability of LCST polymers were conducted by Wang et al.; they studied PNIPAAm block copolymers in bleach at different pH media, observing that chain degradation is dependent on the exposure conditions. Thermoresponsive regenerating properties after exposure to NaClO were also analyzed.21 On the other hand, intentional hydrolysis of polymer systems can be used to influence the polymer composition and its properties. Thereby, polyacrylamide can be transformed into a pH responsive polymer by controlled hydrolysis of the amide units into carboxylic acid.22 Further, various studies have been performed with thermoresponsive polymers and hydrolysis sensitive comonomers influencing properties such as phase transition temperature and polymer biodegradation.23–25
The aim of the present work is to investigate the effect of pH on the thermoresponsive behaviour of poly(AAm-co-AN)-UCST polymers. Chemical and thermoresponsive stability of poly(AAm-co-AN) samples, before and after exposure to “low” and “extreme” hydrolysis conditions with buffer media (pH 3, 6 and 9) as well as with NaOH (pH 14) and HCl (pH 0) solutions at 40 °C were studied. The influence of chemical additives, e.g. formamide on decreasing the hydrolysis rate of UCST polymers in aqueous media at basic pH and high temperatures (85 °C), was also investigated.
2. Experimental section
2.1 Materials
Acrylamide (Sigma-Aldrich, ≥99.9%, electrophoresis grade) and cyanomethyl dodecyl trithiocarbonate (CMDT, 98%; Aldrich) were used as received. Azobisisobutyronitrile (AIBN, Fluka) was recrystallized from ethanol. Acrylonitrile (Sigma-Aldrich, ≥99.9%), dimethyl sulfoxide (DMSO) and methanol were distilled prior to use. Ultrapure water was obtained using a filter. Buffer solutions (VWR) were used as received.
2.2 Analytical techniques
Cloud point measurements were performed on a JASCO V-630 UV/VIS-Spectrometer with an ETCS-761 Peltier thermostat single cell holder at 670 nm wavelength. The heating/cooling rate of 1.0 °C min−1 was constant for all measurements under magnetic stirring. The maximum of the first derivative of the transmittance curve was considered as the cloud point. 1H- NMR (300 MHz) spectra were recorded in d6-DMSO at room temperature on a Bruker Ultrashield-300 spectrometer. FTIR spectroscopy measurements for the analysis of polymer composition were performed on a Digilab Excalibur Series FTS 3000 spectrometer using a ZnSe crystal with attenuated total reflection technique. The analysis of the spectra was performed with the software Win-IR Pro 3.3. The AN content of poly(AAm-co-AN)s was determined using a calibration curve obtained by mixing homopolymer powders of polyacrylamide and polyacrylonitrile as standards in a specific composition. Gel permeation chromatography (GPC) measurements were carried out with DMSO for the determination of the molecular weights and respective molecular weight distributions. Two PLGel Mixed-D columns with the following properties were used: particle size 5 μm and dimension of 7.5 mm × 300 mm. GPC Pullulan standards were employed for calibration. Measurements were carried out at a flow rate of 1.0 mL min−1, and afterwards analysed with WinGpc7 software. Dialysis was carried out with Spectra/Por 6 dialysis tubes from regenerated cellulose (MWCO 3.500 g mol−1). The tubes were thoroughly washed with water before use to open the pores. The hydrolysis degree of the hydrolyzed freeze-dried samples was measured via element analysis (CHN) in an element analyzer EA 3000 (Fa. HEKAtech).
2.3 Synthesis
2.4 Preparation of hydrolyzed samples of poly(AAm-co-AN)
For the preparation of hydrolyzed samples, poly(AAm-co-AN) was dissolved in 2 mL of HCl (1 M) or NaOH (1 M), respectively. After dissolution, the samples were heated at 40 °C in a thermo-shaker (HLC MKR-13, Ditabis) at 250 rpm. Hydrolysis of the samples was stopped at different times (1 and 6 h). Subsequently, the pH of the solution was shifted to pH 4 after cooling at room temperature. All samples were dialyzed over 3 days against water. Dried polymer samples were obtained after 24 h freeze-drying. In order to quantify the degree of hydrolysis, elemental carbon–nitrogen analysis was carried out. The analysis was repeated three times for each sample. The hydrolysis percentage was calculated using the following formula (1): | 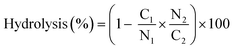 | (1) |
The mass percentages of carbon (C) and nitrogen (N) are represented with the subscripts 1 and 2 for non- and hydrolyzed samples, respectively.
3. Results and discussion
Poly(AAm-co-AN) is one of the few examples of a non-ionic system showing thermoresponsivity of UCST-type in water with sharp transitions and controlled cloud points depending upon the copolymer composition.26 Since AAm and AN are hydrolytically unstable groups, random copolymer samples of poly(AAm-co-AN) might show a changed thermoresponsive behavior under different pH conditions. Therefore, the effect of pH on UCST-type transitions was studied by taking copolymers of AAm and AN made by RAFT polymerization as representative examples. CMDT and AIBN were used as a chain transfer agent and radical initiator, respectively. Details of the controlled RAFT polymerization are given in the ESI in Table S1.† The first-order time-conversion plot and dependence of molar mass on conversion are linear up to about 70% conversion (ESI Fig. S2†), indicating a controlled polymerization. GPC elugrams of all polymers proved to be unimodal with no shoulders or signals of chain aggregation (ESI Fig. S3†). The obtained experimental molar masses (Mn) were lower than the theoretical molar masses (Mn,theo). This type of deviation is attributed to the standards used for GPC calibration (Pullulan), since a relative method was used for molar mass determination. Similar controlled polymerization behavior was observed for UCST-type polymers of poly(AAm-co-St).13 The synthesized samples of poly(AAm87-co-AN12) and poly(AAm70-co-AN16) with molar masses Mn = 20
300 Da and Mn = 24
700 Da, respectively, were used for further hydrolysis experiments. Turbidity curves at pH 3 and 9 (buffer solutions) of poly(AAm87-co-AN12) are compared to the obtained curve in Millipore water in Fig. 1A. Apparently, the influence of pH on the cloud point is negligible, if the measurements are carried out immediately after dissolution of the samples. Repeated measurements after 25 days of dissolution of the same samples showed no change in the cloud point at pH 3, but led to complete disappearance (at least until 0 °C) of thermoresponsive behavior at pH 9. Hou et al. showed that the influence of pH on the thermoresponsive behavior of poly(AAm-co-AN) samples was unimportant, at least after dissolution of the polymers. However, stability studies under different pH conditions were not carried out.27 Whereas the phase transition temperature of poly(AAm-co-AN) was not significantly affected by pH after dissolution, the ions that follow the Hofmeister series do influence hydrogen bonding of macromolecules. The effect of ions has been thoroughly investigated on thermoresponsive polymers with LCST like PNIPAAm28 as well as for non-ionic UCST-type polymers.17 Therefore, the differences observed in the phase transition temperatures in different pH buffer media are due to the presence of chaotropes and kosmotropes, such as K+, Na+, Cl−, OH−, and citrate anions, with a variation of the ionic strength between μ = 1 and 0.1 M (Fig. 1B).
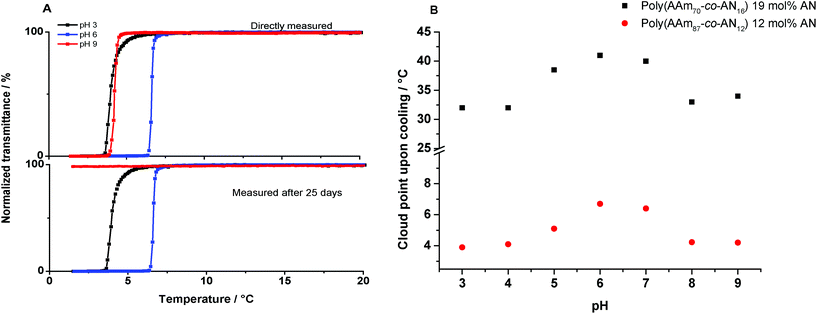 |
| Fig. 1 (A) Turbidity curves (cooling) of poly(AAm87-co-AN12) in Millipore water (pH 6) and buffer solutions of pH 3 and 9 as storage media over 25 days. (B) Cloud point shift in non-hydrolyzed samples by using different buffer solutions from pH 3 to pH 9 (Table 1, entries 1 and 2). | |
The obtained results at pH 3 (buffer solution) after 25 days showed the tolerance of poly(AAm-co-AN) samples to acidic media. Experiments were also carried out under extreme acidic conditions, pH 0 and 40 °C, over 1 h and 6 h, respectively where we expected an increase of polymer hydrolysis and a strong influence on the transition temperatures. After 6 h under the applied conditions no thermoresponsive behavior for the used polymer samples (Table 1, entries 1 and 2) could be observed in Millipore water. Nevertheless, after 1 h treatment the phase transition temperature of poly(AAm70-co-AN16) could be observed in aqueous media. As expected, the cloud point of the sample decreased from 35 °C to 22 °C, due to the formation of carboxylic ions, which increased the hydrophilicity of the copolymer. 1H NMR and FTIR showed slight changes of the chemical structure after acidic hydrolysis, indicating the presence of carboxyl groups, however quantification was not possible (see ESI, Fig. S4 and S5†). Recovery of the lost or decreased thermoresponsivity could be achieved at pH 3 due to protonation of the carboxyl groups (Fig. 2).
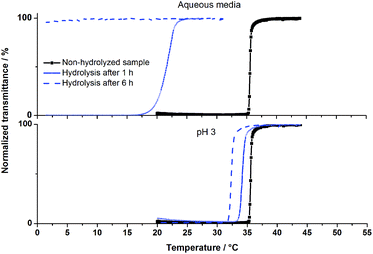 |
| Fig. 2 Turbidity curves (upon cooling) of poly(AAm70-co-AN16) before and after hydrolysis in HCl (pH 0) at 40 °C. Measurements were carried out in aqueous media as well as at pH 3 (HCl). | |
In contrast to the observed stability of poly(AAm-co-AN) samples under acidic conditions, the thermoresponsivity of the polymers was lost after hydrolysis using a buffer of pH 9 at room temperature (RT). Fig. 3A shows the time-dependent decrease of the cloud points within 25 days at pH 9. The disappearance of UCST-type transition at pH 9 is attributed to the hydrolysis of AAm and AN, which lead to the formation of carboxylate groups. The role of ionic groups in suppressing the UCST of PNAGA was shown by us previously.16 The conventional characterization techniques such as NMR and FTIR did not show any appreciable change in the chemical structure, which is due to the presence of carboxyl groups in amounts less than the detectable limits of these techniques (see ESI, Fig. S6 and S7†). Further, due to the mild hydrolysis conditions such as a buffered media and RT, the thermoresponsive behavior could be increased or regenerated using low pH aqueous media (acidic buffer or HCl). The responsive behavior of each hydrolyzed polymer within these 25 days at pH 9 (buffer solution) was recovered by protonating the carboxylic anions at pH 3 (Fig. 3B). The polymers were not responsive in Millipore water, after more than 14 days of hydrolysis in pH 9. The biggest recovery effect could be observed after 25 days of storage; at this point, no thermoresponsive behavior could be observed for the hydrolyzed sample. However, after dissolution of the polymer at pH 3, the phase transition behaviour was regenerated.
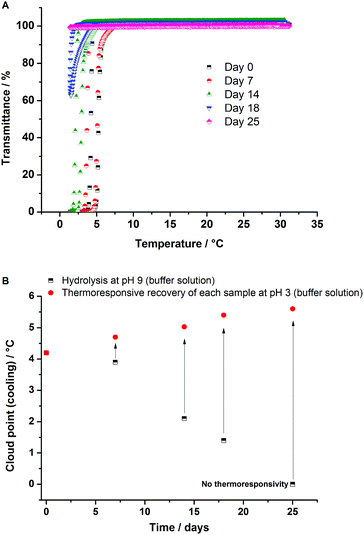 |
| Fig. 3 (A) Gradual loss of thermoresponsivity of poly(AAm87-co-AN12) under alkaline conditions (buffer solution at pH 9) over 25 days. (B) Recovery of the thermoresponsive behaviour at low pH conditions (buffer pH 3) after hydrolysis at pH 9. | |
Whereas poly(AAm-co-AN) samples hydrolyzed slowly in buffer solutions of pH 9, a faster loss of thermoresponsivity could be observed in NaOH solutions of pH 9, when measured after 10 h (Fig. 4A). The regeneration of responsivity of these polymers is presented in Fig. 4B. The recovery of the phase transition temperature by protonation of the carboxylic anions could be achieved with HCl as well as with buffer solutions of pH 3. A further regenerating effect could be achieved by addition of NaCl (33 mM) as chaotropic salt. Chaotropic salts are known to screen the charges of the formed ionic groups.15
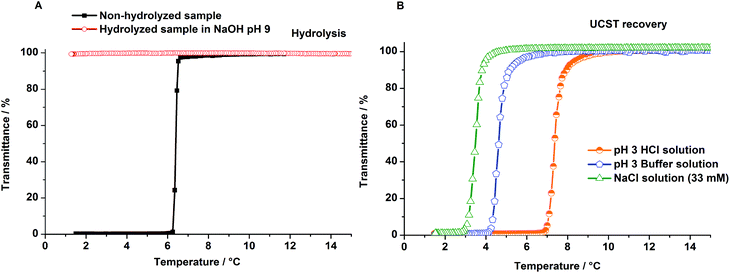 |
| Fig. 4 (A) Hydrolysis of poly(AAm87-co-AN12) in NaOH at RT (10 h). (B) Recovery of the thermoresponsive behavior of a hydrolyzed sample of poly(AAm87-co-AN12) in NaOH with solutions of pH 3 (buffer and HCl) and with aqueous solutions with NaCl as the chaotropic agent (33 mM). | |
Further, to analyze the chemical stability of poly(AAm-co-AN) and the impact of higher hydrolysis degrees, a harder base catalyzed hydrolysis in NaOH solutions (1 M) of pH 14 at 40 °C was used. The samples were hydrolyzed under these conditions over 1 and 6 h, respectively. The polymers were hydrolyzed in the range of 39–44% (according to elemental analysis). FTIR spectra of poly(AAm87-co-AN12) hydrolyzed samples are shown in Fig. 5A. Hydrolyzed samples showed a significant decrease of the C
N band at 2240 cm−1 to nearly total disappearance after 6 h hydrolysis. Additional bands were observed at 1406 and 1560 cm−1. These correspond to the symmetric and the asymmetric stretching mode of the carboxylate ion respectively. Kampalanonwat et al. have shown a similar decrease of the C
N band, due to the formation of the carboxylic acid units by hydrolysis of PAN with NaOH ethanolic/aqueous solutions at 75 °C.29 The hydrolyzed samples also showed significant changes in the 1H NMR (see Fig. S8†), since all signals of the samples were influenced by the NaOH treatment including the reference signal of the RAFT agent (–CH3) at 0.85 ppm, the analysis was carried out using dichloroethane (DCE) as the internal standard (10 mg mL−1).
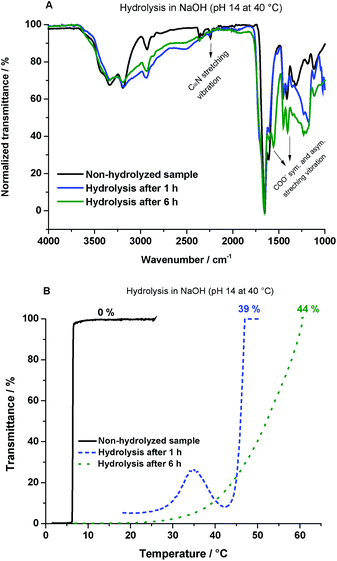 |
| Fig. 5 (A) FTIR spectra of non- and hydrolyzed poly(AAm87-co-AN12). Hydrolysis was carried out in NaOH (pH 14) at 40 °C. (B) Turbidity curves (upon cooling) of poly(AAm87-co-AN12) before and after hydrolysis in NaOH (pH 14) at 40 °C. The polymers were dissolved in HCl solutions (pH 3, 1 wt%). | |
Fig. 5B, shows the thermoresponsive behavior with an increasing hydrolysis degree. Thermoresponsivity in pure water could not be observed. Therefore, the samples were dissolved in aqueous solutions where the pH was adjusted to pH 3. In these solutions, a significant increase of the phase transition temperature from 6 to 47 °C could be observed after 1 h hydrolysis. In this case, the solution became clear to 27% transmittance as it was cooled down to 35 °C. By further cooling, the sample became turbid again. This behavior can indicate a short time settling of the polymer at the bottom, which can decrease the total concentration of the sample in the solution. After 6 h of hydrolysis, only a broad phase transition temperature could be measured. Table 2 summarizes the dependency between the hydrolysis degree and thermoresponsive behavior at pH 3.
Table 2 Influence of hydrolysis degree on the cloud point of poly(AAm87-co-AN12) after hydrolysis in NaOH (pH 14) at 40 °C
Hydrolysis time/h |
Hydrolysis degreea/% |
(O) contenta/% |
Cloud point (cooling)/°C |
Values determined by elemental analysis.
|
0 |
— |
25 |
6 |
1 |
39 |
33 |
47 |
6 |
44 |
36 |
53 |
For the copolymer sample poly(AAm70-co-AN16), with a higher content of AN (Table 1, entry 2), the NaOH hydrolysis changed the transition temperature considerably. These samples, showed in general formation of a “gel” structure after sample preparation in pH 3. The one hour hydrolyzed sample showed a slight turbidity curve with broad hysteresis (Fig. 6A). The lowering of the transition temperature can be due to the low solubility of the polymer in the solution. Longer periods of hydrolysis (6 h) increased directly the formation of an interpolymer complex “gel” (Fig. 6B). This physical crosslinked structure was not soluble in low pH media, whereas it dissolved in neutral or basic media, due to the presence of negative charge carboxylic groups. In this case, no transition temperatures could be measured.
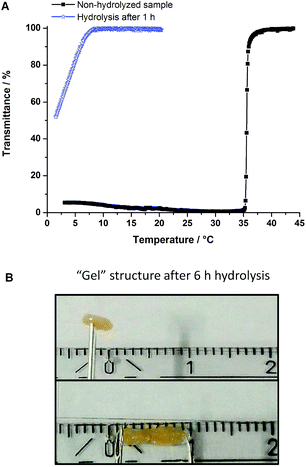 |
| Fig. 6 (A) Turbidity curves (upon cooling) of poly(AAm70-co-AN16) before and after hydrolysis in NaOH pH 14 at 40 °C. The polymers were dissolved in HCl solutions (pH 3, 1 wt%). (B) Interpolymer complex “gel” in pH 3 (hydrolyzed sample over 6 h in NaOH pH 14 at 40 °C). | |
The addition of chemical additives could offer besides the use of buffer solutions, a further alternative to protect the responsive behavior of UCST polymers over longer periods, even under basic conditions (pH 9) and high temperatures (85 °C). Especially sacrificial additives like formamide can delay the hydrolysis of poly(AAm-co-AN) samples without significant interference with the thermoresponsive behavior. The low molar mass formamide is probably preferentially hydrolysed due to the unsubstituted carbonyl carbon in comparison to AAm or AN units of the polymer chain. The steric hindrance for the approaching nucleophile is less for formamide in comparison to the hydrolyzable units (AAm and AN) attached to a high molar mass sterically hindered polymer backbone. Experimental studies are known in the literature showing an order of magnitude greater hydrolysis rate for N-methylformamide in neutral water than N-methylacetamide (H attached to the carbonyl carbon of N-methylformamide is replaced with a methyl group) under the same experimental conditions.30 Moreover, for the base catalysed hydrolysis, the larger substituents restrict the number of water molecules approaching the attacking hydroxide oxygen in the transition state. The free energy barrier for hydrolysis, therefore increases due to the destabilization of the transition state making hydrolysis slower for substituted amides.31
As shown in Fig. 7A, the addition of formamide as a sacrificial additive preserved the thermoresponsivity in NaOH solution of poly(AAm87-co-AN12) at pH 9 over 10 h, whereas the same sample (without additive in NaOH solution) lost the thermoresponsive behavior within the same hydrolysis time. The use of high temperatures over 75 °C has shown the hydrolysis of PNAGA and therefore the loss of thermoresponsivity.16Fig. 7B shows a similar hindered responsivity of poly(AAm87-co-AN12) after 1 h at 85 °C. Whereas, the addition of formamide to the aqueous solution protected the polymer from hydrolysis, preserving the responsive behavior within 9 h.
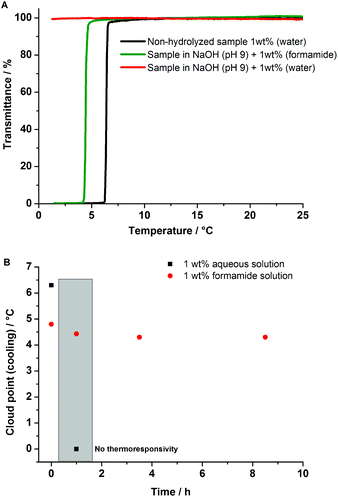 |
| Fig. 7 (A) Hydrolysis stability of poly(AAm87-co-AN12) in NaOH (pH 9), with and without sacrificial additives. (B) Stability of the thermoresponsive behavior in aqueous media of poly(AAm87-co-AN12) at 85 °C depending on time, with and without sacrificial additives. | |
4. Conclusion
Controlled polymerization of AAm and AN could be achieved by RAFT using CMDT as a CTA. The obtained polymers showed narrow molar mass distributions, displaying sharp phase transition temperatures in aqueous media. In the present work it could be shown that the synthesized poly(AAm-co-AN) copolymers have chemical and thermoresponsive stability under acidic and neutral conditions. In the past, we have reported the importance of avoiding ionic units to ensure thermoresponsive behavior of UCST polymers like PNAGA. Nevertheless, the capability of poly(AAm-co-AN) samples to hydrolyze led to a pH dependent UCST-type behavior. Meanwhile, the use of alkaline buffer media or NaOH solutions increased the hydrolysis rate of the polymers, and therefore the formation of ionic groups until the disappearance of the phase transition temperature. The lowering or recovery of responsivity in hydrolyzed samples could be achieved by addition of salts, e.g. NaCl, due to their shielding effect, or by protonation of the carboxylic groups at low pH. Furthermore, the hydrolysis rate under alkaline conditions (pH 9, RT) could be decreased by addition of formamide as a sacrificial agent, thus the UCST behavior could be maintained over 10 h under these conditions. The protecting effect of formamide on thermoresponsivity was even observed at high temperature (85 °C) in aqueous media. This work provides a background for the chemical stability of non-ionic UCST-type polymers and provides the possibility of varying the phase transition temperature under pH dependent conditions using intentional hydrolysis in acidic or alkaline media.
Acknowledgements
The authors would like to thank the Deutsche Forschungsgemeinschaft (DFG) for financial support.
References
- D. Roy, W. L. Brooks and B. S. Sumerlin, Chem. Soc. Rev., 2013, 42, 7214–7243 RSC.
- S. R. Abulateefeh, S. G. Spain, J. W. Aylott, W. C. Chan, M. C. Garnett and C. Alexander, Macromol. Biosci., 2011, 11, 1722–1734 CrossRef CAS PubMed.
- X. Liang, F. Liu, V. Kozlovskaya, Z. Palchak and E. Kharlampieva, ACS Macro Lett., 2015, 4, 308–311 CrossRef CAS.
- B. Kong, J. S. Choi, S. Jeon and I. S. Choi, Biomaterials, 2009, 30, 5514–5522 CrossRef CAS PubMed.
- A. Prasannan, H.-C. Tsai, Y.-S. Chen and G.-H. Hsiue, J. Mater. Chem. B, 2014, 2, 1988–1997 RSC.
- D. Q. Wu, F. Qiu, T. Wang, X. J. Jiang, X. Z. Zhang and R. X. Zhuo, ACS Appl. Mater. Interfaces, 2009, 1, 319–327 CAS.
- J. Hu and S. Liu, Macromolecules, 2010, 43, 8315–8330 CrossRef CAS.
- A. Nykänen, M. Nuopponen, A. Laukkanen, S. Hirvonen, M. Rytelä, O. Turunen, H. Tenhu, R. Mezzenga, O. Ikkala and J. Ruokolainen, Macromolecules, 2007, 40, 5827–5834 CrossRef.
- F. Liu, J. Seuring and S. Agarwal, Macromol. Chem. Phys., 2014, 215, 1466–1472 CrossRef CAS.
- F. Liu and S. Agarwal, Macromol. Chem. Phys., 2015, 216, 460–465 CrossRef CAS.
- M. Boustta, P. E. Colombo, S. Lenglet, S. Poujol and M. Vert, J. Controlled Release, 2014, 174, 1–6 CrossRef CAS PubMed.
- G. Huang, H. Li, S. Feng, X. Li, G. Tong, J. Liu, C. Quan, Q. Jiang, C. Zhang and Z. Li, Macromol. Chem. Phys., 2015, 216, 1014–1023 CrossRef CAS.
- B. A. Pineda-Contreras, F. Liu and S. Agarwal, J. Polym. Sci., Part A: Polym. Chem., 2014, 52, 1878–1884 CrossRef CAS.
- F. Liu, S. Jiang, L. Ionov and S. Agarwal, Polym. Chem., 2015, 6, 2769–2776 RSC.
- J. Seuring and S. Agarwal, Macromol. Rapid Commun., 2012, 33, 1898–1920 CrossRef CAS PubMed.
- J. Seuring, F. M. Bayer, K. Huber and S. Agarwal, Macromolecules, 2012, 45, 374–384 CrossRef CAS.
- F. Liu, J. Seuring and S. Agarwal, J. Polym. Sci., Part A: Polym. Chem., 2012, 50, 4920–4928 CrossRef CAS.
-
L. V. Allen
, Secundum Artem, 7 (3), 1–6: (Resource on the Internet 20.01.2016).
- A. Peled, A. Pevzner, H. Peretz Soroka and F. Patolsky, J. Nanobiotechnol., 2014, 12, 1–11 CrossRef PubMed.
- N. do Nascimento Marques, P. Schroeder Curti, A. M. da Silva Maia and R. de Carvalho Balaban, J. Appl. Polym. Sci., 2013, 129, 334–345 CrossRef CAS.
- Z. Wang and R. Pelton, Eur. Polym. J., 2013, 49, 2196–2201 CrossRef CAS.
- W. Li, H. Zhao, P. R. Teasdale, R. John and S. Zhang, React. Funct. Polym., 2002, 52, 31–41 CrossRef CAS.
- D. Neradovic, C. F. Van Nostrum and W. E. Hennink, Macromolecules, 2001, 34, 7589–7591 CrossRef CAS.
- Z. Cui, B. H. Lee and B. L. Vernon, Biomacromolecules, 2007, 8, 1280–1286 CrossRef CAS PubMed.
- Q. Zhang, N. Vanparijs, B. Louage, B. G. De Geest and R. Hoogenboom, Polym. Chem., 2014, 5, 1140–1144 RSC.
- J. Seuring and S. Agarwal, Macromolecules, 2012, 45, 3910–3918 CrossRef CAS.
- L. Hou and P. Wu, Soft Matter, 2015, 11, 7059–7065 RSC.
- Y. Zhang, S. Furyk, D. E. Bergbreiter and P. S. Cremer, J. Am. Chem. Soc., 2005, 127, 14505–14510 CrossRef CAS PubMed.
- P. Kampalanonwat and P. Supaphol, Ind. Eng. Chem. Res., 2011, 50, 11912–11921 CrossRef CAS.
- P. Duana, L. Daib and P. E. Savagea, J. Supercrit. Fluids, 2010, 51, 362–368 CrossRef.
- Y. Xiong and C. G. Zhan, J. Phys. Chem. A, 2006, 110, 12644–12652 CrossRef CAS PubMed.
Footnote |
† Electronic supplementary information (ESI) available: Controlled RAFT polymerization of AAm and AN, characterization (FTIR and 1H NMR) of hydrolyzed samples in HCl (pH 0 at 40 °C), buffer solution (pH 9 at RT) and 1H NMR of hydrolyzed samples in NaOH (pH 14 at 40 °C). See DOI: 10.1039/c6py00162a |
|
This journal is © The Royal Society of Chemistry 2016 |
Click here to see how this site uses Cookies. View our privacy policy here.