DOI:
10.1039/C5NJ01672J
(Paper)
New J. Chem., 2016,
40, 144-150
Aggregation-induced emission enhancement and living cell imaging of novel diarylanthracene conjugated dyes†
Received
(in Montpellier, France)
28th June 2015
, Accepted 16th October 2015
First published on 21st October 2015
Abstract
In this work, two novel diarylanthracene conjugated dyes, CzAn and BCzAn, which contain carbazolyl substituted triarylamine groups, were synthesized by the Ullmann reaction and characterized by 1H NMR, 13C NMR, and HRMS-MALDI-TOF. Both of the diarylanthracene conjugated dyes CzAn and BCzAn demonstrated aggregation-induced emission enhancement (AIEE) properties. CzAn and BCzAn emitted brightly in solid states with maximum emission wavelengths of 576 and 601 nm. BCzAn emitted weakly in THF solutions with a fluorescence quantum yield of 8%, became a strong emitter in the aggregate states with the fluorescence peak intensity increased in THF–water mixtures and DMF–glycerol mixtures rather than in pure solvents. Nanoparticles (Nps) were prepared from CzAn and BCzAn by a hydrophobic interaction with bovine serum albumin (BSA). The diameters of the AIEE dye based BSA nanoparticles were characterized by transmission electron microscopy (TEM) and their photophysical properties were also measured. These BSA nanoparticles showed high water dispersibility, stable uniform morphology (100–200 nm), strong fluorescence, low cytotoxicity and excellent biocompatibility, making them good optical materials for living HeLa cell imaging.
Introduction
The design and synthesis of high-efficiency organic conjugated molecules with aggregation-induced emission enhancement (AIEE) properties have attracted considerable attention.1 Since the first report by Tang,2 a great number of AIEE-active dyes have been synthesized and researched.3 The AIEE-active dyes have the general feature that they exhibit low or no fluorescence when freely dissolved in a solvent. However, upon addition of a solvent which reduces their solubility, the fluorescence intensity drastically increases as a result of the aggregate formation.4 Restricted intramolecular rotation (RIR) of molecules in the aggregate state has been suggested as the main cause of the AIEE phenomenon.5 Diarylanthracene derivatives are an important class of AIEE-active compounds with high fluorescence quantum yields and photostability.6 A great number of diarylanthracene derivatives have been synthesized and considered to be candidates for fluorescence sensors7 and electroluminescent devices.8 A series of AIEE-active pyridine-end-capped diarylanthracene derivatives which possess good two-photon absorption properties were reported by our group.9
Although diarylanthracene and other AIEE-active dyes have many advantages aforementioned, many of them and dyes only having aggregation-induced emission (AIE) properties cannot be introduced into biological applications such as cell imaging directly because of poor water-solubility and poor biocompatibility.10 Researchers come up with some methods to overcome these disadvantages, such as modifying AIE molecules with ions to improve their solubility in cell media. However, the electric charges of the highly concentrated ionized dyes may affect intracellular physiology and even kill the cells.11 Our group has reported a series of AIE dye doped silica nanoparticles with high water-dispersity and excellent photophysical properties, but they have poor degradability in biological tissues.12 Combining the AIEE/AIE dye with an amphiphilic polymer matrix to form AIEE/AIE dye based nanoparticles can provide us with a valuable method to overcome the challenging issue.13 The frequently-used polymer matrix included chitosan,14 poly(DL-lactide-co-glycolide) (PLGA),15 1,2-distearoyl-sn-glycero-3-phosphoethanolamine-N-(polyethylene glycol) (DSPE-PEG),16etc. AIEE/AIE dye based fluorescent nanoparticles were extensively explored for biosensing, bioimaging and disease treatment because of their distinctive features such as hydrophilicity, size tuning, low cytotoxicity and biocompatibility.17 Tang18 has reported AIE dye based nanoparticles with photodynamic activity and could be successfully used in image-guided photodynamic therapy. Lu19 has reported folate-functionalized AIE dye based nanoparticles by co-encapsulating two dyes acting as donor–acceptor to achieve fluorescence resonance energy transfer (FRET) and showed specific targeting effects for cancer cells. Zhang20 has developed a simple method by combining an AIE-active 9,10-distyrylanthracene and a non-ionic surfactant to achieve hydrophobic nanoparticles which showed novel AIE properties, excellent biocompatibility and were applied in cell imaging.
Based on the discussions above, we can conclude that the synthesis of novel high-efficiency AIEE dyes and the preparation of relevant nanoparticles for biological applications are still desirable. In this paper, two novel diarylanthracene conjugated dyes, CzAn and BCzAn, which contain carbazolyl substituted triarylamine groups, were synthesized by Ullmann reaction and characterized by 1H NMR, 13C NMR, and HRMS-MALDI-TOF. Chemical structures of CzAn and BCzAn are given in Scheme 1. Fluorescence spectra of the diarylanthracene conjugated dyes in the solid state and their AIEE properties in mixed solvents were measured. Bovine serum albumin (BSA) is a commercially available hydrophilic polymer with great biocompatibility.21 BSA nanoparticles based on AIEE dyes CzAn and BCzAn were successfully used in living HeLa cell imaging.
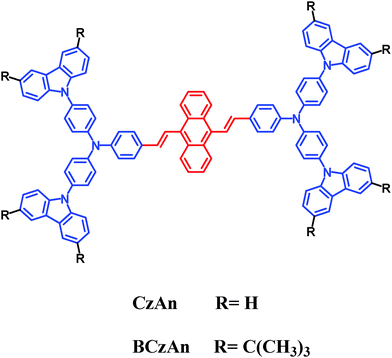 |
| Scheme 1 Chemical structures of diarylanthracene conjugated dyes CzAn and BCzAn. | |
Experimental procedure
Materials and instrument
Solvents were purified and dried using standard protocols. The original compound An-BIPAS was synthesized according to the literature reported by our laboratory.22 The intermediate 3,6-di-tert-butyl-9H-carbazole was synthesised according to the literature23 and identified by 1H NMR. All other chemical reagents were obtained commercially and were used as received without further purification.
Chemical structures were identified by high resolution mass spectrometry (HRMS) and nuclear magnetic resonance (NMR). HRMS was recorded on an Ultraflex-MALDI-TOF mass spectro-photometer. 1H NMR measurements were carried out using a Bruker 300 MHz spectrometer, with TMS (tetramethyl silane) as the internal standard. 13C NMR measurements were carried out using a Bruker 300 MHz spectrometer. UV-visible absorption spectra were recorded on a Shimadu UV-3600 spectrophotometer. Fluorescence spectra were recorded on a HORIBA FL-4 max spectrometer. The melting points were measured on a Microscopic Melting Point Meter X-4. Transmission electron microscopy (TEM) images were recorded on a Tecnai G20 microscope operated at 200 kV, the TEM specimens were made by placing a drop of the nanoparticle suspension on a carbon-coated copper grid. The cell imaging equipment used in our experiment was an Upright Fluorescence Microscope (Imager A1) provided by ZEISS, Germany.
Synthesis
Preparation of An-BIPAS.
The synthetic route of An-BIPAS was in accordance with the known literature procedure reported by our group.22
Preparation of CzAn.
A mixture of An-BIPAS (0.50 g, 0.41 mmol), carbazole (0.33 g, 1.97 mmol), 1,10-phenanthroline (0.12 g, 0.67 mmol), CuI (0.07 g, 0.37 mmol), K2CO3 (0.25 g, 1.81 mmol) in 15 mL of DMF was stirred at room temperature for 1 h. And then the mixture was stirred and heated to 160 °C for 72 h under a nitrogen atmosphere. After cooling, it was poured into 100 mL of water. The precipitate was filtered and dried. The crude product was purified by column chromatography on silica gel (petroleum ether/CH2Cl2) to afford the pure solid CzAn. Yield 60%. m.p. > 300 °C. 1HNMR (C6D6, 300 MHz) δ: 8.65–8.68 (m, 4H), 8.22 (d, 8H, J = 7.65 Hz), 7.95 (d, 2H, J = 16.41 Hz), 7.57 (dd, 12H, J = 8.10 Hz), 7.46–7.51 (m, 12H), 7.39–7.41 (m, 16H), 7.30–7.36 (m, 12H), 6.00 (d, 2H, J = 16.44 Hz); IR (KBr) ν: 3028, 1634, 1595, 1582, 1506, 1468, 1326, 1289, 971 cm−1; 13C NMR (C6D6, 300 MHz) δ 147.82, 147.16, 141.96, 141.81, 137.50, 133.87, 133.73, 133.41, 130.78, 127.35, 126.70, 126.59, 126.09, 125.82, 125.74, 125.61, 125.32, 125.05, 124.43, 124.30, 121.21, 121.10, 121.04, 120.85, 120.73, 110.47, 110.35. HRMS-MALDI-TOF calcd for C102H69N6 [M + H]+ C102H69N6 1377.5578, found 1377.5582.
Preparation of BCzAn.
A mixture of An-BIPAS (0.50 g, 0.41 mmol), 3,6-bis(tert-butyl)carbazole (0.55 g, 1.97 mmol), 1,10-phenanthroline (0.12 g, 0.67 mmol), CuI (0.07 g, 0.37 mmol), K2CO3 (0.25 g, 1.81 mmol) in 15 mL of DMF was stirred at room temperature for 1 h. And then the mixture was stirred and heated to 160 °C for 72 h under a nitrogen atmosphere. After cooling, it was poured into 100 mL of water. The precipitate was filtered and dried. The crude product was purified by column chromatography on silica gel (petroleum ether/CH2Cl2) to afford the pure solid BCzAn. Yield 53%. m.p. > 300 °C; 1H NMR (CDCl3, 300 MHz) δ: 8.50–8.53 (m, 4H), 8.23 (s, 8H), 8.01 (d, 2H, J = 16.38 Hz), 7.78 (d, 4H, J = 8.25 Hz), 7.61 (d, 12H, J = 8.64 Hz), 7.55 (s, 8H), 7.52 (d, 12H, J = 6.30 Hz), 7.43–7.49 (m, 8H), 7.05 (d, 2H, J = 16.32 Hz), 1.55 (s, 72H); 13C NMR (CDCl3, 300 MHz) δ: 149.92, 148.71, 145.45, 141.98, 139.43, 135.74, 132.27, 130.35, 129.18, 127.76, 127.17, 126.66, 126.17, 125.83, 118.89, 111.85, 37.38, 34.68; HRMS-MALDI-TOF calcd for C134H133N6 [M + H]+ 1826.0586 found 1826.0592.
Preparation of AIEE dye based BSA Nps.
BSA (0.1300 g) was dissolved in deionized water (50 mL) and a solution of THF (80 mL) containing 0.0013 g CzAn/BCzAn was added dropwise under sonication. Then 100 μL glutaraldehyde solution (25%) was added and the mixture was stirred for 4 h at room temperature. THF was removed by rotary evaporation and stored at 5 °C for later use.
Cell culture and cell imaging.
HeLa cells (human cancer cell lines) were grown in Dulbecco minimum essential media (DMEM) with 10% fetal bovine serum (FBS), 1% penicillin, and 1% amphotericin B. Before the treatment, the cells were plated in 24-well plates and incubated for 24 h. Then BSA nanoparticles were added at a concentration equal to 1.4 μg L−1 of pure dyes and incubated for 4 hours at 37 °C with 5% CO2. Then the cells were washed twice with PBS and imaged using a fluorescence microscope.
Cytotoxicity of AIEE dye based BSA Nps.
The cytotoxicity of the BSA Nps against HeLa cells was assessed by MTT assay. HeLa cells were seeded in 96-well microplates at a density of 105 cells mL−1 and cultured at 37 °C with 5% CO2 for 24 h. BSA Nps were added into a 96-well plate. The concentrations of BSA Nps were 0.7, 1.4, 2.8 μg L−1. After 24 h and 48 h, 10 μL of the MTT solution (5 mg mL−1 in PBS) was added into each sample well. After 4 h, DMSO (100 μL) was then added and the plate was lightly shaken for 10 min. The absorbance was measured at 570 nm. Every experiment was performed three times.
Results and discussion
Design and synthesis of the diarylanthracene conjugated dyes CzAn and BCzAn
The synthesis routes of the targeted diarylanthracene conjugated dyes CzAn and BCzAn were shown in Scheme 2. The original compound An-BIPAS was synthesized according to the literature reported by our group. Final compounds CzAn and BCzAn were synthesized by the Ullmann reaction with connecting carbazole and 3,6-di-tert-butyl-9H-carbazole to An-BIPAS at the sites of iodine atoms in 60% and 53% yield.
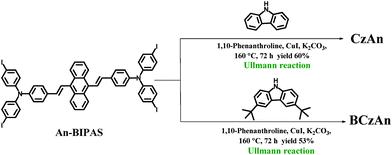 |
| Scheme 2 Synthesis of target diarylanthracene conjugated dyes CzAn and BCzAn. | |
The final compounds were confirmed by magnetic resonance (1H NMR and 13C NMR) and high resolution mass spectrometry (HRMS-MALDI-TOF). The data were found to be in good agreement with the proposed structures.
Fluorescence of the diarylanthracene conjugated dyes in solid states
The normalized fluorescence spectra of the two diarylanthracene conjugated dyes in solid states were shown in Fig. 1. Their maximum emission wavelengths were 576 nm and 601 nm, respectively. An obvious bathochromic shift was observed when tert-butyl was added to the carbazole. As shown in the insets of Fig. 1, under illumination with a 365 nm UV lamp, CzAn and BCzAn in the solid state showed bright emission from yellow to orange. As shown in Fig. 2, the CIE chromaticity coordinate values of CzAn and BCzAn were calculated to be (0.47, 0.46) and (0.58, 0.43).
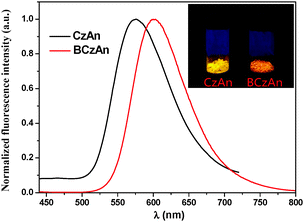 |
| Fig. 1 The normalized fluorescence spectra of CzAn and BCzAn in the solid state excited at 400 nm; the insets are photographs of CzAn and BCzAn in the solid state recorded under 365 nm UV irradiation. | |
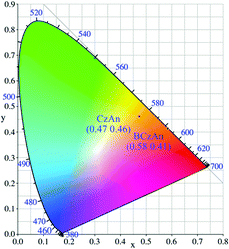 |
| Fig. 2 The CIE chromaticity coordinate of CzAn and BCzAn. | |
Aggregation-induced emission enhancement of the diarylanthracene conjugated dyes in mixed solvents
CzAn and BCzAn are soluble in common organic solvents, such as THF, DMF, dichloromethane and chloroform. However, water is a poor solvent. Considering that the good and poor solvents should be miscible and the potential biological application will be subsequently investigated, we used anhydrous THF as a good solvent and water as a poor solvent to investigate the aggregate optical properties of compounds. Adding water to a THF solution of the two dyes will cause the well-dissolved molecules to aggregate. The optical photographs of BCzAn in THF–water mixtures with the same concentration under illumination with a 365 nm UV lamp are shown in Fig. 3. The solution exhibited very yellow emission and an obvious enhancement of fluorescence intensity was observed when the water fraction increased. This phenomenon was consistent with that described by the aggregation-induced emission enhancement (AIEE) concept.
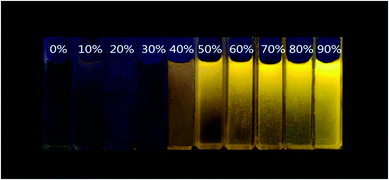 |
| Fig. 3 Optical photographs recorded under 365 nm UV irradiation for and BCzAn in THF–H2O mixtures with various volume fractions of water. | |
In order to further determine the AIEE properties of the diarylanthracene conjugated dyes, their fluorescence spectra in THF with different water fractions were measured. Fluorescence spectra of BCzAn in THF–water mixtures are shown in Fig. 4a (fluorescence spectra of CzAn in THF–water mixtures are provided in the ESI,† Fig. S8). The excitation wavelength was kept at 425 nm and the concentration was kept at 1 × 10−5 mol L−1. In pure THF, BCzAn exhibited very low fluorescence and fluorescence quantum yield was only 0.08. When the volume fraction of water (fw) was <40%, the fluorescence intensity of BCzAn increased slowly. With further addition of water (fw > 40%), the fluorescence intensity increased dramatically. When fw = 50%, the fluorescence quantum yield (Φf) was up to 0.16 (Φf was measured by using quinine in 0.5 N H2SO4 as a standard24). When fw = 90%, the fluorescence intensity reached the maximum and the peak value was found to get 25-fold of that in pure THF. Φf of BCzAn also reached the maximum value of 0.41 (Fig. 4b). Adding water to a THF solution will cause the well-dissolved molecules to aggregate. The results showed that the compound molecules might started to aggregate when the volume fraction of water reached 40%. The maximum emission wavelength of the compound was at 560 nm approximately and changed weakly in different water–THF mixtures.
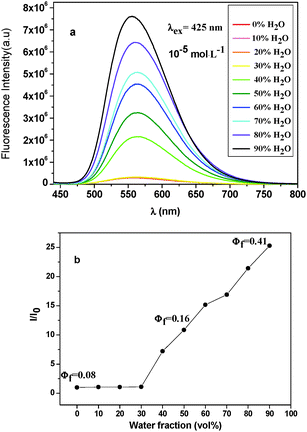 |
| Fig. 4 Fluorescence spectra of BCzAn (10−5 mol L−1) in THF with different water fractions excited at 425 nm (a); fluorescence peak intensities of BCzAn in THF (10−5 mol L−1) with different water fractions (I/I0, I0 = fluorescence intensity in pure THF solution, λex = 425 nm, Φf represents fluorescence quantum yield) (b). | |
Therefore, we can generally rationalize that the diarylanthracene conjugated dyes were characterized as AIEE-active.
To gain insight into the possible mechanism of AIEE, DMF–glycerol mixtures were selected. Glycerol is a common viscous solvent. Blending glycerol with DMF at different ratios can afford solvent mixtures with different viscosities.25 In pure DMF, the rotation of peripheral carbazolyl through a C–N single bond might consume the excited state energy through the nonradiative process and the chromophores emitted weakly (Fig. 5). Fluorescence spectra of BCzAn in DMF–glycerol mixtures are shown in Fig. 6a (fluorescence spectra of CzAn in DMF–glycerol mixtures are provided in the ESI,† Fig. S11). The excitation wavelength was kept at 425 nm and the concentration was kept at 1 × 10−5 mol L−1. The fluorescence intensity of BCzAn was increased by adding glycerol into its DMF solutions. When the volume fraction of glycerol (fg) was <30%, the intensity increase was due to a pure viscosity effect. When fg reached over 30%, the increase was due to the effects of both viscosity and aggregation. In mixtures with a high content of glycerol (fg = 60%), the fluorescence intensity reached the maximum and the peak value was found to get 17-fold of that in pure DMF (Fig. 6b). The increasing solvent viscosity of the mixed solvents can slow down intramolecular rotations, which blocks the nonradiative decay path and activates the radiative decay, hence changing the chromophores highly emissive. The viscosity effects can give us the general conclusion that the formation of aggregates restricting the intramolecular rotations (RIR) might be the cause of the enhancement of fluorescence intensity.
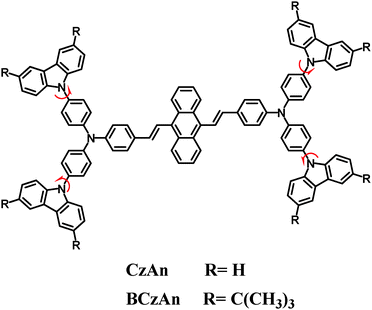 |
| Fig. 5 The rotation of peripheral carbazolyl through a C–N single bond. | |
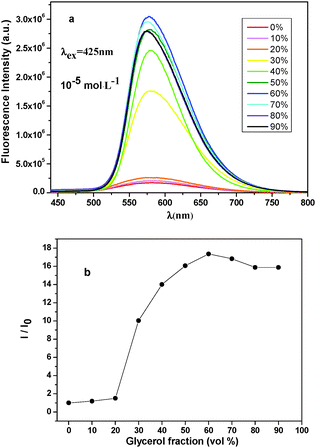 |
| Fig. 6 Fluorescence spectra of BCzAn at 10−5 mol L−1 in DMF with different glycerol fractions (λex = 425 nm) (a); fluorescence peak intensities of BCzAn in DMF (10−5 mol L−1) with different glycerol fractions (I/I0, I0 = fluorescence intensity in pure DMF solution, λex = 425 nm) (b). | |
The detailed photophysical properties of CzAn and BCzAn in solid states and in different mixed solvents are summarized in Table 1.
Table 1 Photophysical properties of CzAn and BCzAn in the solid state, in pure THF, in mixed THF and water, in pure DMF, in mixed DMF and glycerol
|
Solid |
THF |
f
w = 90% THF–water |
DMF |
f
g = 60% DMF–glycerol |
λ
em nm |
λ
abs nm |
λ
em nm/Φf |
λ
abs nm |
λ
em nm/Φf |
λ
em nm |
λ
em nm |
λ
abs UV-vis maximum absorption wavelength; λem maximum emission wavelength; fw water fraction of THF–water mixtures; fg glycerol fraction of DMF–glycerol mixtures; Φf fluorescence quantum yield. |
CzAn |
576 |
424 |
540/0.06 |
430 |
541/0.36 |
571 |
577 |
BCzAn |
601 |
420 |
564/0.08 |
430 |
559/0.41 |
583 |
577 |
BSA nanoparticles based on AIEE dyes CzAn, BCzAn and cell imaging
From the above discussions, it can be concluded that the diarylanthracene conjugated dyes CzAn and BCzAn have excellent AIEE properties. However, their biological applications may be limited because they have poor solubility in water. Nevertheless, bovine serum albumin (BSA) is a commercially available hydrophilic polymer with great biocompatibility. Therefore we used BSA as a matrix to prepare AIEE dye based nanoparticles (Nps), CzAn–BSA Nps and BCzAn–BSA Nps, and explore their biological applications.
The procedure for the preparation of the AIEE dye based BSA Nps is illustrated in Fig. 7. With the THF solution of CzAn/BCzAn gradually added into aqueous solution of BSA, the CzAn/BCzAn molecules gradually aggregate and entangle with the BSA chains. The AIEE dye based BSA Nps form instantly upon sonication. The BSA matrix was knitted together when glutaraldehyde (GA), a common cross-linker, was added. After the reaction finished, the THF is removed and the mixture returned to the aqueous system.26
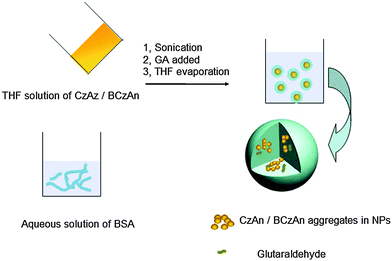 |
| Fig. 7 The preparation process and the model structure of the AIEE dye based BSA nanoparticles. | |
The UV-vis absorption and fluorescence spectra of AIEE dye based BSA Nps in aqueous solution were investigated to assess their photophysical properties. As seen in Fig. 8, the maximum absorption of CzAn–BSA and BCzAn–BSA Nps in aqueous solution was located at 425, 427 nm and their maximum emission wavelengths were located at 545, 557 nm, which were similar to the maximum absorption and emission wavelength of pure AIEE dyes in THF–water mixtures with a high water content. Meanwhile, the quantum yield of BSA Nps is 0.18 and 0.26, respectively. So it can be concluded that the BSA Nps inherit the excellent photophysical properties of AIEE dyes, CzAn and BCzAn.
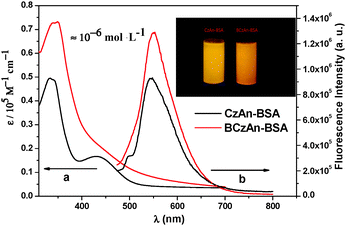 |
| Fig. 8 UV-vis absorbance spectra (a) and fluorescence spectra (b) of AIEE dye based BSA Nps in aqueous solution; the insets are optical photographs of Nps in aqueous solution recorded under 365 nm UV irradiation. | |
Transmission electron microscopy (TEM) images of the AIEE dye based BSA Nps are shown in Fig. 9. The images indicate that these Nps had a spherical shape and a smooth surface with an almost uniform size of around 150 nm.
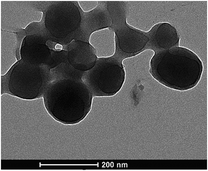 |
| Fig. 9 TEM images of AIEE dye based BSA Nps. | |
The biocompatibilities of BSA Nps with HeLa cells were also investigated using MTT assay. Fig. 10 shows the cell viability of HeLa cells after incubation with BCzAn–BSA Nps at concentrations of 0.7, 1.4, 2.8 μg L−1 for 24 and 48 h, respectively. The results show that no obvious cell viability decrease was found and further confirm that such BSA Nps are biocompatible with cells and promising for biomedical applications.
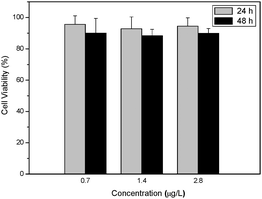 |
| Fig. 10 Cell viability of HeLa cells after incubation with BCzAn–BSA Nps at different concentrations for 24 and 48 h. | |
To exploit the potential biological applications of AIEE dye based BSA Nps, the cell imaging of AIEE dye based BSA Nps using an upright fluorescence microscope was examined. The fluorescence imaging picture of HeLa cells after incubation with BAnCz–BSA Nps is shown in Fig. 11 as an example, bright green fluorescence was observed when cells were incubated with 1.4 μg L−1 of nanoparticles for 4 h. The fluorescence covered the cells very well and BSA Nps showed an enhanced intracellular uptake and a high fluorescence contrast. These data indicate good photostability of the AIEE dye based BSA Nps in a biological environment, which meets the requirements for bioimaging applications.
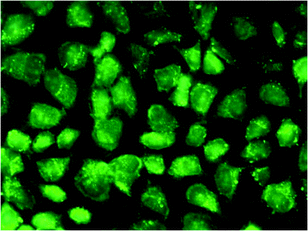 |
| Fig. 11 Fluorescence imaging picture of HeLa cells after incubation with BAnCz–BSA Nps. | |
Conclusions
In the present work, two novel diarylanthracene conjugated dyes containing carbazolyl substituted triarylamine groups, CzAn and BCzAn, were synthesized and demonstrated aggregation-induced emission enhancement (AIEE) properties. CzAn and BCzAn emitted brightly in the solid state with maximum emission wavelengths of 576 and 601 nm. BAnCz emitted weakly in THF solutions with a low fluorescence quantum yield of 0.08, became a strong emitter in the aggregate states. The fluorescence intensity and fluorescence quantum yield increased dramatically in mixtures with a high content of water compared with that in pure THF. The viscosity effects showed that the formation of aggregates restricting the intramolecular rotations (RIR) might be the cause of the enhancement of fluorescence intensity. Moreover, AIEE dye based BSA nanoparticles were prepared to explore the potential biological applications. These nanoparticles were demonstrated to exhibit stable uniform morphology, strong fluorescence, low cytotoxicity properties and were successfully used in HeLa cell imaging. Therefore, we believe that these BSA nanoparticles based on AIEE-active diarylanthracene conjugated dyes CzAn, BCzAn are excellent fluorescence probes and will have high potential for biological applications.
Acknowledgements
We thank the financial support from the National Natural Science Foundation of China (Grant numbers: 61178057). We thank Prof. S. Q. Liu, Dr H. N. Li for their kind help with the cell culture and the MTT assay.
Notes and references
- Y. N. Hong, J. W. Y. Lam and B. Z. Tang, Chem. Commun., 2009, 4332 RSC.
- J. D. Luo, Z. L. Xie, J. W. Y. Lam, L. Cheng, H. Y. Chen, C. F. Qiu, H. S. Kwok, X. W. Zhan, Y. Q. Liu, D. B. Zhu and B. Z. Tang, Chem. Commun., 2001, 1740 RSC.
-
(a) B. K. An, S. K. Kwon, S. D. Jung and S. Y. Park, J. Am. Chem. Soc., 2002, 124, 14410 CrossRef CAS PubMed;
(b) H. Li, Z. Chi, B. Xu, X. Zhang, X. Li, S. Liu, Y. Zhang and J. Xu, J. Mater. Chem., 2011, 21, 3760 RSC;
(c) Y. Zhang, J. Sun, G. Bian, Y. Chen, M. Ouyang, B. Hu and C. Zhang, Photochem. Photobiol. Sci., 2012, 11, 1414 RSC;
(d) L. Yang, J. Ye, L. Xu, X. Yang, W. Gong, Y. Lin and G. Ning, RSC Adv., 2012, 2, 11529 RSC;
(e) M. Baglan, S. Ozturk, B. Guer, K. Meral, U. Bozkaya, O. A. Bozdemir and S. Atilgan, RSC Adv., 2013, 3, 15866 RSC;
(f) Y. Wang, G. Lai, Z. Li, Y. Ma, Y. Shen and C. Wang, Tetrahedron, 2015, 71, 2761 CrossRef CAS;
(g) S. Sharma, C. P. Pradeep and A. Dhir, New J. Chem., 2015, 39, 1822 RSC.
- Y. Hong, J. W. Y. Lam and B. Z. Tang, Chem. Soc. Rev., 2011, 40, 5361 RSC.
-
(a) S. Kamino, Y. Horio, S. Komeda, K. Minoura, H. Ichikawa, J. Horigome, A. Tatsumi, S. Kaji, T. Yamaguchi, Y. Usami, S. Hirota, S. Enomoto and Y. Fujita, Chem. Commun., 2010, 46, 9013 RSC;
(b) X. Feng, B. Tong, J. Shen, J. Shi, T. Han, L. Chen, J. Zhi, P. Lu, Y. Ma and Y. Dong, J. Phys. Chem. B, 2010, 114, 16731 CrossRef CAS PubMed;
(c) S. Kaur, A. Gupta, V. Bhalla and M. Kumar, J. Mater. Chem. C, 2014, 2, 7356 RSC.
- B. Xu, J. Zhang, H. Fang, S. Ma, Q. Chen, H. Sun, C. Im and W. Tian, Polym. Chem., 2014, 5, 479 RSC.
-
(a) H. Li, X. Zhang, Z. Chi, B. Xu, W. Zhou, S. Liu, Y. Zhang and J. Xu, Org. Lett., 2011, 13, 556 CrossRef CAS PubMed;
(b) X. Zhang, Z. Chi, J. Zhang, H. Li, B. Xu, X. Li, S. Liu, Y. Zhang and J. Xu, J. Phys. Chem. B, 2011, 115, 7606 CrossRef CAS PubMed;
(c) X. Zhang, Z. Chi, X. Zhou, S. Liu, Y. Zhang and J. Xu, J. Phys. Chem. C, 2012, 116, 23629 CrossRef CAS;
(d) Y. Dong, B. Xu, J. Zhang, X. Tan, L. Wang, J. Chen, H. Lv, S. Wen, B. Li, L. Ye, B. Zou and W. Tian, Angew. Chem., Int. Ed., 2012, 51, 10782 CrossRef CAS PubMed.
-
(a) Y. C. Chang, S. C. Yeh, Y. H. Chen, C. T. Chen, R. H. Lee and R. J. Jeng, Dyes Pigm., 2013, 99, 577 CrossRef CAS;
(b) C. Chitpakdee, S. Namuangruk, P. Khongpracha, S. Jungsuttiwong, R. Tarsang, T. Sudyoadsuk and V. Promarak, Spectrochim. Acta, Part A, 2014, 125, 36 CrossRef CAS PubMed.
- C. Guan and Y. Qian, Chin. J. Org. Chem., 2014, 34, 537 CrossRef CAS.
-
(a) X. Zhang, X. Zhang, S. Wang, M. Liu, L. Tao and Y. Wei, Nanoscale, 2013, 5, 147 RSC;
(b) H. Lu, X. Zhao, W. Tian, Q. Wang and J. Shi, RSC Adv., 2014, 4, 18460 RSC.
- Y. Yu, C. Feng, Y. Hong, J. Liu, S. Chen, K. M. Ng, K. Q. Luo and B. Z. Tang, Adv. Mater., 2011, 23, 3298 CrossRef CAS PubMed.
- Y. Jin and Y. Qian, New J. Chem., 2015, 39, 2872 RSC.
-
(a) D. Ding, K. Li, B. Liu and B. Z. Tang, Acc. Chem. Res., 2013, 46, 2441 CrossRef CAS PubMed;
(b) X. Zhang, X. Zhang, L. Tao, Z. Chi, J. Xu and Y. Wei, J. Mater. Chem. B, 2014, 2, 4398 RSC;
(c) K. Li and B. Liu, Chem. Soc. Rev., 2014, 43, 6570 RSC;
(d) X. Zhang, K. Wang, M. Liu, X. Zhang, L. Tao, Y. Chen and Y. Wei, Nanoscale, 2015, 7, 11486 RSC.
-
(a) Z. Wang, S. Chen, J. W. Y. Lam, W. Qin, R. T. K. Kwok, N. Xie, Q. Hu and B. Z. Tang, J. Am. Chem. Soc., 2013, 135, 8238 CrossRef CAS PubMed;
(b) X. Zhang, X. Zhang, B. Yang, M. Liu, W. Liu, Y. Chen and Y. Wei, Polym. Chem., 2013, 4, 4317 RSC.
- J. Geng, K. Li, W. Qin, L. Ma, G. G. Gurzadyan, B. Z. Tang and B. Liu, Small, 2013, 9, 2012 CrossRef CAS PubMed.
-
(a) K. Li, Y. Jiang, D. Ding, X. Zhang, Y. Liu, J. Hua, S. S. Feng and B. Liu, Chem. Commun., 2011, 47, 7323 RSC;
(b) K. Li, W. Qin, D. Ding, N. Tomczak, J. Geng, R. Liu, J. Liu, X. Zhang, H. Liu, B. Liu and B. Z. Tang, Sci. Rep., 2013, 3, 1150 Search PubMed;
(c) W. Qin, K. Li, G. Feng, M. Li, Z. Yang, B. Liu and B. Z. Tang, Adv. Funct. Mater., 2014, 24, 635 CrossRef CAS;
(d) D. Ding, C. C. Goh, G. Feng, Z. Zhao, J. Liu, R. Liu, N. Tomczak, J. Geng, B. Z. Tang, L. G. Ng and B. Liu, Adv. Mater., 2013, 25, 6083 CrossRef CAS PubMed.
-
(a) X. Zhang, X. Zhang, B. Yang, Y. Zhang and Y. Wei, ACS Appl. Mater. Interfaces, 2014, 6, 3600 CrossRef CAS PubMed;
(b) M. Liu, X. Zhang, B. Yang, F. Deng, Z. Huang, Y. Yang, Z. Li, X. Zhang and Y. Wei, RSC Adv., 2014, 4, 35137 RSC;
(c) J. Geng, Z. Zhu, W. Qin, L. Ma, Y. Hu, G. G. Gurzadyan, B. Z. Tang and B. Liu, Nanoscale, 2014, 6, 939 RSC.
- Y. Yuan, G. Feng, W. Qin, B. Z. Tang and B. Liu, Chem. Commun., 2014, 50, 8757 RSC.
- H. Lu, X. Zhao, W. Tian, Q. Wang and J. Shi, RSC Adv., 2014, 4, 18460 RSC.
- X. Zhang, X. Zhang, S. Wang, M. Liu, L. Tao and Y. Wei, Nanoscale, 2013, 5, 147 RSC.
- M. Chen and M. Yin, Prog. Polym. Sci., 2014, 39, 365 CrossRef CAS.
- B. Wang and Y. Qian, New J. Chem., 2013, 37, 1402 RSC.
- J. Li, T. Zhang, Y. Liang and R. Yang, Adv. Funct. Mater., 2013, 23, 619 CrossRef CAS.
- A. M. Brouwe, Pure Appl. Chem., 2011, 83, 2213 Search PubMed.
- Q. Zeng, Z. Li, Y. Dong, C. A. Di, A. Qin, Y. Hong, L. Ji, Z. Zhu, C. K. W. Jim, G. Yu, Q. Li, Z. Li, Y. Liu, J. Qin and B. Z. Tang, Chem. Commun., 2007, 70 RSC.
- W. Qin, D. Ding, J. Liu, W. Z. Yuan, Y. Hu, B. Liu and B. Z. Tang, Adv. Funct. Mater., 2012, 22, 771 CrossRef CAS.
Footnote |
† Electronic supplementary information (ESI) available. See DOI: 10.1039/c5nj01672j |
|
This journal is © The Royal Society of Chemistry and the Centre National de la Recherche Scientifique 2016 |
Click here to see how this site uses Cookies. View our privacy policy here.