DOI:
10.1039/C5FO00904A
(Paper)
Food Funct., 2016,
7, 250-261
Proteomic analysis of intestinal tissues from mice fed with Lentinula edodes-derived polysaccharides†
Received
23rd July 2015
, Accepted 4th September 2015
First published on 8th September 2015
Abstract
Lentinula edodes-derived polysaccharides are well known for their immunomodulation and antitumor activities. However, the mechanisms of action have not been fully elucidated. This study presents proteomic analysis of the colon and small intestine from mice fed with an immunostimulating heteropolysaccharide L2 from the fruit body of L. edodes. Two-dimensional gel electrophoresis (2-DE) and MALDI-TOF-TOF MS/MS were employed to characterize the protein profiles. Twenty nine gel spots representing 20 proteins in colon tissues and 38 gel spots in small intestine tissues representing 23 proteins were identified as showing significant changes in abundance. These differential proteins in abundance are mainly involved in metabolism, binding, structural components, and response to stimulus. Protein–protein interaction network analysis demonstrated mapping of the 20 colon proteins to a 7-protein and a 3-protein sub-network, and mapping of the 23 small intestine proteins to a 9-protein and a 5-protein sub-network. All the 40 altered proteins were integrated into a unified network containing 25 proteins, suggesting the existence of a concerted mechanism, although acting on the colon and small intestine separately. These findings facilitate the understanding of the regulatory mechanism in response to L2 treatment.
1 Introduction
Mushrooms are important resources of active substances known for their widespread availability and long history of use in medicine and food in East Asia.1 A number of active substances have been obtained from mushrooms including small molecule compounds, polysaccharides, proteins, and polysaccharide–protein complexes.2 Among all the bioactive constituents, mushroom polysaccharides are known to possess various biological activities, including antibacterial activity,3 immunomodulation,4 anti-infection5 and antitumor activities.6Lentinula edodes is considered as the first medicinal mushroom and the second most popular edible mushroom in the world.7 It has a long history in oriental medicine for the treatment of weakness, ageing, and diseases.7L. edodes-derived polysaccharides are especially remarkable for their immunomodulation and antitumor activities.8,9 However, their mechanisms of action have not been fully elucidated.
The proteomic approach is used to analyse the global accumulation of proteins from a tissue or cell type, and can provide direct information relative to biological systems such as metabolic pathways and regulatory networks. The traditional label-free quantitative proteomics and qualitative proteomics are two-dimensional electrophoresis (2-DE) coupled with mass spectrometry.10 In the past ten years, proteomic approaches have hardly been employed to understand the acting mechanisms of medicinal and nutritional benefits induced by mushroom polysaccharides. For example, comparative proteomics on mouse splenic mononuclear cells treated with polysaccharides extracted from Ganoderma lucidum spores (GL-SP), showed ten differential proteins in abundance.11 Similarly, using the proteomic technique, Li et al.12 investigated the antitumor efficacy of G. lucidum polysaccharides (GLP), the results revealed that GLP inhibited the tumor growth and tumor cell adhesion to human umbilical cord vascular endothelial cells through up-regulation of serum amyloid A. To date, the bioactivity mechanism of L. edodes-derived polysaccharides in vivo has not been studied using a proteomics-based method.
In a previous study,13 we reported the isolation of a new heteropolysaccharide L2 (26 kDa, >97% purity) with immunostimulating activity from the fruit body of L. edodes. Chemical characterization indicated that L2 consists of glucose (87.5%), galactose (9.6%), and arabinose (2.8%). Further studies demonstrated the immunostimulating activity of L2 in vivo and that it could change the composition of gut microbiota along the intestine, especially in the colon.14,15 The gut microbiota plays an important role in modulating the expression of a large set of genes along the mouse intestine,16 indicating that the immunostimulating mechanism of L2 may be associated with differential gene transcription and protein accumulation in the intestine. The present study investigates proteomic profile changes in the colon and small intestine tissues of C57BL/6 mouse fed with L2, and the potential modulation of proteins and networks, to evaluate the underlying mechanisms of immunostimulation.
2 Experimental section
2.1 Materials
L. edodes-derived polysaccharide L2 was obtained as described before.13 Briefly, the fruit body of L. edodes was extracted with boiling water over the course of 2 h at a ratio of 20
:
1 (w/w). The supernatants were concentrated at 80 °C. The precipitates were obtained using 95% ethanol and dried under vacuum. Then, the precipitates were dissolved in distilled water, followed by deproteination using the Sevag method. This process was repeated five times. After being precipitated and redissolved by 95% ethanol and distilled water, respectively, the resulting solution was then freeze-dried to obtain crude polysaccharides. DEAE (diethylaminoethyl)-52 cellulose column chromatography and Sephadex G-200 column chromatography were used for further separation to obtain high purity L. edodes-derived polysaccharide L2. All reagents used in this study were of at least analytical grade.
2.2 Animal experiments
Specific pathogen-free male C57BL/6 mice (8-week old) were bought from Vital River Laboratory Animal Technology Co. Ltd (Beijing, China). The mice were kept at a temperature of 22 °C and in a 12 h light/dark cycle environment for at least two weeks before use, and fed on the same batch of standard laboratory diet to minimize the variation of environmental factors. The experiments were approved by the Animal Care Welfare Committee of Guangzhou University of Chinese Medicine. Adequate measures were taken to minimize pain of the experimental animals. The mice were randomly divided into two groups for 28 consecutive days: (1) L. edodes-derived polysaccharide L2 treatment groups (gavage administration of L2 at a daily dose of 40 mg per kg body weight, n = 7) (L2-treated groups), which were reared in the same cage; (2) normal groups (gavage administration with the same volume of sterile physiological saline, n = 7), which were reared in the same cage. On the last day, the mice were sacrificed by cervical dislocation, the small intestine and colon tissues were sterilely collected separately and fully washed with phosphate buffered saline (PBS), and then immediately stored at −80 °C until further analysis. Three biological replicates were used per condition for the following experiments.
2.3 Protein extraction
Randomly selected colon tissues and small intestine tissues of three mice from each group were prepared as previously described with minor modification.17 In brief, tissue samples were ground in liquid nitrogen and lysed in lysis buffer containing 7 M urea, 2% CHAPS {3-[(3-cholamidopropyl)-dimethylammonio]-1-propanesulfonate}, 2 M thiourea (Amersham), 20 mM Tris-HCl (pH 8.5, Amresco) and 1 mM PMSF (phenylmethylsulfonyl fluoride), followed by sonication on ice (80 W, 0.8 s treatment and 0.8 s duration, 10 times, with 2 min intervals, repeated 6 times) and centrifugation at 12
000g for 20 min at 4 °C. The supernatant was transferred to a new tube with four times the volume of acetone added. The mixture was then precipitated overnight at −20 °C and centrifuged. The precipitate was dried under vacuum conditions and stored at −80 °C until further use.
2.4 Two-dimensional polyacrylamide gel electrophoresis
Two-dimensional electrophoresis (2DE) analysis was performed according to a protocol described previously.17 The precipitate was dissolved in rehydration buffer (7 M urea, 2% CHAPS, 2 M thiourea). Then, the solution was centrifuged at 12
000g for 20 min at 4 °C. The supernatant was transferred to a new tube. The protein content of the supernatant was determined using a 2D Quant kit (GE Healthcare, USA) according to the manufacturer's instructions. The one-dimensional isoelectric focusing (IEF) was performed with Dry Strip IPG strips of 24 cm (pH 4–7), which were rehydrated overnight at room temperature with 460 μL (100 μg of protein for silver staining and 1200 μg of protein for Coomassie Brilliant Blue staining, respectively) of the protein sample in the rehydration buffer (1% DTT, 1% IPG buffer, 1× BPB, bromophenol blue), using the Ettan IPGphor 3 System (GE Healthcare, USA). Isoelectric focusing was performed at 20 °C with the following parameters: 300 V for 0.5 h, 700 V for 0.5 h, 1500 V for 1.5 h, 9000 V for 3 h, and 9000 V for 4 h, for a total of 52
000 V h. After completion of the IEF program, the strips were stored at −20 °C.
Prior to the two-dimensional analysis, the individual strips were equilibrated in order to reduce and alkylate proteins: 15 min in 8 ml denaturing solution (6 M urea, 30% glycerol, 2% SDS, 0.375 M Tris (pH 8.8), 0.1 M DTT) and subsequently for 15 min in 8 ml equilibration buffer containing 250 mM IAA (iodoacetamide). After equilibration, the two-dimensional electrophoresis was performed on a 12.5% SDS polyacrylamide gel using the Ettan DALTSix System (GE Healthcare). The proteins were separated by running the gels at 2 W per gel for the first 45 min and followed by 17 W per gel for about 4.5 h until the dye front reached the bottom of the gel. Finally, the gels were fixed in a fixing solution (ethanol
:
glacial acetic acid
:
deionized water = 4
:
1
:
5) for 2 h. The protein spots were visualized via silver staining or Coomassie Brilliant Blue G-250 staining (for MS analysis). Subsequently, protein spot evaluation and data analysis were carried out using a scanner (Powerlook1100, UMAX) at 200 dpi (dots/in) and the Image Master 2D platinum 5.0 (GE Healthcare). Spots were detected by the 2-DElite automatic spot detection program. To select differential protein spots in abundance, quantitative analysis based on the data from the Image Master 2D platinum 5.0 (GE Healthcare) was performed between tissues of the normal and L2 treated groups on the triplicate gels. Briefly, spot detection, spot measurement, background subtraction and spot matching were performed. Prior to performing spot matching between gel images, one gel image was selected as the reference gel. After automatic matching, the unmatched spots of the member gels were added to the reference gel. The amount of a protein spot was expressed as the volume of that spot which was defined as the sum of the intensities of all the pixels that make up the spot. In order to correct the variability due to staining and to reflect the quantitative variation in the intensity of protein spots, the spot volumes were normalized as a percentage of the total volume in all of the spots present in the gel. The molecular mass of each protein was estimated by comparison with those of a standard marker set, and the isoelectric points (pIs) were determined by the spot positions along the immobilized pH gradient strips. The resulting data from the image analysis were analysed using Student's t-test. Significantly differential protein spots in abundance were labeled in the images and were chosen for further analysis. Three biological replicates were performed for 2-DE analysis to reduce variations.
2.5 In-gel digestion and mass spectrometric analysis
Spots meeting the above criteria were selected and manually excised from the stained gels, then washed and digested with trypsin (Promega, Madison, USA) as previously described.18 The protein sample was dissolved in 1.5 μl solution containing MilliQ water, 30% acetonitrile (ACN) and 0.1% trifluoroacetic acid (TFA). Then, 0.8 μl of the mixture was loaded onto a target plate with a 0.5 μl HCCA (5 mg ml−1 α-cyano-4-hydroxycinnamic acid) matrix containing 50% ACN and 0.1% TFA, dried at room temperature, and applied to further mass spectrometry analysis, which was performed by matrix-assisted laser desorption/ionization time-of-flight tandem time-of-flight mass spectrometry (MALDI-TOF-TOF MS) with a mass spectrometer (Bruker Dalton, Germany). The digests were analyzed by peptide mass fingerprint (PMF) with MALDI-TOF and the precursor ions were chosen for MS/MS analysis in LIFT mode. The number of precursor peaks per spot and S/N filters was set at 5 and 20, respectively. The following threshold criteria and settings were used in the mass spectrometer: a detected mass range of 700–3200 Da (optimal resolution for the quality of 1500 Da). The UV laser was operated at a 200 Hz repetition rate with a wavelength of 355 nm and accelerated voltages of 25 kV and 21.7 kV, respectively. All mass spectra were recorded in positive reflector mode. MALDI-TOF-TOF MS/MS spectra were calibrated using trypsin autodigestive peptide signals and matrix ion signals. Flex Analysis (Bruker Dalton, Germany) and BioTools (Bruker Dalton, Germany) software were used for peptide searching. PMF spectra and MS/MS spectra were interpreted with the MASCOT software against NCBInr database and Swiss-Prot database to identify the protein (Matrix Science, UK, http://www.matrixscience.com). Search parameters were set as follows: tolerance peptide mass variance: 50 ppm; tolerance fragment mass variance: 0.6 Da; fixed modification: carbamidomethyl (C); variable modification: acetyl (N-term) and oxidation (M); taxonomy: mouse; enzyme: trypsin. For the MASCOT search results, protein scores greater than 55 were considered significant (p < 0.05). The molecular function and biological process of the differential proteins in abundance were defined by version 10.0 of PANTHER (Proteins ANalysis THrough Evolutionary Relationships)19 (http://www.pantherdb.org/). Protein–protein interaction networks were built by version 9.1 of STRING20 (http://string-db.org/).
2.6 Western blot
Proteins (20 μg) were separated by 10% SDS-PAGE (7.3 cm × 8.3 cm) and were transferred to a nitrocellulose membrane at 300 mA (tank blot). Subsequently, the membrane was blocked with 5% nonfat dry milk in TBS (150 mM NaCl, 100 mM Tris-HCl, pH 7.5) for 1 h at room temperature and then incubated with the first antibody separately: 78 kDa glucose-regulated protein (GRP78) (1
:
1000 dilution, Santa Cruz Inc., USA); vimentin (VIM) (1
:
500 dilution, Santa Cruz Inc., USA); polymerase I and transcript release factor (PTRF) (1
:
500 dilution, Santa Cruz Inc., USA); calumenin (CALU) (1
:
1000 dilution, Santa Cruz Inc., USA); carbonyl reductase 3 (CRA) (1
:
500 dilution, Santa Cruz Inc., USA); tropomyosin (TP) (1
:
1000 dilution, Santa Cruz Inc., USA); protein disulfide-isomerase A4 (PDIA4) (1
:
250 dilution, Sigma-Aldrich, MO, USA); dihydrolipoyllysine-residue succinyltransferase component of 2-oxoglutarate dehydrogenase complex (DLST) (1
:
500 dilution, Sigma-Aldrich, MO, USA); farnesyl pyrophosphate synthase (FPS) (1
:
250 dilution, Sigma-Aldrich, MO, USA); serum amyloid P-component (SAP) (1
:
800 dilution, Sigma-Aldrich, MO, USA); myosin light polypeptide 6 (MLP6) (1
:
500 dilution, Sigma-Aldrich, MO, USA); heat shock 70 kDa protein 1A (HS71A) (1
:
250 dilution, Santa Cruz Inc., USA) for 1 h under the same conditions. The membrane was washed twice with TBST (150 mM NaCl, 0.05% Tween-20, 100 mM Tris-HCl, pH 7.5) at room temperature and then incubated with a secondary antibody (goat anti-rabbit) following the manufacturer's protocol conjugated with horseradish peroxidase (HRP). The membrane was washed with TBST again, and the blot was developed using the western lightning chemiluminescence reagent. Glyceraldehyde-3-phosphate dehydrogenase (GAPDH) was used as an internal control for the tests.
2.7 Statistical analysis
Student's t-test and one-way analysis of variance (ANOVA) were used to analyze differences. P-Values less than 0.05 were considered as statistically significant.
3 Results
3.1 2DE and mass spectrometry analysis of colon tissue
By 2-DE and quantitative analysis, twenty nine gel spots were significant in percent volume of gels (p < 0.05) (Table S1†). Thirteen gel spots indicating down-accumulation and 16 gel spots indicating up-accumulation in L2-treated mice are shown as labeled by characters and numbers in the 2-DE images (Fig. S1a and S1b†). The differential proteins in abundance were excised by a spot picker and in-gel digested by trypsin. Proteins were identified with MALDI-TOF-TOF MS/MS by PMF and the sequencing of selected peptides was performed in LIFT mode. Further MASCOT search identified 20 differential proteins in the colon tissues (Table 1, ESI† CLN MASCOT). Among these proteins, DLST and mucosal pentraxin precursor were down-accumulated, i.e. not detectable in L2-treated groups (N12, N25). In contrast, GRP78, PDIA4, and SAP were up-accumulated, i.e. not detected in normal mice (Na10, Na18, Na30). The 19 differential proteins in abundance are classified in three functional categories (mucosal pentraxin precursor was not found in the GO database): binding, catalytic activity, and structural molecule activity (Fig. 1a). They belong to 6 main biological processes, namely cellular component, and cellular, developmental, metabolic, and multicellular organismal processes, and response to stimulus, and involve 8 pathways, such as, apoptosis signaling (HS71A and GPR78), cholesterol biosynthesis (FPS) and Parkinson's disease (HS71A, GPR78, 14-3-3 protein zeta/delta) (Fig. 1a).
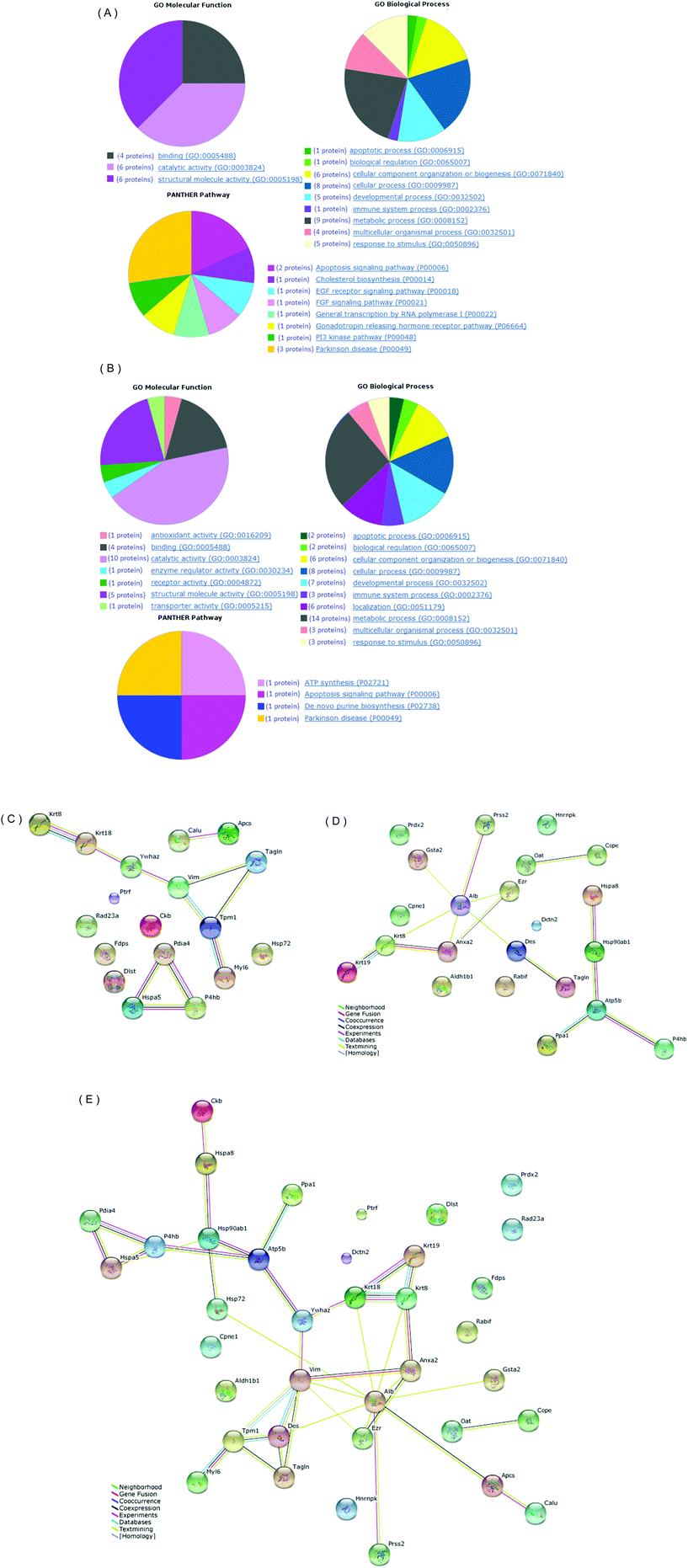 |
| Fig. 1 GO analysis in the colon (a) and small intestine (b) by PANTHER; protein–protein interaction network identified by STRING for differential proteins in abundance (the corresponding gene names are shown), (c) colon, (d) small intestine, (e) both colon and small intestine. Different line colors represent the types of evidence for association. | |
Table 1 Differential proteins in abundance identified in colon tissue in 2DE (n = 3)
Label No. |
Accession |
Protein name |
Gene symbol |
Experimental Mw (Da)/pI |
Fold changea |
P
|
Fold change is calculated as the ratio of the percent volume of a spot in two groups. The lowest value is listed in the table.
P, Student's t-test. —, Spot is not detectable in either group. |
N2 |
P08003 |
Protein disulfide-isomerase A4 |
Pdia4 |
71 726/5.23 |
−1.6 |
<0.001 |
N3 |
Q61696 |
Heat shock 70 kDa protein 1A |
Hspa1a |
64 712/5.54 |
−3.4 |
<0.01 |
N4 |
Q61696 |
Heat shock 70 kDa protein 1A |
64 794/5.56 |
−2.4 |
<0.001 |
N12 |
Q9D2G2 |
Dihydrolipoyllysine-residue succinyltransferase component of 2-oxoglutarate dehydrogenase complex |
Dlst |
49 168/4.65 |
— |
<0.01 |
N16 |
P11679 |
Keratin, type II cytoskeletal 8 |
Krt8 |
39 228/5.10 |
−1.6 |
<0.01 |
N17 |
P05784 |
Keratin, type I cytoskeletal 18 |
Krt18 |
39 456/4.85 |
−1.6 |
<0.01 |
N19 |
Q920E5 |
Farnesyl pyrophosphate synthase |
Fdps |
37 126/5.52 |
−1.6 |
<0.01 |
N25 |
IPI00828912 |
Mucosal pentraxin precursor |
|
25 656/5.41 |
— |
<0.001 |
N26 |
IPI00828912 |
Mucosal pentraxin precursor |
25 138/5.41 |
−3.1 |
<0.01 |
N27 |
P63101 |
14-3-3 protein zeta/delta |
Ywhaz |
24 327/4.76 |
−3.8 |
<0.001 |
Na10 |
P20029 |
78 kDa glucose-regulated protein |
Hspa5 |
69 661/4.65 |
— |
<0.001 |
Na17 |
P20152 |
Vimentin |
Vim |
59 659/5.12 |
2.1 |
<0.01 |
Na18 |
P09103 |
Protein disulfide-isomerase |
P4hb |
58 384/4.81 |
— |
<0.001 |
Na19 |
O54724 |
Polymerase I and transcript release factor |
Ptrf |
53 872/4.18 |
2.3 |
<0.001 |
Na21 |
P54726 |
UV excision repair protein RAD23 homolog A |
Rad23a |
49 287/4.60 |
2.4 |
<0.01 |
Na24 |
O35887 |
Calumenin |
Calu |
43 210/4.56 |
4.7 |
<0.001 |
Na25 |
Q04447 |
Creatine kinase B-type |
Ckb |
42 218/4.54 |
4.1 |
<0.01 |
Na28 |
IPI00169998 |
Carbonyl reductase 3 |
Cbr3 |
27 468/6.32 |
1.7 |
<0.01 |
Na29 |
P58771 |
Tropomyosin alpha-1 chain |
Tpm1 |
26 832/4.72 |
2.7 |
<0.05 |
Na30 |
P12246 |
Serum amyloid P-component |
Apcs |
26 000/5.59 |
— |
<0.001 |
Na35 |
Q60605 |
Myosin light polypeptide 6 |
Myl6 |
14 462/4.21 |
14.7 |
<0.001 |
Na36 |
P37804 |
Transgelin |
Tagln |
13 538/5.69 |
1.5 |
<0.01 |
Na37 |
P37804 |
Transgelin |
13 430/6.07 |
1.7 |
<0.01 |
3.2 2-DE and mass spectrometry analysis of small intestine tissue
Similarly, 38 gel spots were significant in percent volume after L2 treatment (p < 0.05) (Table S2†). Twenty gel spots indicating down-accumulation and 18 gel spots indicating up-accumulation in L2-treated mice are shown labeled as characters and numbers in the 2-DE images (Fig. S2a and S2b†). Spot identification with MALDI-TOF-TOF MS/MS by PMF and sequencing of selected peptides and database search was performed: 23 proteins were identified, with 11 proteins noted to be down-accumulated and 12 proteins up-accumulated (Table 2, ESI† SMI MASCOT). Serum albumin, ATP synthase subunit beta, and ornithine aminotransferase were detected in normal mice only (B11, B28, B47, B53), whereas Dynactin subunit 2, glutathione S-transferase A2, heat shock cognate 71 kDa protein and Transgelin were present in L2-treated groups only (A45, A49, A50, A51). The 23 differential proteins in abundance mainly belong to three main molecular functional categories: binding, catalytic activity, structural molecule activity (Fig. 1b). These are involved in 10 biological processes, such as, cellular component, cellular process, developmental process, localization, and metabolic processes (containing 14 proteins), but only 4 pathways are enriched: ATP synthesis (ATP synthase subunit beta), apoptosis signaling (heat shock cognate 71 kDa protein), de novo purine biosynthesis (ornithine aminotransferase), and Parkinson disease (heat shock cognate 71 kDa protein) (Fig. 1b).
Table 2 Differential proteins in abundance identified in small intestine tissue in 2DE (n = 3)
Label no. |
Accession no. |
Protein name |
Gene symbol |
Experimental Mw (Da)/pI |
Fold changea |
P
|
Fold change is calculated as the ratio of the percent volume of a spot in two groups. The lowest value is listed in the table.
P, Student's t-test. —, Spot is not detectable in either group. |
B10 |
P09103 |
Protein disulfide-isomerase |
P4hb |
52 470/4.69 |
−2.5 |
<0.001 |
B11 |
P07724 |
Serum albumin |
Alb |
50 734/5.97 |
— |
<0.001 |
B13 |
P11499 |
Heat shock protein HSP 90-beta |
Hsp90ab1 |
42 784/4.85 |
−2.7 |
<0.001 |
B15 |
P31001 |
Desmin |
Des |
40 451/4.72 |
−2.5 |
<0.01 |
B28 |
P56480 |
ATP synthase subunit beta |
Atp5b |
36 203/5.00 |
— |
<0.001 |
B32 |
O89079 |
Coatomer subunit epsilon |
Cope |
31 231/4.87 |
−2.8 |
<0.001 |
B38 |
P07146 |
Anionic trypsin-2 |
Prss2 |
27 572/4.22 |
−3.1 |
<0.01 |
B47 |
P29758 |
Ornithine aminotransferase |
Oat |
25 045/4.76 |
— |
<0.001 |
B53 |
P07724 |
Serum albumin |
Alb |
23 678/5.69 |
— |
<0.001 |
B54 |
Q9D819 |
Inorganic pyrophosphatase |
Ppa1 |
23 603/4.80 |
−3.1 |
<0.001 |
B56 |
P19001 |
Keratin, type I cytoskeletal 19 |
Krt19 |
22 923/5.99 |
−3.7 |
<0.001 |
B59 |
P07356 |
Annexin A2 |
Anxaz |
22 355/5.30 |
−2.7 |
<0.01 |
B66 |
P19001 |
Keratin, type I cytoskeletal 19 |
Krt19 |
17 000/4.73 |
−4.4 |
<0.001 |
B70 |
P26040 |
Ezrin |
Ezr |
13 466/6.51 |
−2.7 |
<0.001 |
A07 |
P61979 |
Heterogeneous nuclear ribonucleoprotein K |
Hnrnpk |
64 207/5.49 |
2.1 |
<0.01 |
A10 |
Q8C166 |
Copine-1 |
Cpne1 |
61 714/5.47 |
2.6 |
<0.001 |
A15 |
P11679 |
Keratin, type II cytoskeletal 8 |
Krt8 |
54 631/5.88 |
2.5 |
<0.001 |
A17 |
P61979 |
Heterogeneous nuclear ribonucleoprotein K |
Hnrnpk |
49 276/6.19 |
2.0 |
<0.01 |
A19 |
Q9CZS1 |
Aldehyde dehydrogenase X |
Aldh1b1 |
43 169/6.49 |
2.3 |
<0.01 |
A23 |
P31001 |
Desmin |
Des |
39 109/4.63 |
2.4 |
<0.001 |
A24 |
P11679 |
Keratin, type II cytoskeletal 8 |
Krt8 |
39 015/4.88 |
— |
<0.01 |
A41 |
P31001 |
Desmin |
Des |
23 480/6.20 |
1.8 |
<0.01 |
A42 |
IPI00128108 |
Trypsin 4 |
Try4 |
22 543/6.24 |
2.0 |
<0.01 |
A45 |
Q99KJ8 |
Dynactin subunit 2 |
Dctn2 |
20 865/5.76 |
— |
<0.001 |
A48 |
Q61171 |
Peroxiredoxin-2 |
Prdx2 |
19 927/4.64 |
2.3 |
<0.001 |
A49 |
P10648 |
Glutathione S-transferase A2 |
Gsta2 |
18 157/5.89 |
— |
<0.001 |
A50 |
P63017 |
Heat shock cognate 71 kDa protein |
Hspa8 |
17 395/6.50 |
— |
<0.001 |
A51 |
P37804 |
Transgelin |
Tagln |
17 143/6.66 |
— |
<0.001 |
A53 |
Q91X96 |
Guanine nucleotide exchange factor MSS4 |
Rabif |
14 564/5.15 |
1.9 |
<0.01 |
3.3 Protein–protein interaction network analysis
To further explore the likely mechanisms of L2 in mice, protein–protein interaction network analysis was performed on the differential proteins in abundance using STRING. The 20 differential proteins in abundance from mice colon tissues were mapped to an interaction network (Fig. 1c), in which two sub-networks were enriched: one included 7 proteins (Krt8, Krt18, Ywhaz, Vim, Tagln, Tpm1, Myl6) and another included 3 proteins (Pdia4, Hspa5, P4bh). Similarly, the 23 differential proteins in abundance from small intestine tissues were mapped to an interaction network (Fig. 1d), and two sub-networks were formed, each consisting 9 (Krt19, Krt8, Anxa2, Ezr, Alb, Gsta2, Des, Prss2, Tagln) and 5 proteins (Hspa8, Hsp90b1, Atp5b, Ppa1, P4hb). Furthermore, all the 40 differential proteins in abundance from the colon and small intestine tissues were mapped to an interaction network, and a bigger sub-network was generated, in which 25 proteins were included (Fig. 1e).
3.4 Western blot verification
Of the differential proteins in abundance from the colon of mice, 12 proteins were selected for western blot validation (Fig. 2). The results indicated that L2 treatment significantly increased the abundance of GPR78, VIM, PTRF, CRA, SAP, and MLP6, but clearly reduced the abundance of PDIA4, DLST, FPS, and HS71A. However, no difference was observed in the accumulation levels of CALU and TP. In all, 10 proteins exhibited consistent results between 2-DE and western blot experiments, except for CALU and TP, which exhibited similar abundance in normal and L2-treated mice (Table 3).
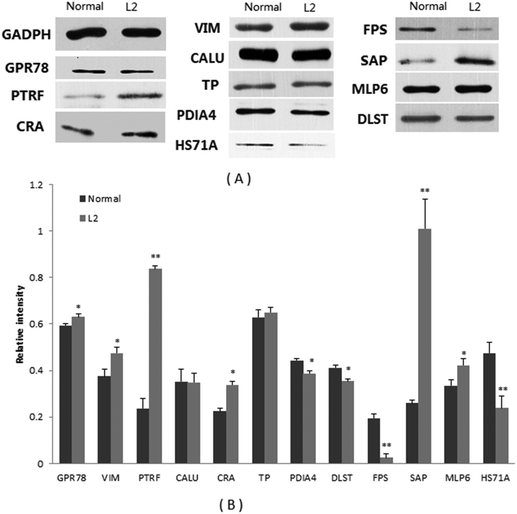 |
| Fig. 2 Western blot analysis of 12 proteins in the colon tissues. Glyceraldehyde-3-phosphate dehydrogenase (GAPDH) was used as an internal control. (A) Representative immunoblot results: GAPDH, GPR78, PTRF, CRA, VIM, CALU, TP, PDIA4, FPS, SAP, MLP6, DLST, HS71A. (B) Densitometric analysis of blots for 12 proteins. Normal: normal mice. L2: L2-treated mice. GAPDH, glyceraldehyde-3-phosphate dehydrogenase; GPR78, 78 kDa glucose-regulated protein; PTRF, polymerase I and transcript release factor; CRA, carbonyl reductase 3; VIM, vimentin; CALU, calumenin; TP, tropomyosin; PDIA4, protein disulfide-isomerase A4; FPS, farnesyl pyrophosphate synthase; SAP, serum amyloid P-component; MLP6, myosin light polypeptide 6; DLST, dihydrolipoyllysine-residue succinyltransferase component of 2-oxoglutarate dehydrogenase complex; HS71A, heat shock 70 kDa protein 1A. Values are mean ± SD of 3 animals. *p < 0.05, **p < 0.01, compared with normal mice. ANOVA. | |
Table 3 Summary of the 2DE and western blot results in normal and L2-treated mice
|
Relative abundance in colon tissue (n = 3) |
Protein name |
Control (WB) |
L2-treated (WB) |
Fold change (2DE) |
WB: western blot. *p < 0.05, **p < 0.01. |
GADPH (marker) |
1 |
1 |
— |
GPR78 |
0.594 |
0.631* |
6.1 |
VIM |
0.375 |
0.574* |
2.1 |
PTRF |
0.237 |
0.836** |
2.3 |
CALU |
0.352 |
0.349 |
2.7 |
CRA |
0.225 |
0.339* |
1.7 |
TP |
0.628 |
0.650 |
2.7 |
PDIA4 |
0.443 |
0.388* |
−1.6 |
DLST |
0.412 |
0.354* |
— |
FPS |
0.194 |
0.025** |
−1.6 |
SAP |
0.260 |
1.009** |
— |
MLP6 |
0.334 |
0.422* |
14.1 |
HS71A |
0.474 |
0.238** |
−2.4 |
4 Discussion
The intestine is exposed continuously to vast amounts of foreign antigenic material, such as gut microbiota and dietary components. At the same time, the intestinal barriers are the first line to interact and discriminate between harmful antigens and harmless materials. Mushroom polysaccharides are well known for their most fungal potent substances with antitumor and immunomodulation properties.21L. edodes is among the most valuable medicinal mushrooms.7 The oral administration of L. edodes-derived polysaccharides has shown antitumor and immunomodulation activities in vivo.7,9 However, its effects on gut tissue protein accumulation have not been investigated. The present study compares the proteomic profiles of mice colon and small intestine tissues with and without the oral administration of L. edodes-derived polysaccharide L2 by 2-DE analysis. A total of 20 and 23 differential proteins in abundance were successfully identified by MALDI-TOF-TOF MS/MS in the colon and small intestine tissues, respectively. A number of proteins displayed obvious differences in theoretical and experimental molecular weights, for instance, the decreased Keratin type II cytoskeletal 8, Transgelin, ornithine aminotransferase, Ezrin, Keratin type I cytoskeletal 19 and Dynactin subunit 2; the increased UV excision repair protein RAD23 homolog A and heterogeneous nuclear ribonucleoprotein K. This observation has also been reported in other proteomic studies.22,23 This may be due to the occurrence of protein degradation or protein complex. In addition, some proteins had different pI values but similar molecular weights, which might reflect different states of modification.24 For example, creatine kinase B-type was frequently phosphorylated in the disease state.25 Our proteome mining did not identify post-translational modifications since MALDI ionization tends to induce phosphorylation loss.26
Following the oral administration of L2 for four weeks, there was an increase in cytokine levels in the serum, along with an improvement in the natural killer cell (NK cell) activities and the proliferation of T cells of splenocytes.14 In particular, gene expression levels of the mucosa were affected along the intestine.14 Protein accumulations were altered by L2 in intestinal tissue. GO analysis revealed that most of the differential proteins in abundance in the colon and small intestine tissues were involved in cellular organization, metabolism, and developmental processes. Polysaccharides extracted from the mushrooms Agaricus bisporus, Agaricus blazei, and Phellinus linteus could lower NF-κB transcription in colon Caco-2 cells with IL-1β stimulation.27 Lentinan, as one of the types of shiitake mushroom polysaccharides, affected IL-8 mRNA expression in Caco-2 cells.28 These results indicate that L. edodes-derived L2 might directly influence the accumulation of proteins in the gut tissue. In addition, the gut is colonized by a dense community of commensal microbes, referred to as the gut microbiota. Mushroom polysaccharides are considered to be non-digestible carbohydrates that are fermented by gut microbiota.29 Numerous studies have demonstrated that polysaccharides can change both the composition as well as metabolites of intestinal microbiota,9 such as short chain fatty acids (SCFAs) (i.e. acetate, propionate, and butyrate), all of which play an important role in maintaining the epithelial barrier function, and regulating epithelial proliferation.30 The gut microbiota can significantly influence host development and metabolism homeostasis.31,32 Interestingly, the gut microbiota appear specifically in spatial distributions along the intestine.33 In our previous study, we reported that L2 can alter the structure of gut microbiota along the intestine in mice, especially in the colon.15 In the present study, most of the differential proteins varied between the colon and the small intestine, further indicating associations between protein accumulation and gut microbiota-related effects. Taken together, these results show not only the direct effects of L2, but also its impacts indirectly on the intestinal protein accumulations, such as gut microbiota-dependent effects.34 To illuminate the direct and indirect effects of L2 on protein accumulation in the intestine, more controls such as making the gut germ-free or sterilization of the gut by a broad-spectrum antibiotic mice model should be necessary in future studies.35
The differential proteins in abundance affected by L2 may help to elucidate the effects of mushroom polysaccharides on immune-modulation, as an anti-allergy, for protection of intestinal mucosa, and as a positive influence on the metabolism of lipid and sugars.7,36 In our previous study, L2 significantly enhanced mucosal immune and systematic immune responses.14 Previous results indicate that some of these proteins are involved in the host immune system function. For example, vimentin is known to maintain cellular integrity and provide resistance against stress. Xu et al.37 have demonstrated that vimentin plays an important role in T-cell vaccination, which has been effectively applied to the prevention and treatment of experimental autoimmune diseases. Tropomyosin is implicated in the pathogenesis of ulcerative colitis (UC). A predominant colonic epithelial Tm isoform, hTm5, can induce both humoral (B-cells) and cellular (T-cells) response in patients with UC. However, hTm5 seems to be expressed on the colon epithelial cell surface but not on small intestinal epithelial cells.38 The plasma protein SAP, a member of the pentraxin family of proteins, was increased in chronic Trichuris muris infected mice, indicating an association between the immune system function and SAP.39 Furthermore, SAP can activate the complement system.40 Interestingly, serum amyloid A3, of the family of serum amyloid proteins, was found to be regulated by gut microbiota,41 suggesting that SAP might also be regulated by gut microbiota. HS71A, GPR78, HSP90AB1, and HSPA8 belong to the family of heat shock proteins (HSPs). HSPs are produced by cells in response to stressful stimuli. The production of HSPs in intestinal epithelial cells regulated by the dietary component and gut microbiota is crucial for maintaining the mucosal barrier integrity, gut homeostasis as well as immune function.42 Protein disulfide-isomerase catalyzes disulfide formation which is important for the maturation of extracellular proteins.43 The host protein disulfide-isomerase family is also closely involved in pathogen antigen processing.44
In addition, L. edodes-derived polysaccharides show potential therapeutic applications in the treatment of metabolic syndromes such as hyperlipidemia.7 In the present study, the biological function of the most differential proteins in abundance was related to metabolic regulation. PTRF was found to be a mediator of lipolysis and regulates adipocyte differentiation.45,46 Carbonyl reductases are NADPH-dependent and known to be involved in the metabolism of many endogenous and xenobiotic carbonyl compounds,47 such as intermediate metabolites of sugar and lipid. FPS catalyzes the biosynthesis of terpenes, terpenoids, and sterols in the HMG-CoA reductase pathway. These observations indicate that L2 may have other biological activities except for immunostimulation. Interestingly, some of the differential proteins are related to cellular structural components. However, no literature is available on the role of mushroom polysaccharides in the modulation of cellular structural components. More studies are needed to clarify the direct and/or indirect effects of L2 on host immune responses as well as on other biological functions in order to better understand the potential health benefits of mushroom polysaccharides.
Moreover, there were 11 proteins which are markedly altered, i.e. hardly or not detectable in the partner sample. Among them, Pentraxin 3 (PTX3) could be induced in vivo from epithelial cells by lipopolysaccharides or other stimuli, and may be an important mediator for host defense and tissue damage,48 indicating the influence of the gut microbiota on the abundance of the mucosal pentraxin precursor. Mucosal pentraxin (MPTX) was found to be down-accumulated approximately 10-fold by dietary heme and up-accumulated 3-fold by calcium in rat colon. MPTX may be a marker for diet-modulated mucosal integrity.49 Down-accumulation of ATP synthase subunit beta may be related to the immune response of the lymphoid organ to pathogen infection.50 Mice immunized with protein disulfide-isomerase showed significantly higher levels of specific antibodies, IFN-gamma (IFN-γ), IL-2 and IL-4 production, corresponding to a protective immune reaction,51 and suggesting that protein disulfide-isomerase might play a role in immune responses. Glutathione S-transferase A2 and heat shock cognate 71 kDa protein may be associated with stress-generated immune responses like mercury and heavy oil exposures.52,53 Transgelin was increased upon lipopolysaccharide stimulation in alligator leukocytes.54 Although the immune responses of related proteins were observed in other animals, homologous proteins may share the same function. In general, these marked differential proteins exhibit some associations with the host immune functions. Thus, the effect of L2 on immunomodulation may also be closely related to these proteins and these warrant detailed exploration.
Further network analysis by STRING was performed to identify protein–protein interactions responsible for the acting mechanism of L2 in the gut. A separate analysis of the colon and small intestine proteins demonstrated that 2 sub-networks were enriched for a part of the differential proteins in colon tissues, and 2 modules were mapped for a part of the differential proteins from small intestine tissues, centered at Atp5b and Alb, respectively. This suggests that some of the differential proteins in abundance have direct (physical) and indirect (functional) associations. However, high resolution protein separation methods are needed for deep mapping the protein interaction network. Only 3 differential proteins were identified both in the colon and small intestine tissues (protein disulfide-isomerase, type II cytoskeletal 8, Transgelin). Most of the differential proteins showed spatial specification. These observations are similar to the characters of spatial distributions of the microbial community along the mouse gastrointestinal tract.15,33 However, when analyzing colon and small intestine proteins as a whole, a unified network was formed containing 25 differential proteins, linking the initially separated sub-networks together, and incorporating the original off-network proteins into the bigger network. This suggests that L2 can act on the colon and the small intestine separately, but in concert by regulating a site-independent and unified protein network. This hypothesis should be an interesting topic for future studies.
5 Conclusion
The current work reports the proteomic profiles of the colon and small intestine tissues from mice fed with a L. edodes-derived polysaccharide L2. In total, 40 differential proteins in abundance were identified, which were primarily involved in metabolism, binding, cellular structural components, and response to stimulus. L2 displayed different actions in the colon and small intestine for some proteins, as confirmed by western blot experiments. Network analysis revealed the existence of a unified protein–protein interaction network, the key nodes of which include Alb, Vim and Hsp90ab1. These findings may help to understand the molecular mechanisms of L2-induced bioactivity.
Declaration of interest
The authors report no declaration of interest.
Acknowledgements
This work is partially supported by the Fundamental Research Funds for the Central Universities. We greatly appreciate Dr Reshma Ramracheya, Oxford Centre for Diabetes, Endocrinology & Metabolism, University of Oxford, for improvement of writing and the reviewers for their constructive comments and suggestions.
References
- S. P. Wasser, Medicinal mushrooms as a source of antitumor and immunomodulating polysaccharides, Appl. Microbiol. Biotechnol., 2002, 60, 258–274 CrossRef CAS PubMed.
- U. Lindequist, T. H. Niedermeyer and W. D. Jülich, The pharmacological potential of mushrooms, J. Evidence-Based Complementary Altern. Med., 2005, 2, 285–299 CrossRef PubMed.
- H. Zhu, K. Sheng, E. Yan, J. Qiao and F. Lv, Extraction, purification and antibacterial activities of a polysaccharide from spent mushroom substrate, Int. J. Biol. Macromol., 2012, 50, 840–843 CrossRef CAS PubMed.
- L. Zhu, Q. Tang, S. Zhou and Y. Y. Liu,
et al., Isolation and purification of a polysaccharide from the caterpillar medicinal mushroom Cordyceps militaris (Ascomycetes) fruit bodies and its immunomodulation of RAW 264.7 macrophages, Int. J. Med. Mushrooms, 2014, 16, 247–257 CrossRef CAS PubMed.
- O. F. Seniuk, L. F. Gorovoj, G. V. Beketova and H. O. Savichuk,
et al., Anti-infective properties of the melanin-glucan complex obtained from medicinal tinder bracket mushroom, Fomes fomentarius (L.: Fr.) Fr. (Aphyllophoromycetideae), Int. J. Med. Mushrooms, 2011, 13, 7–18 CrossRef PubMed.
- S. Silva, S. Martins, A. Karmali and E. Rosa, Production, purification and characterisation of polysaccharides from Pleurotus ostreatus with antitumour activity, J. Sci. Food Agric., 2012, 92, 1826–1832 CrossRef CAS PubMed.
- P. S. Bisen, R. K. Baghel, B. S. Sanodiya and G. S. Thakur,
et al., Lentinus edodes: A macrofungus with pharmacological activities, Curr. Med. Chem., 2010, 17, 2419–2430 CrossRef CAS PubMed.
- H. H. Lee, J. S. Lee, J. Y. Cho and Y. E. Kim,
et al., Study on immunostimulating activity of macrophage treated with purified polysaccharides from liquid culture and fruiting body of Lentinus edodes, J. Microbiol. Biotechnol., 2009, 19, 566–572 CAS.
- X. F. Xu, H. D. Yan, J. Tang and J. Chen,
et al., Polysaccharides in Lentinus edodes: isolation, structure, immunomodulating activity and future prospective, Crit. Rev. Food Sci. Nutr., 2014, 54, 474–487 CrossRef CAS PubMed.
- T. Rabilloud, The whereabouts of 2D gels in quantitative proteomics, Methods Mol. Biol., 2012, 893, 25–35 CAS.
- C. Ma, S. H. Guan, M. Yang and X. Liu,
et al., Differential protein expression in mouse splenic mononuclear cells treated with polysaccharides from spores of Ganoderma lucidum, Phytomedicine, 2008, 15, 268–276 CrossRef CAS PubMed.
- Y. B. Li, R. Wang, H. L. Wu and Y. H. Li,
et al., Serum amyloid A mediates the inhibitory effect of Ganoderma lucidum polysaccharides on tumor cell adhesion to endothelial cells, Oncol. Rep., 2008, 20, 549–556 CAS.
- X. F. Xu, H. D. Yan and X. W. Zhang, Structure and immuno-stimulating activities of a new heteropolysaccharide from Lentinula edodes, J. Agric. Food Chem., 2012, 60, 11560–11566 CrossRef CAS PubMed.
- X. F. Xu, J. G. Yang, Z. Luo and X. W. Zhang,
Lentinula edodes-derived polysaccharide enhances systemic and mucosal immunity by spatial modulation of intestinal gene expression in mice, Food Funct., 2015, 6, 2068–2080 CAS.
- X. F. Xu and X. W. Zhang,
Lentinula edodes-derived polysaccharide alters the spatial structure of gut microbiota in mice, PLoS One, 2015, 10, e0115037 Search PubMed.
- E. Larsson, V. Tremaroli, Y. S. Lee and O. Koren,
et al., Analysis of gut microbial regulation of host gene expression along the length of the gut and regulation of gut microbial ecology through MyD88, Gut, 2012, 61(8), 1124–1131 CrossRef CAS PubMed.
- D. H. Zhou, Z. G. Yuan, F. R. Zhao and H. L. Li,
et al., Modulation of mouse macrophage proteome induced by Toxoplasma gondii tachyzoites in vivo, Parasitol. Res., 2011, 109, 1637–1646 CrossRef CAS PubMed.
- D. H. Zhou, F. R. Zhao, S. Y. Huang and M. J. Xu,
et al., Changes in the proteomic profiles of mouse brain after infection with cyst-forming Toxoplasma gondii, Parasites Vectors, 2013, 6, 96–102 CrossRef CAS PubMed.
- H. Mi and P. Thomas, Protein networks and pathway analysis, Methods Mol. Biol., 2009, 2563(Part 2), 123–140 Search PubMed.
- A. Franceschini, D. Szklarczyk, S. Frankild and M. Kuhn,
et al., STRING v9.1: protein-protein interaction networks, with increased coverage and integration, Nucleic Acids Res., 2013, 41, D808–D815 CrossRef CAS PubMed.
- M. Zhang, S. W. Cui, P. C. K. Cheung and Q. Wang, Antitumor polysaccharides from mushrooms: a review on their isolation process, structural characteristics and antitumor activity, Trends Food Sci. Technol., 2007, 18, 4–19 CrossRef CAS.
- G. Li, J. Chen, P. Xie and Y. Jiang,
et al., Protein expression profiling in the zebrafish (Danio rerio) embryos exposed to the microcystin-LR, Proteomics, 2011, 11, 2003–2018 CrossRef CAS PubMed.
- N. Qiu, W. Liu, M. Ma and L. Zhao,
et al., Differences between fertilized and unfertilized chicken egg white proteins revealed by 2-dimensional gel electrophoresis-based proteomic analysis, Poult. Sci., 2013, 92, 782–786 CrossRef CAS PubMed.
- J. G. Burniston, J. Kenyani, D. Gray and E. Guadagnin,
et al., Conditional independence mapping of DIGE data reveals PDIA3 protein species as key nodes associated with muscle aerobic capacity, J. Proteomics, 2014, 106, 230–245 CrossRef CAS PubMed.
- G. Lin, Y. Liu and K. M. MacLeod, Regulation of muscle creatine kinase by phosphorylation in normal and diabetic hearts, Cell. Mol. Life Sci., 2009, 66, 135–144 CrossRef CAS PubMed.
- K. L. Bennett, A. Stensballe, A. V. Podtelejnikov and M. Moniatte,
et al., Phosphopeptide detection and sequencing by matrix-assisted laser desorption/ionization quadrupole time-of-flight tandem mass spectrometry, J. Mass Spectrom., 2002, 37, 179–190 CrossRef CAS PubMed.
- J. J. Volman, J. P. Helsper, S. Wei and J. J. Baars,
et al., Effects of mushroom-derived beta-glucan-rich polysaccharide extracts on nitric oxide production by bone marrow-derived macrophages and nuclear factor-kappaB transactivation in Caco-2 reporter cells: can effects be explained by structure?, Mol. Nutr. Food Res., 2010, 54(2), 268–276 CAS.
- M. Mizuno, Y. Nishitani, T. Hashimoto and K. Kanazawa, Different suppressive effects of fucoidan and lentinan on IL-8 mRNA expression in in vitro gut inflammation, Biosci., Biotechnol., Biochem., 2009, 73, 2324–2325 CrossRef CAS PubMed.
- K. H. Wong, K. Y. Wong, H. S. Kwan and P. C. Cheung, Dietary fibers from mushroom sclerotia: 3. In vitro fermentability using human fecal microflora, J. Agric. Food Chem., 2005, 53, 9407–9412 CrossRef CAS PubMed.
- K. M. Maslowski and C. R. Mackay, Diet, gut microbiota and immune responses, Nat. Immunol., 2011, 12, 5–9 CrossRef CAS PubMed.
- L. Geurts, A. M. Neyrinck, N. M. Delzenne and C. Knauf,
et al., Gut microbiota controls adipose tissue expansion, gut barrier and glucose metabolism: novel insights into molecular targets and interventions using prebiotics, Benefic. Microbes, 2014, 5, 3–17 CrossRef CAS PubMed.
- F. Sommer and F. Bäckhed, The gut microbiota–masters of host development and physiology, Nat. Rev. Microbiol., 2013, 11, 227–238 CrossRef CAS PubMed.
- S. Gu, D. Chen, J. N. Zhang and X. Lv,
et al., Bacterial community mapping of the mouse gastrointestinal tract, PLoS One, 2013, 8, e74957 CAS.
- J. R. Goldsmith and R. B. Sartor, The role of diet on intestinal microbiota metabolism: downstream impacts on host immune function and health, and therapeutic implications, J. Gastroenterol., 2014, 49, 785–798 CrossRef CAS PubMed.
- S. Viaud, F. Saccheri, G. Mignot and T. Yamazaki,
et al., The intestinal microbiota modulates the anticancer immune effects of cyclophosphamide, Science, 2013, 342(6161), 971–976 CrossRef CAS PubMed.
- O. Rop, J. Mlcek and T. Jurikova, Beta-glucans in higher fungi and their health effects, Nutr. Rev., 2009, 67, 624–631 CrossRef PubMed.
- W. Xu, Z. Yuan and X. Gao, Immunoproteomic analysis of the antibody response obtained in mouse following vaccination with a T-cell vaccine, Proteomics, 2011, 11, 4368–4375 CrossRef CAS PubMed.
- K. M. Das and M. Bajpai, Tropomyosins in human diseases: ulcerative colitis, Adv. Exp. Med. Biol., 2008, 644, 158–167 CrossRef CAS PubMed.
- P. Bercik, E. F. Verdu, J. A. Foster and J. Macri,
et al., Chronic gastrointestinal inflammation induces anxiety-like behavior and alters central nervous system biochemistry in mice, Gastroenterology, 2010, 139, 2102–2112 CrossRef CAS PubMed.
- Y. J. Ma, A. Doni, M. O. Skjoedt and C. Honoré,
et al., Heterocomplexes of mannose-binding lectin and the pentraxins PTX3 or serum amyloid P component trigger cross-activation of the complement system, J. Biol. Chem., 2011, 286, 3405–3417 CrossRef CAS PubMed.
- C. S. Reigstad, G. O. Lunden, J. Felin and F. Bäckhed, Regulation of serum amyloid A3 (SAA3) in mouse colonic epithelium and adipose tissue by the intestinal microbiota, PLoS One, 2009, 4, e5842 Search PubMed.
- H. Liu, J. Dicksved, T. Lundh and J. E. Lindberg, Heat Shock Proteins: Intestinal gatekeepers that are influenced by dietary components and the gut microbiota, Pathogens, 2014, 3, 187–210 CrossRef PubMed.
- B. Wilkinson and H. F. Gilbert, Protein disulfide isomerase, Biochim. Biophys. Acta, 2004, 1699, 35–44 CrossRef CAS.
- B. S. Stolf, I. Smyrnias, L. R. Lopes and A. Vendramin,
et al., Protein disulfide isomerase and host-pathogen interaction, Sci. World J., 2011, 11, 1749–1761 CrossRef CAS PubMed.
- N. Aboulaich, P. C. Chui, J. M. Asara and J. S. Flier,
et al., Polymerase I and transcript release factor regulates lipolysis via a phosphorylation-dependent mechanism, Diabetes, 2011, 60, 757–765 CrossRef CAS PubMed.
- S. Perez-Diaz, L. A. Johnson, R. M. DeKroon and J. M. Moreno-Navarrete,
et al., Polymerase I and transcript release factor (PTRF) regulates adipocyte differentiation and determines adipose tissue expandability, FASEB J., 2014, 28, 3769–3779 CrossRef CAS PubMed.
- P. Malatkova, E. Maser and V. Wsol, Human carbonyl reductases, Curr. Drug Metab., 2010, 11, 639–658 CrossRef CAS PubMed.
- B. Han, M. Mura, C. F. Andrade and D. Okutani,
et al., TNF alpha-induced long pentraxin PTX3 expression in human lung epithelial cells via JNK, J. Immunol., 2005, 175, 8303–8311 CrossRef CAS.
- J. E. Drew, A. J. Farquharson, J. Keijer and L. N. Barrera, Complex regulation of mucosal pentraxin (Mptx) revealed by discrete micro-anatomical locations in colon, Biochim. Biophys. Acta, 2006, 1762, 844–848 CrossRef CAS PubMed.
- J. Zhang, F. Li, H. Jiang and Y. Yu,
et al., Proteomic analysis of differentially expressed proteins in lymphoid organ of Fenneropenaeus chinensis response to Vibrio anguillarum stimulation, Fish Shellfish Immunol., 2010, 29, 186–194 CrossRef CAS PubMed.
- H. L. Wang, Y. Q. Li, L. T. Yin and X. L. Meng,
et al., Toxoplasma gondii protein disulfide isomerase (TgPDI) is a novel vaccine candidate against toxoplasmosis, PLoS One, 2013, 8, e70884 CAS.
- J. A. Motts, D. L. Shirley, E. K. Silbergeld and J. F. Nyland, Novel biomarkers of mercury-induced autoimmune dysfunction: a cross-sectional study in Amazonian Brazil, Environ. Res., 2014, 132, 12–18 CrossRef CAS PubMed.
- K. Nakayama, S. Kitamura, Y. Murakami and J. Y. Song,
et al., Toxicogenomic analysis of immune system-related genes in Japanese flounder (Paralichthys olivaceus) exposed to heavy oil, Mar. Pollut. Bull., 2008, 57, 445–452 CrossRef CAS PubMed.
- M. Merchant, C. Kinney and P. Sanders, Differential protein expression in alligator leukocytes in response to bacterial lipopolysaccharide injection, Comp. Biochem. Physiol., Part. D: Genomics Proteomics, 2009, 4, 300–304 CrossRef PubMed.
Footnote |
† Electronic supplementary information (ESI) available. See DOI: 10.1039/c5fo00904a |
|
This journal is © The Royal Society of Chemistry 2016 |