DOI:
10.1039/D1RA05819C
(Paper)
RSC Adv., 2021,
11, 38739-38749
Preparative separation of 1,1-diphenyl-2-picrylhydrazyl inhibitors originating from Saxifraga sinomontana employing medium-pressure liquid chromatography in combination with reversed-phase liquid chromatography†
Received
31st July 2021
, Accepted 19th October 2021
First published on 2nd December 2021
Abstract
Traditional Tibetan medicines elaborately document the health benefits of Saxifraga sinomontana. However, there have been limited reports on its chemical make-up, presumably because of the complicated separation and purification process. In this work, a methanolic extract of Saxifraga sinomontana was utilized for targeted separation of 4 key 1,1-diphenyl-2-picrylhydrazyl inhibitors employing the medium-pressure liquid chromatography, reversed-phase liquid chromatography in combination with on-line reversed-phase liquid chromatography-1,1-diphenyl-2-picrylhydrazyl detection. Pre-treatment of the sample was carried out by employing medium-pressure liquid chromatography using MCI GEL® CHP20P as the stationary phase, furnishing 2.4 g of fraction Fr3 and 3.4 g of fraction Fr4 (the percentage retrieval was 32.7%). The 1,1-diphenyl-2-picrylhydrazyl inhibitors contained in fractions Fr3 and Fr4 were subjected to additional separation using a C18 (ReproSil-Pur C18 AQ) column and yielded 106.2 mg of Fr3-1, 246.9 mg of Fr3-2, 248.5 mg of Fr4-1 and 41.8 mg of Fr4-2. The degree of purity, structures and 1,1-diphenyl-2-picrylhydrazyl inhibition activity of the isolated DPPH inhibitors were determined, and four 1,1-diphenyl-2-picrylhydrazyl inhibitors including two new diarylnonanoids (3-methoxy-4-hydroxyphenol-(6′-O-galloyl)-1-O-β-D-glucopyrano side with IC50 of 39.6 μM, 3,4,5-trimethoxyphenyl-(6′-O-galloyl)-1-O-β-D-glucopyranoside with IC50 of 46.9 μM, saximonsin A with IC50 of 11.4 μM, and saximonsin B with IC50 of 20.6 μM) were isolated with a percentage purity above 95%. The methodology thus evolved has good efficacy for preparatively isolating high-purity 1,1-diphenyl-2-picrylhydrazyl inhibitors from extracts of Saxifraga sinomontana and could be efficiently utilized for rapidly isolating 1,1-diphenyl-2-picrylhydrazyl inhibitors from other natural products.
1. Introduction
During ATP generation in the mitochondria, aerobic organisms give out free radicals, and at moderate levels, these species have advantageous functions in cell signaling and the immune system.1 Nevertheless, over-production of free radicals results in oxidative stress leading to cell damage and subsequently inflammation, aging, cancer, and cardiovascular and neurodegenerative diseases.2–4 Though organisms combat oxidative stress in several known ways, it has been shown that the effects of free radicals can be diminished by the addition of exogenous radical scavengers.5,6 The distinctive in vitro anti-oxidant potential of extracts of the Saxifraga plant prompted us to aim at isolating the chief free radical inhibitors and subsequently identification of them since the contemporary literature incorporated only brief reports on Saxifraga plants in terms of their chemical profile.7–11
Saxifraga sinomontana J. T. Pan & Gornall (S. sinomontana, family Saxifragaceae, also known as the “Sai Ren Jiao Mu”), is a perennial herb and extensively utilized in TTMs.12 Its advantages for treating hepatic and gall diseases, spleen and stomach diseases, and neuropathic diseases have been well known. However, there was only one detailed study on the chemical constituents from S. sinomontana and eighteen compounds (including phenylpropanoid glycoside, coumarins, phenolic acid, flavonoids and steroids) were identified. Previous study demonstrated the potential antioxidative activity of the isolated chemical constituents from the S. sinomontana,12 however, no reports on radical scavengers isolated from S. sinomontana exist to date.
The conventional method for purifying radical scavengers from NPs involves the isolation of compounds and subsequent testing of their antioxidative capacity.13–18 The mentioned process consumes a lot of time and a portion of antioxidative constituents can still be lost. The advancement in on-line HPLC-DPPH screening had a significant role in facilitating the screening and discovery of antioxidative molecular entities from NPs.19–24 An HPLC column is employed to isolate compounds in this technique and by dissolving DPPH in methanol or ethanol (that exhibits 517 nm as its absorbance maxima A517) enables their recognition as radical scavengers by undergoing a reaction with them following their exit from the column. The potential of individual compounds for scavenging radicals is proportional to the decline in A517. DPPH inhibitors can be efficiently identified in extracts of various NPs using this technique.25–30
Reversed-phase liquid chromatography (RPLC) is a technique of choice for isolating and purifying individual components from NPs.31–36 As a technique with high reproducibility and real-time tracking of the chemical constitution of the eluent, it can largely be automated.37,38 The major disadvantage associated with the use of RPLC is column contamination, hence it is impossible to inject crude extracts directly into the system. Prior to RPLC separation, some additional separation techniques must be employed to pre-treat the sample to improve the overall content of desired compounds and for the removal of interfering ingredients. A common pretreatment technique is medium-pressure liquid chromatography (MPLC). Different types of polyamide, gel, silica gel or MCI GEL® CHP20P are deployed in this technique as materials for column packing. The process of enriching the bioactive compounds originating from S. sinomontana relies on choosing the most effective technique in terms of pre-treatment, which eases the purification steps further. A frequently employed procedure for enriching bioactives from a variety of NPs is NPs MCI GEL® CHP20P MPLC, bearing the advantages of excellent reproducibility, on-line detection, and automatic control and was selected for pretreating our samples.39–41
As far as we are aware, there were no report on the chromatographic recognition, isolation and purification DPPH inhibitors from NPs except our laboratory reports. In our earlier work, we carried out the targeted separation of DPPH inhibitors originating from Saxifraga atrata by making use of three-steps of MPLC, RPLC, and HILIC regulated using an on-line HPLC-DPPH screening to preparative isolation of the DPPH inhibitors (11-O-galloylbergenin, ethyl gallate, isoquercitrin, and rutin).21 However, this work comprises an on-line RPLC-DPPH analysis in combination with two-steps of MCI GEL® CHP20P MPLC and RPLC to aid the exploration of powerful DPPH inhibitors from S. sinomontana. The present work is the first report on the use two-steps preparative separation strategy of MPLC and RPLC to recognize, isolate, and purify the DPPH inhibitors from NPs. A number of related works on the recognition and separation of DPPH inhibitors or radical scavengers from NPs could also benefit from the bioactivity-led recognition and isolation technique established and reported in this work. The bioactivity-guided recognition and targeted separation method can be transferrable to researches related to the separation of DPPH inhibitors from NPs.
2. Materials and methods
2.1 Apparatus and chemicals
A 5 mL manual injector, three prep-HPLC pumps (NP7000), an LC workstation, and a UV-Vis detector (NU3000) comprised the set up for preparative liquid chromatography from Hanbon Science & Technology Co., China. On-line RPLC-DPPH screening was carried out using Essentia LC-10AD and LC-16 instruments (Shimadzu Instruments Co. Japan). Two binary gradient pumps, an LC workstation, a fraction collector, and a UV/Vis detector comprise the key components of each HPLC. A reaction coil (18.0 m × 0.25 mm i.d.) with polyetheretherketone (PEEK) and a triple valve coupled the two devices. The Essentia LC-16A system was used to carry out the HPLC analysis and the LC-10AD system was employed to obtain the DPPH screening chromatogram. A Waters QDa ESI mass spectrometer (Waters Instruments Co. USA) was used to conduct ESI-MS analysis whereas a Q Exactive Orbitrap device (Thermo Fisher Scientific Inc., USA) was used to conduct HRESI-MS evaluation. The 600 MHz Bruker Avance was employed to obtain 1H and 13C NMR spectra (Bruker Instruments Co. Germany) using MeOH-d4 for the NMR solvent.
For separation, the purchase of MCI GEL® CHP20P (120 μm) was made from Mitsubishi Chemical Corporation (Japan). ReproSil-Pur C18 AQ (20 × 250 mm, 5 μm) preparative columns and ReproSil-Pur C18 AQ (4.6 × 250 mm, 5 μm) analytical columns were procured from Maisch Corporation (Germany).
Kelon Chemical Reagent Factory (Chengdu, China) was contacted for the purchase of acetonitrile (ACN) and analytical grade methanol (MeOH) used in preparative liquid chromatography. ACN (HPLC grade) was taken from Xinlanjing Chemical Industry (Yunnan, China). Moore water purification station (Chongqing, China) provided the HPLC grade water used in HPLC analysis and preparative liquid chromatography.
2.2 Preparation of sample, recognition of DPPH inhibitor and enrichment of active fraction
S. sinomontana, whole herb, was procured from Hainan County (4478 m, N 34°51′, 37.06′′, E 100°55′, 13.40′′), Qinghai, China in August 2018 and authenticated via Professor Qingbo Gao of the Northwest Institute of Plateau Biology, Chinese Academy of Sciences. A specimen was submitted to the Key Laboratory of Adaptation and Evolution of Plateau Biota, Chinese Academy of Sciences (No. Gao2018179).
S. sinomontana herbs were dried and ground into a powder. Methanol was then used to extract 170.0 g of the herb material twice (4.0 L and 3 d for individual run). Following filtration and concentration of the whole extract (8.0 L) at 40 °C under low pressure, DPPH inhibitors from S. sinomontana extract were screened by utilizing the on-line RPLC-DPPH system. ReproSil-Pur C18 AQ (4.6 × 250 mm, 5 μm) analytical column was used to accomplish the separation of the methanolic extract. ACN (mobile phase A) was increased linearly in water (mobile phase B) from 5–50% extending over 60 min thereby employing gradient elution and the temperature of the column was sustained at 25 °C. The eluent was allowed to flow at 1.0 mL min−1 whereas the volume of the injection was 5 μL and using the standard procedure a wavelength of 210 nm was used to monitor the absorbance. An ethanol (EtOH) solution of DPPH (25 μg mL−1) was applied for post-column derivatization maintaining a 0.8 mL min−1 rate of flow whereas the absorbance was at a wavelength of 517 nm.
50.0 g of dried polyamide (100–200 mesh) was mixed with approximately 30.0 mL of the concentrated methanolic extract which was rather incompletely dry and dried at 40 °C. A stationary phase comprising a pre-assembled 49 × 460 mm column filled using 1.2 L of MCI GEL® CHP20P was employed to load the resulting dry mixture (13.5 g) and a CH3OH/H2O mixture was used to elute it. Within a 90 min duration, the percentage of methanol underwent a linear increase from 0 to 100%. A 57.0 mL min−1 rate of flow was maintained, and the absorbance was tracked again at 210 nm. Following repeated separations for five cycles, the fractions Fr1 to Fr4 were gathered together, combined, and later concentrated to furnish sample fraction Fr1–Fr4.
2.3 Reversed-phase liquid chromatographic separation of DPPH inhibitors from the target fraction
Through MCI GEL® CHP20P MPLC pretreatment, 4 fractions namely Fr1, Fr2, Fr3, and Fr4 were obtained. RPLC analysis indicated that the fractions Fr3 and Fr4 contained DPPH inhibitors and were further separated by making use of a ReproSil-Pur C18 AQ (20 × 250 mm, 5 μm) preparative column. A 2.4 g sample of the fraction Fr3 was solubilized in an H2O (8.0 mL) and upon filtration via a 0.45 μm membrane, furnished a 300.0 mg mL−1 sample solution of Fr3. The stationary phase constituted a preparative column of ReproSil-Pur C18 AQ (20 × 250 mm, 5 μm) whereas the mobile phase was made up of ACN in H2O. Prior to separation, the system was allowed to equilibrate for 10.0 min. Until 60 min, an isocratic elution was carried out using 2% ACN. Each run was based on an injection of 1.0 mL of the Fr3 sample solution. The rate of flow of the eluent was constantly maintained at 19 mL min−1, and the process of elution was tracked at 210 nm. Redissolution of the 3.4 g fraction Fr4 sample dissolved in 12.0 mL of 80
:
20 v/v MeOH/H2O2 followed by filtration through a membrane with 0.45 μm, yielded a 366.7 mg mL−1 sample solution of Fr4. The column utilized was the preparative column of ReproSil-Pur C18 AQ (20 × 250 mm, 5 μm), and the mobile phase comprised ACN in H2O. Prior to separation, the system was set to equilibrate for 10.0 min. 15% ACN was applied for isocratic elution over 60 min. Each run involved an approximately 3.0 mL injection of the Fr4 sample solution. The rate of flow related to the eluent was maintained at 19 mL min−1 meanwhile tracking the elution at 210 nm.
2.4 Assessment of activity and purity of the obtained DPPH inhibitors
Evaluation of the degree of purity of the isolated compounds and estimation of their antioxidant potential was conducted using the on-line RPLC-DPPH system. A ReproSil-Pur C18 AQ (4.6 × 250 mm, 5 μm) analytical column was utilized to separate the DPPH inhibitors. Water and acetonitrile respectively were employed as the mobile phases. Linear gradient elution was based on 5–50% B for 60 min at a flow rate of 1.0 mL min−1. The volume of injection was 5 μL and the absorbance was tracked at 210 nm. The concentration of DPPH was 25 μg mL−1, whereas the eluent was allowed to flow at a rate of 0.8 mL min−1. At 517 nm, the UV-Vis detector-based chromatograms of the methanolic solution of DPPH were acquired.
2.5 mg of DPPH was dissolved in 100 mL of ethanol (25 μg mL−1) and stored in dark conditions (0–4 °C). Each isolated DPPH inhibitor (1.0 mg) was dissolved in ethanol (1.0 mL) and prepared into different concentrations (0–100 μg mL−1). 30 μL of each test DPPH inhibitors solution and 70 μL DPPH solution were added into the 96-well plates. All measurements were performed in triplicate. Then, the mixed solution was placed in darkness and incubated for 30 min. The ultraviolet absorbance of the mixture at 517 nm was determined and recorded as A. The scavenging rate of DPPH radical was calculated according to the following equation.
A0 was absorbance of the blank group (ethanol),
A1 was absorbance of the control group and
A was the absorbance of the sample (30 μL of each test DPPH inhibitors solution and 70 μL DPPH solution).
2.5 Statistical analysis
The statistical analysis was performed by applying SPSS version 20.0 software (SPSS, Chicago, IL, USA). All data given represented three repeat experiments, the concentrations 0–100 μg mL−1 were converted to μM and the nonlinear regression was used to calculate the concentration of the isolated DPPH inhibitors that caused 50% inhibition (IC50) of the scavenging activities. The DPPH scavenging activities curve was performed by the Prism 8.0 software.
3. Results and discussion
3.1 DPPH inhibitors recognition and MCI GEL® CHP20P medium-pressure liquid chromatography enrichment
MeOH was selected as the extraction solvent due to its excellent solubility and low-cost. Approximately 17.7 g (subtracted overdried 50.0 g polyamide from 67.7 g dried polyamide mixture) of crude extract was obtained from 170.0 g air-dried whole herb of S. sinomontana, producing an extraction yield of approximately 10.4%. Since few literature reports on DPPH inhibitors constituents of S. sinomontana are available, the methanolic concentrated extract (30.0 mL) of S. sinomontana was subjected to an on-line system of RPLC-DPPH provided with a ReproSil-Pur C18 AQ analytical column. Fig. 1B and C (Fig. 1A represents the schematic and actual diagrams of the on-line RPLC-DPPH system), respectively showed the separation and recognition chromatogram. As evident from Fig. 1B and C, the 4 major DPPH inhibitors peaks that in Fig. 1B had been marked with hearts were obtained between the retention times of 10 to 35 min. Keeping in mind that the extract was poorly soluble and complex, dried polyamide (50.0 g, 100–200 mesh) was supplemented to 30.0 mL of concentrated extract, mixed and dried. The obtained air-dried mixture comprised 17.7 g of whole herb extract of S. sinomontana.
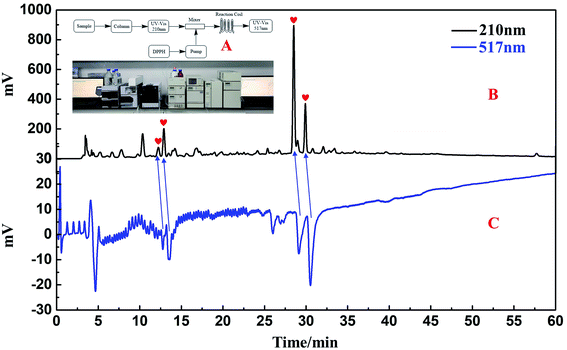 |
| Fig. 1 The schematic and actual diagrams of the on-line HPLC-DPPH apparatus (A), analytical chromatogram (B) on a ReproSil-Pur C18 AQ analytical column and DPPH inhibitory activity profile (C) of the S. sinomontana crude sample. | |
RPLC separation could be interfered by the presence of lipids and chlorophyll in plant extracts thereby contaminating the preparative column, so removal of these constituents prior to preparative separation was of utmost importance. MPLC, using a renewable MCI GEL® CHP20P sorbent-filled medium pressure column (49 × 460 mm) was utilized for the pretreating S. sinomontana extract. A novel dry extract-polyamide mixture (13.5 g) was added in a small MPLC column (sample loading column, 49 × 100 mm) and the preparative RPLC was connected to two MPLC columns (Fig. 2A). As shown in Fig. 2B four fractions (Fr1, Fr2, Fr3, and Fr4) were accumulated together following a single enrichment cycle using water–methanol based eluent.
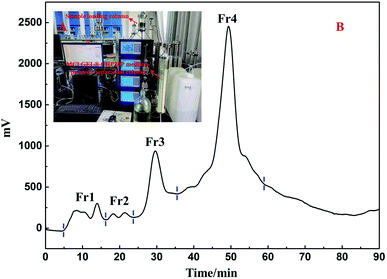 |
| Fig. 2 The actual MCI GEL® CHP20P MPLC system (A) and S. sinomontana extract-polyamide mixture separation chromatogram (B). | |
The collected fractions Fr1–Fr4 were combined after five repeated MCI GEL® CHP20P MPLC separations. They were concentrated and subjected to analysis by an analytical column of ReproSil-Pur C18 AQ, employing Essentia LC-16A system as in Fig. 1A. Fig. 3A–E presented the analysis chromatogram pertaining to the crude sample of S. sinomontana and the fractions Fr1–Fr4. Compared with Fig. 3A, D and E, the four key DPPH inhibitors from the crude sample of S. sinomontana were enriched efficiently in fractions Fr3 and Fr4 (the hearts in Fig. 3D and E), respectively. Additionally, peaks 1–4 (Fig. 3A and E) belonged to the 4 macroconstituent in the crude sample of S. sinomontana. Fractions Fr3 and Fr4 were gathered and after evaporation of the solvent, 2.4 g of fraction Fr3 and 3.4 g of fraction Fr4 were yielded respectively (32.7% recovery). The fractions Fr2 and Fr3 samples were severally solubilized in 8.0 mL of H2O and 12.0 mL MeOH/H2O2 mixture (80
:
20 v/v), and prepared for further chromatographic separation by passing through a 0.45 μm filter.
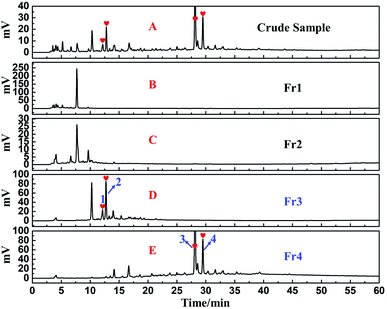 |
| Fig. 3 HPLC analysis of the S. sinomontana crude extract (A) and Fr1–Fr4 (B–E) on the ReproSil-Pur C18 AQ analytical column. | |
3.2 Reversed-phase liquid chromatographic separation of DPPH inhibitors from the fractions Fr3 and Fr4
Initiating with the chromatogram given in Fig. 3D and E, the separation conditions were further optimized on the same analytical column to enhance the resolution of the peaks as well as the efficiency of purification. Two well-resolved peaks (peaks 1 and 2, Fig. 4A correspond to DPPH negative peaks I to II, Fig. 4B) were observed by employing the on-line RPLC-DPPH set-up using the optimized conditions for fraction Fr3 on the ReproSil-Pur C18 AQ analytical column separation. Following linear amplification, 19.0 mL min−1 was maintained when preparatively separating Fr3 on the ReproSil-Pur C18 AQ preparative column, whereas the injection volume was kept at 1.0 mL. Fig. 4C showed the preparative chromatogram of the Fr3 fraction of S. sinomontana. A comparison of Fig. 4C and A makes it evident that active chromatographic peaks 1 and 2 were found to have nearly identical retention times on the ReproSil-Pur C18 AQ preparative column and the ReproSil-Pur C18 AQ analytical column. After eight repeated preparative separations, the fractions Fr3-1 and Fr3-2 were respectively gathered, the residues were dried after evaporating the solvent, resulting in 106.2 mg yield of Fr3-1 and 246.9 mg yield of Fr3-2; the recovery being 14.7%.
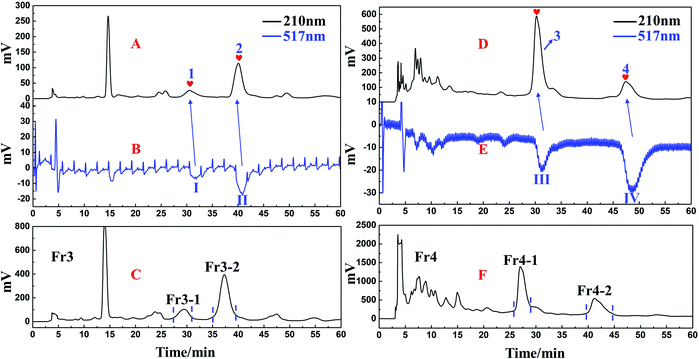 |
| Fig. 4 The optimized analytical chromatogram (A) and DPPH radical scavenging profile (B) of the S. sinomontana Fr3 sample on the ReproSil-Pur C18 AQ analytical column and preparative chromatogram (C) of the S. sinomontana Fr3 sample on the ReproSil-Pur C18 AQ preparative column; the optimized analytical chromatogram (D) and DPPH radical scavenging profile (E) of the S. sinomontana Fr4 sample on the ReproSil-Pur C18 AQ analytical column and preparative chromatogram (F) of the S. sinomontana Fr4 sample on the ReproSil-Pur C18 AQ preparative column. | |
For fraction Fr4, the optimized conditions of fraction Fr4 on the ReproSil-Pur C18 AQ analytical column separation were also conducted and two well-separated peaks (peaks 3 and 4, Fig. 4D that correspond to DPPH negative peaks III to IV, Fig. 4E) were clearly observed employing the on-line system of RPLC-DPPH. Subsequently, for the separation of Fr4, the linear amplification on the ReproSil-Pur C18 AQ preparative column, was used to calculate the flow rate (19.0 mL min−1) while the volume of the injection was maintained at 1.5 mL. Fig. 4F showed the preparative chromatogram of the S. sinomontana fraction Fr4. When compared with Fig. 4D and F, similar retention times were observed for active chromatographic peaks 3 and 4 on the preparative column of ReproSil-Pur C18 AQ (Fig. 4F) and analytical column of ReproSil-Pur C18 AQ (Fig. 4D). After eight repeated preparative separations, the fractions Fr4-1 and Fr4-2 were respectively obtained, and the residues were dried following evaporation of the solvent, giving 248.5 mg of Fr4-1 and 41.8 mg of Fr4-2 with a percentage retrieval of 8.5%.
3.3 Purity, structural characterization and activity of the isolated DPPH inhibitors
Isolated DPPH inhibitors Fr3-1, Fr3-2, Fr4-1 and Fr4-2 were re-evaluated for the degree of their purity and their activities by employing the on-line RPLC-DPPH system with a ReproSil-Pur C18 AQ analytical column. The four constituent compounds were found to have purities well above 95% as illustrated in Fig. 5A–H and the contents of the four DPPH inhibitors were 0.62‰ for Fr3-1, 1.45‰ for Fr3-2, 1.46‰ for Fr4-1 and 0.24‰ for Fr4-2, respectively. IR, UV, MS, NMR, and optical rotation spectra were acquired to explicate the detailed structural information pertaining to isolated DPPH inhibitors. A comparison of Fr3-1 and Fr3-2 was made with the already published literature reports. The details of MS data and NMR of Fr3-1 and Fr3-2 (given below, full spectra in ESI†) agreed respectively with the data for 3-methoxy-4-hydroxyphenol-(6′-O-galloyl)-1-O-β-D-glucopyranoside and 3,4,5-trimethoxyphenyl-(6′-O-galloyl)-1-O-β-D-glucopyranoside, (structures of Fr3-1 and Fr3-2 in Fig. 6). The structures of Fr4-1 and Fr4-2 were identified to two new diarylnonanoids NPs (named saximonsin A and saximonsin B, Fig. 6) based on their spectroscopic data.
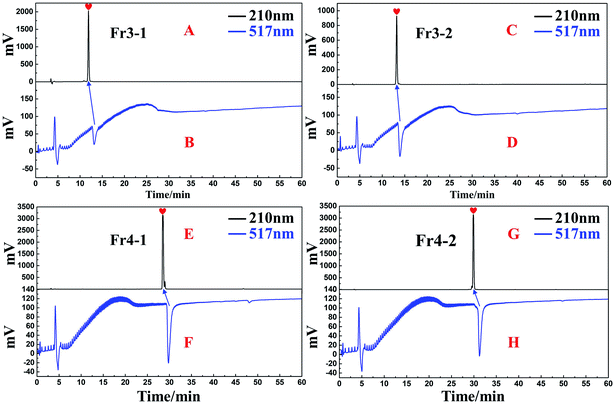 |
| Fig. 5 Purity and DPPH inhibitory activity of the target fraction activity verification chromatogram of the isolated Fr3-1 (A and B), Fr3-2 (C and D), Fr4-1 (E and F), and Fr4-2 (G and H) fractions on the ReproSil-Pur C18 AQ analytical column. | |
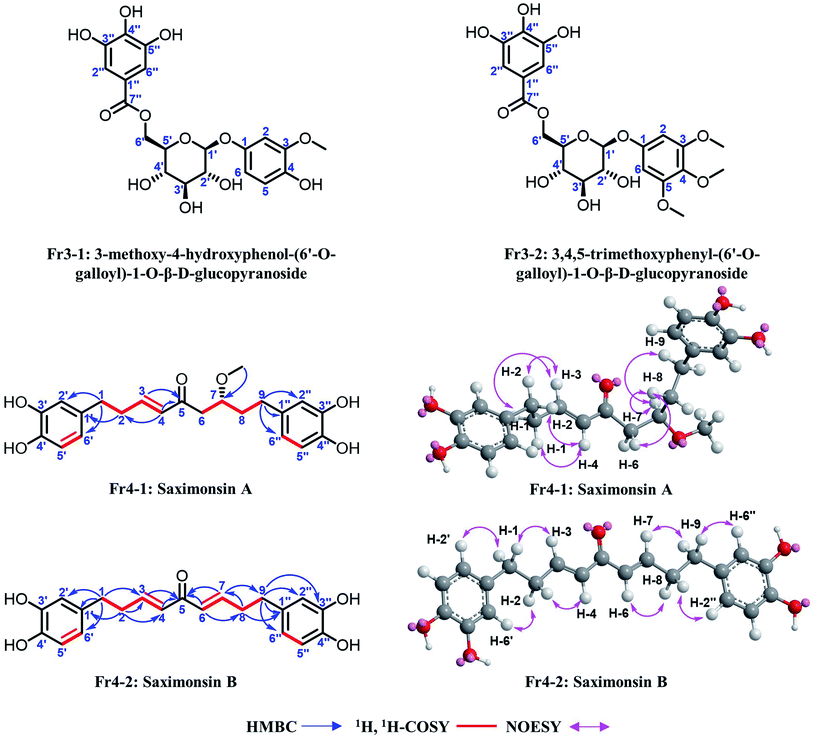 |
| Fig. 6 Chemical structures and the HMBC, 1H, 1H-COSY and NOESY correlations of the isolated DPPH inhibitors. | |
Fraction Fr3-1 (3-methoxy-4-hydroxyphenol-(6′-O-galloyl)-1-O-β-D-glucopyranoside, ESI-MS m/z 477.26, [M + Na]+, 453.34, [M − H]−, calc. for C20H22O12 m/z 454.1111): 1H NMR (600 MHz, MeOH-d4) 7.09 (2H, s, H-2′′, 6′′), 6.93 (1H, d, J = 8.7 Hz, H-5), 6.42 (1H, d, J = 2.8 Hz, H-2), 6.17 (1H, dd, J = 8.7, 2.8 Hz, H-6), 4.66 (1H, d, J = 7.5 Hz, H-1′), 4.53 (1H, dd, J = 11.8, 2.1 Hz, H-b), 4.42 (1H, dd, J = 11.8, 6.4 Hz, H-a), 3.77 (3H, s, –OCH3), 3.63 (1H, m, H-5′), 3.46 (3H, m, H-2′, 3′, 4′); 13C NMR (151 MHz, MeOH-d4) 168.3 (C-7′′), 154.9 (C-4), 152.0 (C-3), 146.5 (C-3′′, 5′′), 141.0 (C-1), 139.9 (C-4′′), 121.4 (C-1′′), 120.5 (C-5), 110.3 (C-2′′, 6′′), 107.7 (C-6), 104.5 (C-1′), 101.8 (C-2), 77.8 (C-3′), 75.6 (C-5′), 75.0 (C-2′), 71.7 (C-4′), 64.7 (C-6′), 56.5 (–OCH3). The data correspond to 3-methoxy-4-hydroxyphenol-(6′-O-galloyl)-1-O-β-D-glucopyranoside.42
Fraction Fr3-2 (3,4,5-trimethoxyphenyl-(6′-O-galloyl)-1-O-β-D-glucopyranoside, ESI-MS m/z 521.28, [M + Na]+, 497.35, [M − H]−, calc. for C22H26O13 m/z 498.1373): 1H NMR (600 MHz, MeOH-d4) 7.04 (2H, s, H-2′′, 6′′), 6.93 (1H, s, H-2, 6), 4.81 (1H, d, J = 7.6 Hz, H-1′), 4.61 (1H, dd, J = 11.9, 1.9 Hz, H-b), 4.41 (1H, dd, J = 11.9, 6.7 Hz, H-a), 3.75 (1H, m, H-5′), 3.66 (3H, brs, 4-OCH3), 3.65 (6H, brs, 3, 5-OCH3), 3.45 (3H, m, H-2′, 3′, 4′); 13C NMR (151 MHz, MeOH-d4) 168.3 (C-7′′), 155.9 (C-1), 154.8 (C-3, 5), 146.6 (C-3′′, 5′′), 139.9 (C-4′′), 134.7 (C-4), 121.4 (C-1′′), 110.1 (C-2′′, 6′′), 103.3 (C-1′), 96.3 (C-2, 6), 77.7 (C-3′), 75.8 (C-5′), 74.9 (C-2′), 71.8 (C-4′), 65.1 (C-6′), 61.2 (4-OCH3), 56.5 (3, 5-OCH3). The data correspond to 3,4,5-trimethoxyphenyl-(6′-O-galloyl)-1-O-β-D-glucopyranoside.43
Fraction Fr4-1 (saximonsin A, brown solid, [α]25D +80.1 (c 0.02, CHCl3); UV (MeOH, λmax, nm) (log
ε): 282.5 (3.77); IR (KBr, νmax, cm−1): 3429, 2959, 2918, 2851, 1736, 1617, 1261, 1098, 1030, 804; HRESI-MS m/z 387.1796, [M + H]+, calc. for C22H27O6 m/z 387.1802, [M + H]+). The 1H NMR spectrum of fraction Fr4-1 revealed that the compound contained two 1,3,4-trisubstituted benzene rings [6.66 (1H, d, J = 8.0 Hz, H-5′), 6.62 (1H, d, J = 2.0 Hz, H-2′), 6.49 (1H, dd, J = 8.0, 2.0 Hz, H-6′) and 6.66 (1H, d, J = 8.0 Hz, H-5′′), 6.60 (1H, d, J = 2.0 Hz, H-2′′), 6.48 (1H, dd, J = 8.0, 2.0 Hz, H-6′′)], a pair of trans olefinic protons [6.87 (1H, dt, J = 15.9, 7.0 Hz) and 6.07 (1H, br d, J = 15.9 Hz)], 11 aliphatic proton signals distributed in the high field range of 1.65–3.65 and a methoxy group [3.27 (3H, s)]. The 13C NMR and DEPT spectra of fraction Fr4-1 showed a total of 22 carbon resonances containing; seven quaternary carbons atoms at δC 201.6, 146.2, 146.2, 144.6, 144.3, 134.8, 133.8 ppm; nine methine carbons at δC 149.7, 132.0, 120.7, 120.6, 116.5, 116.5, 116.3, 116.3, 78.5 ppm; five methylene carbons at δC 45.3, 37.2, 35.6, 34.8, 31.7 ppm and one methoxy carbon at δC 57.2 ppm. The nine methine aromatic or olefinic carbons contained two sets of 2,5,6 positional CH signals (6 CH groups, δC 120.7, 120.6, 116.5, 116.5, 116.3, 116.3 ppm) of 1,3,4-trisubstituted benzene rings, which was consistent with information of the 1H NMR (two 1,3,4-trisubstituted benzene rings). The rest of the CH (δC 149.7, 132.0 ppm) and 78.5 ppm were corresponded with the double bond group and oxygenated methine carbon, respectively. Combining with the carbonyl carbon at δC 201.6 and five aliphatic methylene carbons, we speculated a long straight chain with nine carbons was existed in the structure. After searching the relevant literatures, the 1H and 13C chemical shifts were similar to those of C9 chain of ericanone,44 which was isolated from Erica cinerea except the dehydration synthesis of 3, 4 carbons. The methoxy group was positioned at C-7 on the basis of the HMBC correlations from the methoxy proton signal to C-7. Therefore, the structure of fraction Fr4-1 was established as (E)-1,9-bis(3,4-dihydroxyphenyl)-7-methoxynon-3-en-5-one (Fig. 6) and named saximonsin A (diarylnonanoid). Its signal assignment of 1H and 13C chemical shifts were in Table 1 and the HMBC, 1H, 1H-COSY and NOESY correlations of fraction Fr4-1 were depicted in the Fig. 6.
Table 1 The NMR data for fraction Fr4-1 and Fr4-2 in CD3OD (δH, 3.30 ppm; δC, 49.0 ppm)
No. |
Fr4-1 |
Fr4-2 |
1H NMR |
13C NMR |
1H NMR |
13C NMR |
1 |
2.63 (2H, t, 7.7) |
34.8 |
2.64 (2H, t, 7.7) |
34.9 |
2 |
2.48 (2H, br q-like, 7.3) |
35.6 |
2.49 (2H, br q-like, 7.3) |
35.8 |
3 |
6.87 (1H, dt, 15.9, 7.0) |
149.7 |
6.88 (1H, dt, 15.7, 7.0) |
149.5 |
4 |
6.07 (1H, br d, 15.9) |
132.0 |
6.31 (1H, br d, 15.7) |
129.9 |
5 |
— |
201.6 |
— |
192.2 |
6 |
2.61 (1H, dd, 15.3, 5.3) |
45.3 |
6.31 (1H, br d, 15.7) |
129.9 |
2.80 (1H, dd, 15.3, 7.2) |
7 |
3.63 (1H, m) |
78.5 |
6.88 (1H, dt, 15.7, 7.0) |
149.5 |
8 |
1.65–1.78 (2H, m) |
37.2 |
2.49 (2H, br q-like, 7.3) |
35.8 |
9 |
2.45–2.56 (2H, m) |
31.7 |
2.64 (2H, t, 7.7) |
34.9 |
1′ |
— |
133.8 |
— |
133.9 |
2′ |
6.62 (1H, d, 2.0) |
116.5 |
6.62 (1H, d, 2.0) |
116.5 |
3′ |
— |
146.2 |
— |
146.2 |
4′ |
— |
144.6 |
— |
144.5 |
5′ |
6.66 (1H, d, 8.0) |
116.3 |
6.66 (1H, d, 8.0) |
116.3 |
6′ |
6.49 (1H, dd, 8.0, 2.0) |
120.7 |
6.50 (1H, dd, 8.0, 2.0) |
120.7 |
1′′ |
— |
134.8 |
— |
133.9 |
2′′ |
6.60 (1H, d, 2.0) |
116.5 |
6.62 (1H, d, 2.0) |
116.5 |
3′′ |
— |
146.2 |
— |
146.2 |
4′′ |
— |
144.3 |
— |
144.5 |
5′′ |
6.66 (1H, d, 8.0) |
116.3 |
6.66 (1H, d, 8.0) |
116.3 |
6′′ |
6.48 (1H, dd, 8.0, 2.0) |
120.6 |
6.50 (1H, dd, 8.0, 2.0) |
120.7 |
OCH3 |
3.27 (3H, s) |
57.2 |
— |
— |
Fraction Fr4-2 (saximonsin B, brown solid; UV (MeOH, λmax, nm) (log
ε): 279.5 (3.81), 375.0 (3.30); IR (KBr, νmax, cm−1): 3427, 2919, 2851, 1631, 1603, 1261, 1114, 805; HRESI-MS m/z 355.1536, [M + H]+, calc. for C21H23O5 m/z 355.1540, [M + H]+). The 1H NMR spectrum of fraction Fr4-2 showed that the structure also contained two 1,3,4-trisubstituted benzene ring moieties, two trans olefinic protons [6.88 (1H, dt, J = 15.7, 7.0 Hz) and 6.31 (1H, br d, J = 15.7 Hz)], two methylene signals (2.64 (2H, t, J = 7.7 Hz) and 2.49 (2H, br q-like, J = 7.3 Hz)), which indicated the structure was symmetric. The 13C NMR and DEPT spectra of fraction Fr4-2 also proved the symmetry of the structure. Considering the NMR signal characteristics of fractions Fr4-2 and Fr4-1, the structure of fraction Fr4-2 was established as (3E,6E)-1,9-bis (3,4-dihydroxyphenyl)nona-3,6-dien-5-one named saximonsin B (diarylnonanoid). Its signal assignment of 1H and 13C chemical shifts were in Table 1 and the HMBC, 1H, 1H-COSY and NOESY correlations of fraction Fr4-2 were depicted in the Fig. 6.
In order to evaluated the in vitro DPPH scavenging activities of the isolated compounds Fr3-1, Fr3-2, Fr4-1, Fr4-2, DPPH free radical scavenging assay were performed in this study with the modified method based on Zhao et al.45 and Damiano et al.46 with some modifications. As shown in Fig. 7, the four isolated DPPH inhibitors displayed strong antioxidant activity and the IC50 value were 39.6 μM, 46.9 μM, 11.4 μM and 20.6 μM for Fr3-1, Fr3-2, Fr4-1 and Fr4-2 respectively. Compared with the structures of Fr3-1 and Fr3-2, which had similar parent nucleus except the different substituent groups of C4 and C5, the hydroxyl substituted C4 and nonsubstituted C5 (Fr3-1) had lower IC50 value (39.6 μM) compared with methoxy-substituted C4 and C5 (Fr3-2, IC50 value, 46.9 μM). In addition, the structures of Fr4-1 and Fr4-2, the methoxy-substituted C7 diarylnonanoid (saximonsin A) had lower IC50 value (11.4 μM) compared with dehydrogenize C6 and C7 diarylnonanoid (saximonsin B) with IC50 value of 20.6 μM.
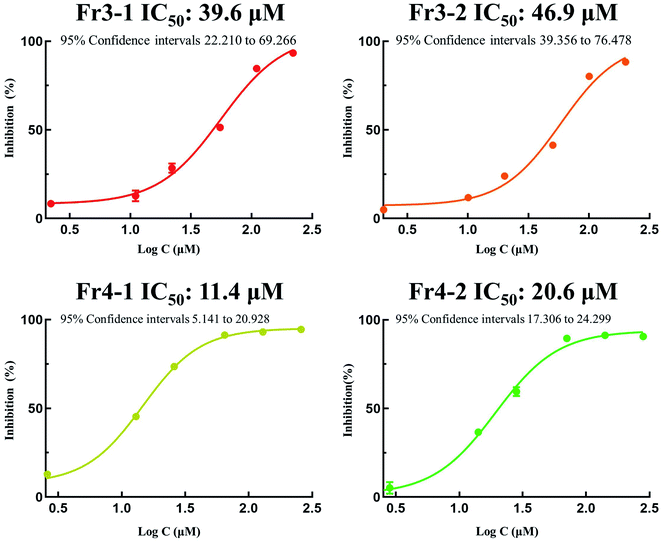 |
| Fig. 7 DPPH inhibition activities of the isolated DPPH inhibitors (Fr3-1, Fr3-2, Fr4-1 and Fr4-2) at different concentrations (μM) were fitted with a logistic function to count the IC50 value. | |
4. Conclusion
The current work established an efficient and rapid technique comprising MPLC and RPLC supplemented with on-line RPLC-DPPH assay for screening, isolating, and finally purifying DPPH inhibitors from S. sinomontana. The methanolic extract of the S. sinomontana gave a total of four DPPH inhibitors which were identified as per the standard procedure. To isolate the targeted fractions, the extract was pre-treated utilizing an MCI GEL® CHP20P sorbent, furnishing 2.4 g and 3.4 g of fraction Fr3 and fraction Fr4 respectively (32.7% recovery). Additional separation of the fractions Fr3 and Fr4 was carried out using isocratic elution on the preparative column of ReproSil-Pur C18 AQ, whereby 106.2 mg of Fr3-1 and 246.9 mg of Fr3-2 (14.7% recovery) were isolated from fraction Fr3 and 248.5 mg of Fr4-1 and 41.8 mg of Fr4-2 (8.5% recovery) were isolated from fraction Fr4. The radical scavenging activity and purity were thoroughly re-evaluated and all four fractions (Fr3-1, Fr3-2, Fr4-1, and Fr4-2) were found to be active towards DPPH inhibition, the purity was determined to be >95% and the IC50 value were 39.6 μM, 46.9 μM, 11.4 μM and 20.6 μM for Fr3-1, Fr3-2, Fr4-1 and Fr4-2 respectively. The outcome illustrated that the process of screening, isolating and purifying different NPs-based antioxidant molecules (including new antioxidative diarylnonanoids), can potentially benefit from the use of reported methodology. These results demonstrate that the reported methodology can be used for screening, isolation and purification of potent DPPH inhibitors from different natural products.
Abbreviations
TTMs | Traditional Tibetan medicines |
NPs | Natural products |
DPPH | 1,1-Diphenyl-2-picrylhydrazyl |
S. sinomontana | Saxifraga sinomontana |
MPLC | Medium-pressure liquid chromatography |
RPLC | Reversed-phase liquid chromatography |
Author contributions
Jun Dang: methodology, structural identification, data curation, manuscript development, review, editing and funding support; Jinbin Ma: methodology, data curation; Yangzom Dawa: methodology, data curation; Chuang Liu: methodology, data curation; Tengfei Ji: methodology, data curation, structural identification, manuscript development, review, and editing; Qilan Wang: data curation, funding support, manuscript development, review, and editing.
Conflicts of interest
The authors confirm having no known personal relationships or competing for financial interests that can impact the work reported in this paper.
Acknowledgements
This work capitalized on the support of the International Cooperation Project of Qinghai Province (2019-HZ-805), the Joint Research Project of Three-River Headwaters National Park, Chinese Academy of Sciences and The People's Government of Qinghai Province (LHZX-2020-09) and the Applied Basic Research Project of Qinghai Province (2020-ZJ-706).
References
- M. Valko, D. Leibfritz, J. Moncol, M. T. D. Cronin, M. Mazur and J. Telser, Free radicals and antioxidants in normal physiological functions and human disease, Int. J. Biochem. Cell Biol., 2007, 39, 44–84 CrossRef CAS PubMed.
- P. Newsholme, V. F. Cruzat, K. N. Keane, R. Carlessi and P. I. H. de Bittencourt, Molecular mechanisms of ROS production and oxidative stress in diabetes, Biochem. J., 2016, 473, 4527–4550 CrossRef CAS PubMed.
- L. Rochette, J. Lorin, M. Zeller, J. C. Guilland, L. Lorgis, Y. Cottin and C. Vergely, Nitric oxide synthase inhibition and oxidative stress in cardiovascular diseases: possible therapeutic targets?, Pharmacol. Ther., 2013, 140, 239–257 CrossRef CAS PubMed.
- M. Valko, C. J. Rhodes, J. Moncol, M. Izakovic and M. Mazur, Free radicals, metals and antioxidants in oxidative stress-induced cancer, Chem.-Biol. Interact., 2006, 160, 1–40 CrossRef CAS PubMed.
- J. K. Willcox, S. L. Ash and G. L. Catignani, Antioxidants and prevention of chronic disease, Crit. Rev. Food Sci. Nutr., 2004, 44, 275–295 CrossRef CAS PubMed.
- H. C. Wei, X. S. Zhang, J. F. Zhao, Z. Y. Wang, D. Bickers and M. Lebwohl, Scavenging of hydrogen peroxide and inhibition of ultraviolet light-induced oxidative DNA damage by aqueous extracts from green and black teas, Free Radical Biol. Med., 1999, 26, 1427–1435 CrossRef CAS PubMed.
- G. Ksouda, M. Hajji, S. Sellimi, F. Merlier, A. Falcimaigne-Cordin, M. Nasri and B. Thomasset, A systematic comparison of 25 Tunisian plant species based on oil and phenolic contents, fatty acid composition and antioxidant activity, Ind. Crops Prod., 2018, 123, 768–778 CrossRef CAS.
- D. Badral, B. Odonbayar, T. Murata, T. Munkhjargal, B. Tuvshintulga, I. Igarashi, K. Suganuma, N. Inoue, A. H. Brantner, G. Odontuya, K. Sasaki and J. Batkhuu, Flavonoid and galloyl glycosides isolated from Saxifraga spinulosa and their antioxidative and inhibitory activities against species that cause piroplasmosis, J. Nat. Prod., 2017, 80, 2416–2423 CrossRef CAS PubMed.
- J. Dang, Y. D. Tao, Y. Shao, L. J. Mei, L. Zhang and Q. L. Wang, Antioxidative extracts and phenols isolated from Qinghai-Tibet Plateau medicinal plant Saxifraga tangutica, Engl., Ind. Crops Prod., 2015, 78, 13–18 CrossRef CAS.
- K. S. Bodakhe, K. P. Namdeo, K. C. Patra, L. Machwal and S. K. Pareta, A polyherbal formulation attenuates hyperoxaluria-induced oxidative stress and prevents subsequent deposition of calcium oxalate crystals and renal cell injury in rat kidneys, Chin. J. Nat. Med., 2013, 11, 466–471 CAS.
- I. Chevalley, A. Marston and K. Hostettmann, A new gallic acid fructose ester from Saxifraga stellaris, Phytochemistry, 1999, 50, 151–154 CrossRef CAS.
- J. X. Liu, D. L. Di and Y. P. Shi, Diversity of chemical constituents from Saxifraga montana H, J. Chin. Chem. Soc., 2008, 55, 863–870 CrossRef CAS.
- Z. N. Yang, B. J. Su, Y. Q. Wang, H. B. Liao, Z. F. Chen and D. Liang, Isolation, absolute configuration, and biological activities of chebulic acid and brevifolincarboxylic acid derivatives from Euphorbia hirta, J. Nat. Prod., 2020, 83, 985–995 CrossRef CAS PubMed.
- C. Bustos-Brito, P. Joseph-Nathan, E. Burgueno-Tapia, D. Martinez-Otero, A. Nieto-Camacho, F. Calzada, L. Yepez-Mulia, B. Esquivel and L. Quijiano, Structure and absolute configuration of abietane diterpenoids from Salvia clinopodioides: antioxidant, antiprotozoal, and antipropulsive activities, J. Nat. Prod., 2019, 82, 1207–1216 CrossRef CAS PubMed.
- X. Y. Pang, X. P. Lin, J. Yang, X. F. Zhou, B. Yang, J. F. Wang and Y. H. Liu, Spiro-phthalides and isocoumarins isolated from the marine-sponge-derived fungus Setosphaeria sp SCSIO41009, J. Nat. Prod., 2018, 81, 1860–1868 CrossRef CAS PubMed.
- K. Kato, M. Ninomiya, K. Tanaka and M. Koketsu, Effects of functional groups and sugar composition of quercetin derivatives on their radical scavenging properties, J. Nat. Prod., 2016, 79, 1808–1814 CrossRef CAS PubMed.
- Z. Z. Jiao, S. Yue, H. X. Sun, T. Y. Jin, H. N. Wang, R. X. Zhu and L. Xiang, Indline amide glucosides from Portulaca oleracea: isolation, structure, and DPPH radical scavenging activity, J. Nat. Prod., 2015, 78, 2588–2597 CrossRef CAS PubMed.
- K. H. Kim, Y. J. Park, K. H. Chung, M. L. R. Yip, J. Clardy, D. Senger and S. Cao, Iridoid glycosides from Barleria lupulina, J. Nat. Prod., 2015, 78, 320–324 CrossRef CAS PubMed.
- S. S. Chen, H. N. Zhou, G. Zhang, Q. Dong, Z. H. Wang, H. L. Wang and N. Hu, Characterization, antioxidant, and neuroprotective effects of anthocyanins from Nitraria tangutorum Bobr. fruit, Food Chem., 2021, 353, 129435 CrossRef CAS PubMed.
- U. Jug, K. Naumoska and I. Vovk, (-)-Epicatechin-an important contributor to the antioxidant activity of Japanese Knotweed Rhizome bark extract as determined by antioxidant activity-guided fractionation, Antioxidants, 2021, 10, 133 CrossRef CAS PubMed.
- Y. Z. Dawa, Y. R. Du, Q. Wang, C. B. Chen, D. L. Zou, D. S. Qi, J. B. Ma and J. Dang, Targeted isolation of 1,1-diphenyl-2-picrylhydrazyl inhibitors from Saxifraga atrata using medium- and high-pressure liquid chromatography combined with online high performance liquid chromatography-1,1-diphenyl-2-picrylhydrazyl detection, J. Chromatogr. A, 2021, 1635, 461690 CrossRef CAS PubMed.
- V. Radenkovs, T. Pussa, K. Juhnevica-Radenkova, J. Kviesis, F. J. Salar, D. A. Moreno and I. Drudze, Wild apple (Malus spp.) by-products as a source of phenolic compounds and vitamin C for food applications, Food Biosci., 2020, 38, 100744 CrossRef CAS.
- L. Dienaite, M. Pukalskiene, C. V. Pereira, A. A. Matias and P. R. Venskutonis, Valorization of european Cranberry Bush (Viburnum opulus L.) berry pomace extracts isolated with pressurized ethanol and water by assessing their phytochemical composition, antioxidant, and antiproliferative activities, Foods, 2020, 9, 1413 CrossRef CAS PubMed.
- J. Dang, C. B. Chen, J. B. Ma, Y. Dawa, Q. Wang, Y. D. Tao, Q. L. Wang and T. F. Ji, Preparative isolation of highly polar free radical inhibitor from Floccularia luteovirens using hydrophilic interaction chromatography directed by on-line HPLC-DPPH assay, J. Chromatogr. B: Anal. Technol. Biomed. Life Sci., 2020, 1142, 122043 CrossRef CAS PubMed.
- F. Braham, D. O. Carvalho, C. M. R. Almeida, F. Zaidi, J. M. C. S. Magalhaes, L. F. Guido and M. P. Goncalves, Online HPLC-DPPH screening method for evaluation of radical scavenging phenols extracted from Moringa oleifera leaves, S. Afr. J. Bot., 2020, 129, 146–154 CrossRef CAS.
- W. D. Wang, L. J. Jiao, Y. D. Tao, Y. Shao, Q. L. Wang, R. T. Yu, L. J. Mei and J. Dang, On-line HPLC-DPPH bioactivity-guided assay for isolated of antioxidative phenylpropanoids from Qinghai-Tibet Plateau medicinal plant Lancea tibetica, J. Chromatogr. B: Anal. Technol. Biomed. Life Sci., 2019, 1106, 1–10 CrossRef PubMed.
- G. Pepe, E. Sommella, D. Cianciarulo, C. Ostacolo, M. Manfra, V. Di Sarno, S. Musella, M. Russo, A. Messore, B. Parrino, A. Bertamino, G. Autore, S. Marzocco and P. Campiglia, Polyphenolic extract from Tarocco (Citrus sinensis L. Osbeck) clone “Lempso” exerts anti-inflammatory and antioxidant effects via NF-kB and Nrf-2 activation in murine macrophages, Nutrients, 2018, 10, 1961 CrossRef PubMed.
- A. Acquaviva, A. Jones, G. R. Dennis, R. A. Shalliker and A. Soliven, Detection selectivity in the analysis of “reactive” chemical compounds derived from natural samples via reaction flow chromatography, Microchem. J., 2018, 139, 315–321 CrossRef CAS.
- M. M. Zhang, S. P. Cheng, Y. Liang, Y. Mu, H. J. Yan, Q. Liu, Y. L. Geng, X. Wang and H. Q. Zhao, Rapid purification of antioxidants from Magnolia officinalis by semi-prep-HPLC with a two-step separation strategy guided by on-line HPLC-radical scavenging detection, J. Chromatogr. B: Anal. Technol. Biomed. Life Sci., 2018, 1100, 104–147 CrossRef PubMed.
- H. D. Zhao, X. Hu, X. Q. Chen, S. Y. Shi, X. Y. Jiang, X. J. Liang, W. Chen and S. H. Zhang, Analysis and improved characterization of minor antioxidants from leaves of Malus doumeri using a combination of major constituents' knockout with high-performance liquid chromatography-diode array detector-quadrupole time-of-flight tandem mass spectrometry, J. Chromatogr. A, 2015, 1398, 57–65 CrossRef CAS PubMed.
- Z. D. Li, D. S. Jiang, Y. P. Dai, Z. S. Dai, Y. Jin, Q. Fu and X. M. Liang, Isolation of three polyoxins by reversed-phase liquid chromatography with pure aqueous mobile phase, J. Sep. Sci., 2021, 44, 2020–2028 CrossRef CAS PubMed.
- Y. J. Li, H. M. Lin, J. B. Zhang and X. Deng, An efficient procedure for preparing high-purity pingyangmycin and boanmycin from Streptomyces verticillus var. pingyangensis fermentation broth via macroporous cation-exchange resin and subsequent reversed-phase preparative chromatography, J. Chromatogr. B: Anal. Technol. Biomed. Life Sci., 2020, 1136, 121883 CrossRef CAS PubMed.
- I. A. H. Ahmad, V. Shchurik, T. Nowak, B. F. Mann and E. L. Regalado, Introducing multifactorial peak crossover in analytical and preparative chromatography via computer-assisted modeling, Anal. Chem., 2020, 92, 13443–13451 CrossRef PubMed.
- G. Adili, G. Y. Sun, M. Abuduaini, Y. X. Zhao, R. Abdulla and H. A. Aisa, Simultaneous determination of loading capacity and selectivity in preparative off-line two-dimensional separation: an application for purification of corilagin from Pomegranate flower extracts, J. Chromatogr. A, 2020, 1622, 461129 CrossRef CAS PubMed.
- E. F. Queiroz, A. Alfattani, A. Afzan, L. Marcourt, D. Guillarme and J. L. Wolfender, Utility of dry load injection for an efficient natural products isolation at the semi-preparative chromatographic scale, J. Chromatogr. A, 2019, 1598, 85–91 CrossRef CAS PubMed.
- Y. Q. Wang, X. Tang, J. F. Li, Y. L. Wu, Y. Y. Sun, M. J. Fang, Z. Wu, X. M. Wang and Y. K. Qiu, Development of an on-line mixed-mode gel liquid chromatography x reversed phase liquid chromatography method for separation of water extract from Flos Carthami, J. Chromatogr. A, 2017, 1519, 145–151 CrossRef CAS PubMed.
- X. Y. Wang, J. F. Li, Y. M. Jian, Z. Wu, M. J. Fang and Y. K. Qiu, On-line comprehensive two-dimensional normal-phase liquid chromatography × reversed-phase liquid chromatography for preparative isolation of Peucedanum praeruptorum, J. Chromatogr. A, 2015, 1387, 60–68 CrossRef CAS PubMed.
- Y. K. Qiu, F. F. Chen, L. L. Zhang, X. Yan, L. Chen, M. J. Fang and Z. Wu, Two-dimensional preparative liquid chromatography system for preparative separation of minor amount components from complicated natural products, Anal. Chim. Acta, 2014, 820, 176–186 CrossRef CAS PubMed.
- Q. Wang, W. J. Chen, Q. L. Wang, Y. D. Tao, R. T. Yu, G. Q. Pan and J. Dang, Preparative separation of isoquinoline alkaloids from Corydalis impatiens using middle chromatogram isolated gel column coupled with positively charged reversed-phase liquid chromatography, J. Sep. Sci., 2020, 43, 2521–2528 CrossRef CAS PubMed.
- G. Q. Pan, J. W. Shen, Y. H. Ma, Y. F. He, Y. Bao, R. R. Li, S. S. Wang, Q. Wang, P. C. Lin and J. Dang, Preparative separation of isoquinoline alkaloids from Corydalis impatiens using a middle-pressure chromatogram isolated gel column coupled with two-dimensional liquid chromatography, J. Sep. Sci., 2019, 42, 3182–3190 CrossRef CAS PubMed.
- D. Pei, X. J. Xi, X. Y. Huang, K. J. Quan, J. T. Wei, N. L. Wang and D. L. Di, Isolation of high-purity peptide Val-Val-Tyr-Pro from globin peptide using MCI gel column combined with high-speed counter-current chromatography, J. Sep. Sci., 2018, 41, 4559–4566 CrossRef CAS PubMed.
- F. L. Hsu, W. J. Huang, T. H. Wu, M. H. Lee, L. C. Chen, H. J. Lu, W. C. Hou and M. H. Lin, Evaluation of antioxidant and free radical scavenging capacities of polyphenolics from pods of Caesalpinia pulcherrima, Int. J. Mol. Sci., 2012, 13, 6073–6088 CrossRef CAS PubMed.
- L. Verotta, M. Dell'Agli, A. Giolito, M. Guerrini, P. Cabalion and E. Bosisio, In vitro antiplasmodial activity of extracts of Tristaniopsis species and identification of the active constituents: ellagic acid and 3,4,5-trimethoxyphenyl-(6'-O-galloyl)-O-β-D-glucopyranoside, J. Nat. Prod., 2001, 64, 603–607 CrossRef CAS PubMed.
- B. Bennini, A. J. Chulia, M. Kaouadji, P. Fondaneche and D. P. Allais, Diarylnonanoids and their glucosides from Erica cinerea, Tetrahedron Lett., 2011, 52, 1597–1600 CrossRef CAS.
- X. H. Zhao, B. Dong, P. Li, W. Wei, J. Dang, Z. G. Liu, Y. D. Tao, H. P. Han, Y. Shao and H. L. Yue, Fatty acid and phytosterol composition, and biological activities of Lycium ruthenicum Murr. seed oil, J. Food Sci., 2018, 83, 2448–2456 CrossRef CAS PubMed.
- S. Damiano, M. Forino, A. De, L. A. Vitali, G. Lupidi and O. Taglialatela-Scafati, Antioxidant and antibiofilm activities of secondary metabolites from Ziziphus jujuba leaves used for infusion preparation, Food Chem., 2017, 230, 24–29 CrossRef CAS PubMed.
Footnote |
† Electronic supplementary information (ESI) available. See DOI: 10.1039/d1ra05819c |
|
This journal is © The Royal Society of Chemistry 2021 |
Click here to see how this site uses Cookies. View our privacy policy here.