DOI:
10.1039/C5NJ02444G
(Paper)
New J. Chem., 2016,
40, 838-843
MoO2Cl2 catalyzed efficient synthesis of functionalized 3,4-dihydropyrimidin-2(1H)-ones/thiones and polyhydroquinolines: recyclability, fluorescence and biological studies†
Received
(in Montpellier, France)
11th September 2015
, Accepted 9th November 2015
First published on 30th November 2015
Abstract
A simple, facile and efficient synthesis of functionalized dihydropyrimidinones and polyhydroquinolines using molybdenum(VI) dichloride dioxide (MoO2Cl2) has been developed. The present protocol demonstrates the exceptional tolerance towards acid labile protecting groups such as tert-butyl dimethyl silyl (TBDMS) and tert-butyl diphenyl silyl (TBDPS). This is the first report of exploring Lewis acid properties of MoO2Cl2 in the diversity oriented synthesis of Biginelli and Hantzsch reactions. Biologically important and highly structured conjugates of dihydropyrimidinone and polyhydroquinoline derivatives containing coumarin, pyrazole, indole and triazole moieties were synthesized in good to excellent yields. Compound 4o exhibited blue fluorescence at a maximum UV absorbance λmax of 326 nm. In the preliminary MTT [3-(4,5-dimethylthiazol-2-yl)-2,5-diphenyltetrazolium bromide] assay, compound 4n displayed remarkable cytotoxic activity against A549 and PC3 cell lines while compound 7q was found to be cytotoxic against HGC-27 and PC3 cancer cell lines.
Introduction
Molybdenum coordination chemistry is one of the intriguing research areas due to the inevitable occurrence of Mo in the active sites of molybdoenzymes.1 Some of the potential applications of molybdenum compounds such as corrosion inhibitors,2a lubricants,2a,b pigments,2c,d smoke suppressants,3a and fertilizers3b have also been reported. Moreover, recently, molybdenum(VI) dichloride dioxide (MoO2Cl2) has been gaining importance on account of its ease of availability, less toxicity, thermal stability and water tolerant nature.4a,b In addition, many research groups are exploiting the potential of MoO2Cl2 in modern organic synthesis.4c It has been widely used as an efficient catalyst for several organic transformations such as the hydrosilylation of aldehydes and ketones,5a the hydrophosphonylation of aldehydes,5b the reduction of imines, esters, sulfoxides and pyridine N-oxides to the corresponding amines, alcohols, sulfides and pyridines respectively,5c,d the epoxidation of double bonds, the oxidation of alcohols to carbonyl compounds,5e the conversion of β-hydroxycarbonyls into α-bromo 1,3-dicarbonyls,6a the formation of carbamates from alcohols and isocyanates.6b,c Multi-component reactions (MCRs) have emerged as an efficient and powerful tool in combinatorial chemistry with the facile and rapid creation of several multiple bonds in a one-pot reaction with minimal waste of time and energy.7 Transition-metal catalyzed organic transformations comply with the principles of “Green Chemistry” such as the minimization of waste, clean catalysts/reagents and minimum use of energy. In recent decades, dihydropyrimidinones and polyhydroquinolines derivatives (Biginelli and Hantzsch products) are the promising scaffolds that have gained importance in organic and medicinal chemistry owing to their pharmacological properties. Dihydropyrimidinones exhibit a broad range of biological activities such as anticancer, calcium channel modulator, anti-hypertensive, anti-viral, anti-oxidant, anti-bacterial, anti-inflammatory, neuropeptide Y (NPY) antagonist and α1a-adrenergic antagonist.8 Polyhydroquinolines are often used as antitumor, vasodilator, hepatoprotective, antiatherosclerotic, geroprotective, antidiabetic, antiasthmatic, antibacterial, anti-inflammatory and tyrosine kinase inhibitors.9–11 Very recently, coumarin-dihydropyrimidinone hybrids have been reported to exhibit fluorescent properties, which can be further utilized in the synthesis of new biological and chemical probes.12
In view of the biological, industrial and synthetic importance of polyhydroquinolines and dihydropyrimidinones, a plethora of protocols and catalytic systems have been developed for these significant organic transformations. However, the application of non-noble metal complexes based on molybdenum (MoO2Cl2) as a Lewis acid catalyst in the diversity oriented synthesis of dihydropyrimidinones/thiones and polyhydroquinolines remains unexplored. To the best of our knowledge this is the first report of using MoO2Cl2 as a homogenous catalyst in the synthesis of dihydropyrimidinone/thione and polyhydroquinoline derivatives via a Biginelli and Hantzsch reaction (Scheme 1).
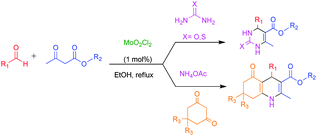 |
| Scheme 1 MoO2Cl2 catalyzed synthesis of dihydropyrimidinone/thiones and polyhydroquinolines. | |
Results and discussion
Synthesis of dihydropyrimidinones/thiones and polyhydroquinolines
A Biginelli test reaction was performed involving a mixture of benzaldehyde, ethyl acetoacetate, urea in the presence of MoO2Cl2 (0.5 mol%) in EtOH at room temperature for 24 h (Table 1, entry 1), and the dihydropyrimidinone product was obtained in less yield due to the incomplete conversion of the reactants, thus it was assumed that thermal energy may be required to drive the reaction to completion. Furthermore, the effect of variation in temperatures from 50 °C to reflux conditions, along with an increase in catalyst concentrations were studied (Table 1, entries 2–5). To our delight, it was observed that 1 mol% of MoO2Cl2 in EtOH at reflux temperature afforded 95% yield in a short reaction time (Table 1, entry 4) and a further increment in catalyst concentration does not affect the reaction yield (Table 1, entry 5). Moreover, the catalytic efficiency of MoO2Cl2 was examined using different solvents (Table 1, entries 6–9). The yields and the reaction time required depict that protic solvents (water, MeOH and EtOH) were more preferable than aprotic solvents (acetonitrile, THF). Among the protic solvents, EtOH gave the best results (Table 1, entry 4). A control experiment in the absence of a catalyst resulted in nominal yield which proves the emphasis on the importance of a catalyst (Table 1, entry 10).
Table 1 Optimization of reaction conditions for the synthesis of dihydropyrimidinone 4aa
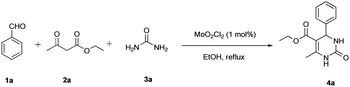
|
Entry |
MoO2Cl2 (mol%) |
Solvent |
Temperature (°C) |
Time (h) |
Yieldb (%) |
Reaction conditions: benzaldehyde (1a, 1 mmol), ethyl acetoacetate (2a, 1 mmol), urea (3a, 1.5 mmol) in EtOH (10 mL).
Isolated yield.
|
1 |
0.5 |
EtOH |
rt |
24 |
35 |
2 |
0.5 |
EtOH |
50 |
3 |
64 |
3 |
0.5 |
EtOH |
Reflux |
2 |
80 |
4 |
1 |
EtOH |
Reflux |
1 |
95 |
5 |
2 |
EtOH |
Reflux |
1 |
96 |
6 |
1 |
Water |
Reflux |
2 |
75 |
7 |
1 |
MeOH |
Reflux |
2 |
72 |
8 |
1 |
CH3CN |
Reflux |
4 |
78 |
9 |
1 |
THF |
Reflux |
6 |
67 |
10 |
— |
EtOH |
Reflux |
12 |
15 |
With the optimized reaction conditions in hand (Table 1, entry 4), the generality and scope of the present one-pot, three-component reaction was explored. The reaction with different aromatic aldehydes containing electron donating and withdrawing groups afforded excellent yields of 85–96% in 40 min to 2 h (Fig. 1). Interestingly, substrates involving acid sensitive protecting groups such as TBDMS and TBDPS afforded good yields with the absence of deprotected side products (Fig. 1, 4k and 4l). The present protocol was amenable to substituted heterocyclic aldehydes such as coumarin, pyrazole and thiophene where the reaction proceeded smoothly with excellent yields (Fig. 1, 4m and 4n) except in the case of coumarin derivatives; a moderate yield of 75% was obtained after 2 h (Fig. 1, 4o). Similarly, thiopyrimidinones were also synthesized with high yields (Fig. 1, 4g–4i and 4p).
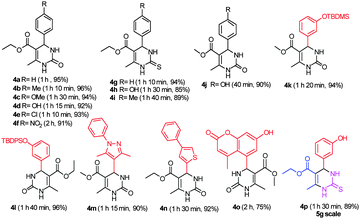 |
| Fig. 1 Different dihydropyrimidinone/thiones synthesized via a one-pot three component Biginelli reaction. | |
To test the feasibility of the reaction, a 5g scale synthesis of monastrol (a specific inhibitor of mitotic kinesin Eg5) was attempted. 3-Hydroxy benzaldehyde, ethyl acetoacetate, thiourea were stirred under the optimized reaction conditions and achieved monastrol in 89% yield with 97.99% purity (ESI†) (Scheme 2).
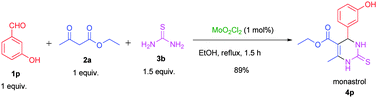 |
| Scheme 2 MoO2Cl2 catalyzed 5 g scale synthesis of monastrol. | |
Encouraged with the successful synthesis of dihydropyrimidinones/thiones, our attention was shifted towards the synthesis of polyhydroquinolines. Polyhydroquinolines are generally synthesized via a Hantzsch reaction (a one-pot, four component reaction) using aldehyde, dimedone, β-ketoester and ammonium acetate. Several protocols have been reported for the synthesis of polyhydroquinolines13–19 but still emphasis on the efficacy of the catalyst with high yields of products creates requisite to explore newer methodologies. Fascinatingly, we found the potential utility of MoO2Cl2 in one-pot, four component synthesis of polyhydroquinolines in admirable yields. Various substituted aromatic and heteroaromatic aldehydes (1 mmol) were treated with dimedone/1,3-cyclohexanedione (1 mmol), β-ketoesters (1 mmol), ammonium acetate (1.2 mmol) in the presence of MoO2Cl2 (1 mol%) in EtOH at reflux temperature. Gratifyingly, the desired products (7a–s) were formed in 20–40 min with 80–94% yields as shown in Table 2. Polyhydroquinolines with an acid labile protecting group (TBDMS) were synthesized in superior yields (Table 2, 7g, 7j). Triazole and indole containing substrates were also employed in the synthesis of polyhydroquinolines and the desired products were accomplished in excellent yields (Table 2, 7q, 7s). Large scale synthesis of 7k (Table 2) was performed using benzaldehyde, ethyl acetoacetate, dimedone and ammonium acetate to obtain desired products with 85% yield.
Table 2 MoO2Cl2 catalyzed synthesis of polyhydroquinolinesa
Recyclability
From the economical point of view as well as environmental concern, the recyclability of the MoO2Cl2 catalyst was tested upon the condensation of benzaldehyde, 1,3-cyclohexandione, ethyl acetoacetate and ammonium acetate using 1 mol% of MoO2Cl2 in EtOH (Table 2, entry 1).
After the completion of the reaction, EtOH was evaporated and ethylacetate was added to the reaction mixture followed by usual aqueous workup. The aqueous layer was separated, decanted, and dried to recover MoO2Cl2. Later, the recovered MoO2Cl2 catalyst was reused for 5 consecutive cycles. The yields obtained after each run are depicted in Fig. 2.
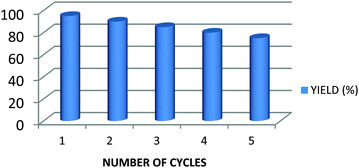 |
| Fig. 2 Catalyst recyclability chart of compound 7a. | |
A plausible mechanistic pathway for the synthesis of dihydropyrimidinones/thiones is outlined in Scheme 3, which is in similarity with the established mechanism as reported in the literature.20 The one-pot Biginelli reaction may be initiated with the reaction of aldehyde and urea followed by condensation activated by the co-ordination of MoO2Cl2 resulting in the formation of acyl imine intermediate 8, a rate limiting step. Further, the iminium intermediate 9 undergoes nucleophilic attack by the β-dicarbonyl ester enolate to produce open chain intermediate ureide 11, followed by cyclization and subsequent loss of water to afford dihydropyrimidinone 4a.
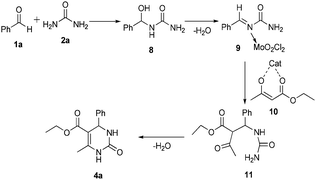 |
| Scheme 3 Plausible mechanism for dihydropyrimidione formation using MoO2Cl2. | |
A plausible mechanism for the MoO2Cl2 mediated Hantzsch reaction towards the synthesis of polyhydroquinoline is also proposed. First, aldehyde is activated by MoO2Cl2 and dimedone in enol form reacts in the Knoevenagel fashion to give intermediate 13. On the other hand, the MoO2Cl2 activated β-ketoester and ammonium acetate afford enamine 14. Then, intermediate 13 and enamine 14 undergo a Michael reaction followed by intramolecular condensation to produce 7 (Scheme 4).
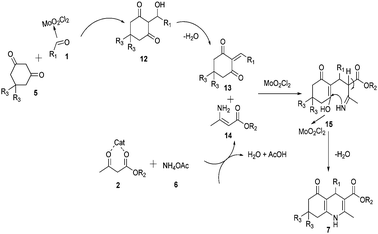 |
| Scheme 4 Plausible mechanism for polyhydroquinoline formation using MoO2Cl2. | |
Spectroscopic properties of compound (4o)
Further, we measured the absorbance and emission maxima of umbelliferone and coumarin-dihydropyrimidinone derivatives (4o) in methanol at 30 μm concentration (Table 3). The fluorescent emission spectrum of compound 4o was quite similar to umbelliferone with a Stokes shift of 71 nm. Blue fluorescence was observed when 4o was irradiated at maximum UV absorbance λmax 326 nm (Fig. 3). This result could be an inception to utilize the properties of compound 4o in further development of the chemical probes.
Table 3 Fluorescence properties of coumarin derivatives
Compound |
Absorbance λmax (nm) |
Emission λmax (nm) |
Stoke shift (nm) |
Values were obtained from ref. 21.
|
Umbelliferonea |
324 |
392 |
68 |
4o
|
326 |
397 |
71 |
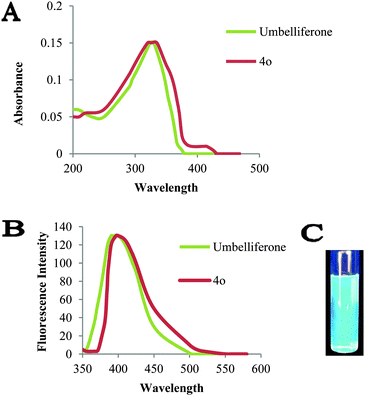 |
| Fig. 3 (A) Normalized UV-vis spectra of MeOH. (B) Normalized fluorescence emission spectra of MeOH with excitation on λmax of the compound. All spectra were recorded at a concentration of 30 μm in MeOH. (C) Blue fluorescence of compound 4o was observed at λmax 326 nm. | |
Biological evaluation
In vitro evaluation.
Among all the synthesized compounds 4m, 4n, 4o, 7q and 7s were found to be diverse and highly functionalized molecules. Similar scaffolds have been reported earlier for their potent biological activities.22 This prompted us to further examine the in vitro cytotoxic potential of aforementioned compounds. These compounds were evaluated against four cancer cell lines namely, A549 (lung cancer), HGC-27 (gastric cancer) and PC3 (prostate cancer), BT-549 (breast cancer) by employing the MTT assay. Concentration response course analysis was performed to determine drug concentrations required to inhibit the growth of cancer cells by 50% (IC50) after incubation for 48 h. The results of in vitro anticancer activity revealed that compound (4n) showed IC50 of 10.4 μM against A549 and 19.3 μM for PC3 cancer cell lines. Compound 7q showed IC50 of 15.6 μM against HGC-27 and 19.4 μM for PC3 cancer cell lines (Table 4). These preliminary results indicate that compounds 4n and 7q could be the potential leads for the development of novel anticancer agents.
Table 4
In vitro anticancer activity (IC50 μM)
S. No. |
Compound |
A549 |
HGC-27 |
PC3 |
BT 549 |
NA: not active. |
1 |
4m
|
NA |
NA |
NA |
NA |
2 |
4n
|
10.4 ± 1.7 |
22.1 ± 2.2 |
19.3 ± 1.3 |
26.8 ± 2.7 |
3 |
4o
|
31.3 ± 0.9 |
36.4 ± 1.3 |
36.3 ± 1.2 |
20.5 ± 0.7 |
4 |
7q
|
28.6 ± 2.6 |
15.6 ± 2.4 |
19.4 ± 1.6 |
NA |
5 |
7s
|
NA |
NA |
NA |
NA |
Conclusion
An efficient and operationally simple protocol was developed for the synthesis of dihydropyrimidinones and polyhydroquinolines. The MoO2Cl2 catalyst offers striking advantages such as less-toxicity, water-tolerance, thermal-stability, and most importantly its reusability. MoO2Cl2 was reused for 5 consecutive cycles and desired products were obtained in good to moderate yields. Large scale syntheses were also established with good yields and in a short reaction time. Heterocyclic aldehydes were amenable in the present protocol. In the present method, acid sensitive protecting groups such as tert-butyl dimethyl silyl and tert-butyl diphenyl silyl were well tolerated. The coumarin-dihydropyrimidinone hybrid (4o) exhibited blue fluorescence at λmax 326 nm, which could be useful as a chemical probe. Compounds 4n and 7q displayed promising anticancer activity directing towards the development as the potential anticancer agents.
Experimental section
General
The MoO2Cl2 catalyst (Product no: 373710) was procured from Sigma Aldrich. Compounds were characterized by nuclear magnetic resonance using 300 and 500 spectrometers. 1H NMR spectra were measured at 300 and 500 MHz. 13C NMR spectra were measured at 75 and 125 MHz. Mass spectrometric studies were carried out on an Agilent 1200 series LC instrument coupled with a QTOF mass spectrometer (Q-TOF LC/MS 6540 series equipped with an ESI source and operated in the positive ionization mode). UV absorption spectra and Fluorescence spectra were recorded on a Spectramax M4 spectrophotometer with a quartz cell of 10 mm optical path.
General procedure for the synthesis of dihydropyrimidinones/thiones from substituted benzaldehydes (Fig. 1, 4a–p).
A mixture of aldehyde 1 (1 mmol), methyl/ethyl acetoacetate 2 (1 mmol), and urea/thiourea 3 (1.5 mmol) in EtOH (10 mL) was refluxed in the presence of MoO2Cl2 (1 mol%). After the completion of the reaction, as indicated by TLC analysis, the solvent was evaporated. The resulting mass was extracted with ethyl acetate (3 × 10 mL) followed by the treatment of brine. The combined organic layers were evaporated and dried over anhydrous Na2SO4. Recrystallization using ethanol was performed to give pure product 4.
General procedure for the synthesis of polyhydroquinolines from substituted benzaldehydes (Table 2, 7a–t).
To a mixture of aldehyde 1 (1 mmol), methyl/ethyl acetoacetate 2 (1 mmol), and 1,3-cyclohexanedione/dimedone 5 (1 mmol), NH4OAc 6 (1.2 mmol) in EtOH (10 mL) was refluxed in the presence of MoO2Cl2 (1 mol%). After the completion of the reaction, the contents were extracted with EtOAc (3 × 10 mL). The combined organic layers were washed with brine, water, dried over anhydrous Na2SO4. The solvent was evaporated and the crude product was purified by silica gel column chromatography (EtOAc
:
hexane) as an eluent to give desired product 7.
Acknowledgements
The authors thank Department of Pharmaceuticals, Ministry of Chemicals and Fertilizers (Govt. of India) for providing funds.
References
-
(a)
J. M. Tunney, in Comprehensive Coordination Chemistry II, ed. J. McMaster and C. D. Garner, Elsevier, Amsterdam, 2003, vol. 8, p. 459 Search PubMed;
(b) J. H. Enemark, J. J. A. Cooney, J.-J. Wang and R. H. Holm, Chem. Rev., 2004, 104, 1175 CrossRef CAS PubMed;
(c) C. J. Whiteoak, G. J. P. Britovsek, V. C. Gibson and A. J. P. White, Dalton Trans., 2009, 2337 RSC;
(d) A. L. Bingham, J. E. Drake, M. B. Hursthouse, M. E. Light, R. Kumar and R. Ratnani, Polyhedron, 2006, 25, 3238 CrossRef CAS;
(e) R. J. Butcher, B. R. Penfold and E. Sinn, J. Chem. Soc., Dalton Trans., 1979, 668 RSC.
-
(a)
E. R. Braithwaite, in Molybdenum an outline of its chemistry and uses, ed. J. Haber, Elsevier, Amsterdam, 1994 Search PubMed;
(b) E. R. Braithwaite and A. B. Greene, Chem. Ind., 1978, 46, 405 CAS;
(c) W. W. Williams and J. W. Conley, Ind. Eng. Chem., 1955, 47, 1507 CrossRef CAS;
(d) W. G. Huckle and E. Lalor, Ind. Eng. Chem., 1955, 47, 1501 CrossRef CAS.
-
(a) F. W. Moore, G. A. Tsigdinos and T. R. Weber, Polym. Sci. Technol., 1984, 26, 215 CAS;
(b)
U. C. Gupta, Molybdenum in agriculture, Cambridge University Press, Cambridge, 1997 Search PubMed.
-
(a) C.-T. Chen, J.-H. Kuo, V. D. Pawar, S. M. Yogesh, S.-S. Weng, C.-H. Ku and C.-Y. Liu, J. Org. Chem., 2005, 70, 1188 CrossRef CAS PubMed;
(b) H. K. Kadam, Synlett, 2014, 1793 CAS;
(c) K. Jeyakumar and D. K. Chand, J. Chem. Sci., 2009, 121, 111 CrossRef CAS.
-
(a) A. C. Fernandes, R. Fernandes, C. C. Romao and B. Royo, Chem. Commun., 2005, 213 RSC;
(b) R. G. De Noronha, P. J. Costa, C. C. Romao, M. J. Calhorda and A. C. Fernandes, Organometallics, 2009, 28, 6206 CrossRef CAS;
(c) A. C. Fernandes and C. C. Romao, Tetrahedron Lett., 2005, 46, 8881 CrossRef CAS;
(d) A. C. Fernandes and C. C. Romao, Tetrahedron, 2006, 62, 9650 CrossRef CAS;
(e) S. Maignien, S. Ait-Mohand and J. Muzart, Synlett, 1996, 439 CrossRef CAS.
-
(a) K. Jeyakumar and D. K. Chand, Synthesis, 2009, 306 CAS;
(b) C. Stock and R. Brückner, Adv. Synth. Catal., 2012, 354, 2309 CrossRef CAS;
(c) C. Stock and R. Brückner, Synlett, 2010, 2429 CAS.
-
(a) M. F. Moghaddam, H. Saeidian, Z. Mirjafary and A. Sadeghi, J. Iran. Chem. Soc., 2009, 6, 317 CrossRef;
(b) P. J. Edwards, B. Allart, M. J. I. Andrews, J. A. Clase and C. Menet, Curr. Opin. Drug Discovery Dev., 2006, 9, 425 CAS;
(c) A. Dömling and I. Ugi, Angew. Chem., Int. Ed., 2000, 39, 3168 CrossRef.
-
(a) S. Goldman and J. Stoltefuses, Angew. Chem., Int. Ed. Engl., 1991, 30, 1559 CrossRef;
(b) C. O. Kappe, Tetrahedron, 1993, 49, 6937 CrossRef CAS;
(c) J. P. Wan and Y. Liu, Synthesis, 2010, 3943 CrossRef CAS;
(d) C. O. Kappe, Acc. Chem. Res., 2000, 33, 879 CrossRef CAS PubMed;
(e) Suresh and J. S. Sandhu, ARKIVOC, 2012, 1, 66 Search PubMed.
-
(a) V. Klusa, Drugs Future, 1995, 20, 135 CrossRef;
(b) R. G. Bretzel, C. C. Bollen, E. Maeser and K. F. Federlin, Am. J. Kidney Dis., 1993, 21, 53 CrossRef CAS PubMed;
(c) R. Boer and V. Gekeler, Drugs Future, 1995, 20, 499 Search PubMed;
(d) H. L. Davis and T. E. Davis, Cancer Treat. Rep., 1979, 63, 809 CAS.
-
(a) R. Simsek, U. B. Ismailoglu, C. Safak and I. Sahin-Erdemli, Farmaco, 2000, 55, 665 CrossRef CAS PubMed;
(b) R. D. Larsen, E. G. Corley, A. O. King, J. D. Carrol, P. Davis, T. R. Verhoeven, P. J. Reider, M. Labelle, J. Y. Gauthier, Y. B. Xiang and R. Zamboni, J. Org. Chem., 1996, 61, 3398 CrossRef CAS;
(c) Y. L. Chen, K. C. Fang, J. Y. Sheu, S. L. Hsu and C. C. Tzeng, J. Med. Chem., 2001, 44, 2374 CrossRef CAS PubMed.
-
(a) G. Roma, M. D. Braccio, G. Grossi and M. Chia, Eur. J. Med. Chem., 2000, 35, 1021 CrossRef CAS PubMed;
(b) D. Doube, M. Bloun, C. Brideau, C. Chan, S. Desmarais, D. Eithier, J. P. Falgueyeret, R. W. Friesen, M. Girad, Y. Girad, J. Guay, P. Tagari and R. N. Yong, Bioorg. Med. Chem. Lett., 1998, 8, 1225 CrossRef;
(c) M. P. Maguire, K. R. Sheets, K. Mcvety, A. P. Spada and A. Ziberstein, J. Med. Chem., 1994, 37, 2129 CrossRef CAS PubMed.
- F. Vitorio, T. M. Pereira, R. N. Castro, G. P. Guedes, C. S. Graebinab and A. E. Kümmerle, New J. Chem., 2015, 39, 2323 RSC.
-
(a) A. Kumar, S. Sharma, V. D. Tripathi, R. A. Maurya, S. P. Srivastava, G. Bhatia, A. K. Tamrakar and A. K. Srivastava, Bioorg. Med. Chem., 2010, 18, 4138 CrossRef CAS PubMed;
(b) L. E. Hinkel, J. Chem. Soc. Trans., 1920, 117, 137 RSC;
(c) L. Saikia, D. Dutta and D. K. Dutta, Catal. Commun., 2012, 19, 1 CrossRef CAS;
(d) L.-M. Wang, J. Sheng, L. Zang, J.-W. Han, Z.-Y. Fan, H. Tian and C.-T. Qian, Tetrahedron, 2005, 61, 1539 CrossRef CAS.
-
(a) S. K. Kumar and K. N. Singh, J. Heterocycl. Chem., 2010, 47, 194 Search PubMed;
(b) M. Maheswara, V. Siddaiah, G. L. V. Damu and C. V. Rao, ARKIVOC, 2006, 2, 201 Search PubMed;
(c) M. Kidwai, R. Chauhan, D. Bhatnagar, A. K. Singh, B. Mishra and S. Dey, Monatsh. Chem., 2012, 143, 1675 CrossRef CAS;
(d) D. S. Raghuvanshi and K. N. Singh, Indian J. Chem., Sect. B: Org. Chem. Incl. Med. Chem., 2013, 52, 1218 Search PubMed.
-
(a) K. A. Undale, T. S. Shaikh, D. S. Gaikwad and D. M. Pore, C. R. Chim., 2011, 14, 511 CrossRef CAS;
(b) J. P. Nirmal, P. V. Dadhaniya, M. P. Patel and R. G. Patel, Indian J. Chem., Sect. B: Org. Chem. Incl. Med. Chem., 2010, 49, 587 Search PubMed;
(c) A. Rajendran, C. Karthikeyan and K. Rajathi, Int. J. ChemTech Res., 2011, 3, 810 CAS;
(d) E. Rajanarendar, M. N. Reddy and S. Raju, Indian J. Chem., Sect. B: Org. Chem. Incl. Med. Chem., 2011, 50, 751 Search PubMed.
-
(a) W. H. Correa and J. L. Scott, Green Chem., 2001, 3, 296 RSC;
(b) J. L. Donelson, R. A. Gibas and S. K. De, J. Mol. Catal. A: Chem., 2006, 256, 309 CrossRef CAS;
(c) A. Kumar and R. A. Maurya, Tetrahedron Lett., 2007, 48, 3887 CrossRef CAS;
(d) S. Ko and C.-F. Yao, Tetrahedron, 2006, 62, 7293 CrossRef CAS.
-
(a) M. M. Heravi, K. Bakhtiari, N. M. Javadi, F. F. Bamoharram, M. Saeedi and H. A. Oskooie, J. Mol. Catal. A: Chem., 2007, 264, 50 CrossRef CAS;
(b) J. C. Legeay, J. Y. Goujon, J. J. V. Eynde, L. Toupet and J. P. Bazureau, J. Comb. Chem., 2006, 8, 829 CrossRef CAS PubMed;
(c) S. Ko, M. N. V. Sastry, C. Lin and C.-F. Yao, Tetrahedron Lett., 2005, 46, 5771 CrossRef CAS.
-
(a) M. Tajbakhsh, H. Alinezhsd, M. Norouzi, S. Baghery and M. Akbari, J. Mol. Liq., 2013, 177, 44 CrossRef CAS;
(b) A. Kumar and R. A. Maurya, Tetrahedron, 2007, 63, 1946 CrossRef CAS;
(c) N. N. Karade, V. H. Budhewar, S. V. Shinde and W. N. Jadhav, Lett. Org. Chem., 2007, 4, 16 CrossRef CAS;
(d) G. Song and B. Wang, Synth. Commun., 2005, 35, 2875 CrossRef CAS;
(e) S. R. Cherkupally and R. Mekala, Chem. Pharm. Bull., 2008, 56, 1002 CrossRef CAS PubMed.
-
(a) X.-L. Zhang, S.-R. Sheng, X.-L. Lu and X.-L. Liu, ARKIVOC, 2007, 13, 79 Search PubMed;
(b) U. C. Rajesh, S. Manohar and D. S. Rawat, Adv. Synth. Catal., 2013, 355, 3170 CrossRef CAS;
(c) M. M. Heravi, M. Saeedi, N. Karimi, M. Zakeri, Y. S. Beheshtiha and A. Davoodnia, Synth. Commun., 2010, 40, 523 CrossRef CAS.
-
(a) C. O. Kappe, Tetrahedron, 1993, 49, 6937 CrossRef CAS;
(b) E. H. Hu, D. R. Sidler and U.-H. Dolling, J. Org. Chem., 1998, 63, 3454 CrossRef CAS;
(c) K. Folkers, H. J. Harwood and T. B. Johnson, J. Am. Chem. Soc., 1932, 54, 3751 CrossRef CAS.
- K. Azuma, S. Suzuki, S. Uchiyama, T. Kajiro, T. Santa and K. Imai, Photochem. Photobiol. Sci., 2003, 2, 443 CAS.
-
(a) V. Sharma, P. Kumar and D. Pathak, J. Heterocycl. Chem., 2010, 47, 491 CAS;
(b) R. Romagnoli, P. G. Baraldi, V. Remusat, M. D. Carrion, C. L. Cara, D. Preti, F. Fruttarolo, M. G. Pavani, M. A. Tabrizi, M. Tolomeo, S. Grimaudo, J. Balzarini, M. A. Jordan and E. Hamel, J. Med. Chem., 2006, 49, 6425 CrossRef CAS PubMed;
(c) S. Mallena, M. P. H. Lee, C. Bailly, S. Neidle, A. Kumar, D. W. Boykin and W. D. Wilson, J. Am.
Chem. Soc., 2004, 126, 13659 CAS;
(d) V. Kumar, K. Kaur, G. K. Gupta and A. K. Sharma, Eur. J. Med. Chem., 2013, 69, 735 CrossRef CAS PubMed.
Footnote |
† Electronic supplementary information (ESI) available. See DOI: 10.1039/c5nj02444g |
|
This journal is © The Royal Society of Chemistry and the Centre National de la Recherche Scientifique 2016 |