DOI:
10.1039/C5FO00885A
(Paper)
Food Funct., 2016,
7, 390-397
Production of immunoregulatory polysaccharides from Crassostrea hongkongensis and their positive effects as a nutrition factor in modulating the effectiveness and toxicity of 5-FU chemotherapy in mice
Received
20th July 2015
, Accepted 13th October 2015
First published on 15th October 2015
Abstract
Chemotherapy is generally accompanied by undesirable side effects, such as immunosuppression and malnutrition, which reduce tolerance to cancer therapies. Prior studies have shown that immunonutrition improves the clinical outcomes of cancer patients. In this study, immunoregulatory polysaccharides from Crassostrea hongkongensis were included in a nutrition formula that was administered to S180 tumor-bearing mice in combination with 5-fluorouracil (5-FU) treatment. The C30–60% fraction of the polysaccharides was characterized as a branched polysaccharide, with a high amount of D-glucose (96.76% of the total) and the highest uronic acid and sulfate groups’ content among all of the polysaccharide fractions. The C30–60% polysaccharide fraction showed a maximal proliferative effect on RAW264.7 cells and T lymphocytes at a concentration of 0.0391 mg mL−1 and 0.0781 mg mL−1, respectively. Moreover, the combination treatment of the C30–60% polysaccharide-based nutrition formula (OPNF) with the administration of 5-FU effectively inhibited the growth of tumors and notably increased the leucocyte and lymphocyte counts in S180 tumor-bearing mice. In addition, a slight increase in the erythrocyte and hemoglobin values was observed in the mice treated with the combination of OPNF and 5-FU. These results suggest that supplementation with a C30–60%-based enteral formula would be beneficial for patients undergoing chemotherapy with 5-FU.
1. Introduction
Significant malnutrition is found in a high percentage of cancer patients.1 Malnutrition is associated with defects in immune function that may impair the host response to malignancy. The combination of malnutrition and immunosuppression makes patients highly susceptible to postoperative or chemoradiotherapy-associated infections and complications. Nutritional management for cancer patients involves more than simple weight gain or improvement in nutritional markers, it also aims to alleviate symptoms and antitumor therapy side-effects and decrease the risk of infection. Meta-analysis has shown that immunonutrition improves the clinical outcomes of cancer patients, especially reducing postoperative infections, improving wound healing and shortening the length of hospital stay.2–4
The immune system has the capacity to prevent tumor development, a function that depends on the balance between the pro-tumor and anti-tumor mediators of both the innate and adaptive immune systems.5 Within the immune system, macrophages are the primary defense cells that initiate immune responses and activate immune cells such as lymphocytes. Activated macrophages express increasing levels of class II major histocompatibility complex (MHC) molecules and costimulatory molecules that trigger the immune response and serve as effector cells. Furthermore, macrophages are unique components of the innate immune system and have a range of functions related to the activation process.6,7 Lymphocyte proliferation is an important index that evaluates the cellular and humoral immune function. Multiple dietary components may affect immune function. In particular, the important role of polysaccharides, amino acids and lipids in modulating immune functions has been recognized.8
Studies have shown that a variety of polysaccharides can act as immunomodulators, helping to stimulate the immune system so that it can eliminate tumor cells and foreign invaders more effectively. Polysaccharides represent a structurally diverse class of macromolecules, and the structural variability of polysaccharides can profoundly affect their cell-type specificity and biological activity in T cells and macrophages.9 Polysaccharides have been shown to improve the proliferation and function of intestinal intraepithelial γδT cells in tumor-bearing mice.10 The sulfate at the C-6 position of an oyster polysaccharide appears to increase effective promotion of splenic lymphocyte proliferation.11 Pacific oyster-derived polysaccharides have also been shown to modulate antigen-specific T cell responses, including antigen-induced splenocyte proliferation and T cell cytokine expression.12 Polysaccharides derived from marine organisms are typically nontoxic and free from side effects,13,14 which make them promising potential immunonutrition supplements for cancer patients. Crossostrea hongkongensis, which contains more than 20% glycogen, has been used as a dual-purpose medicine/food shellfish in South China for hundreds of years and remains quite popular in China nowadays.15 Because of the oyster's functional polysaccharides and glycoprotein, oyster extracts have been developed into functional foods and medicines for the prevention and management of chronic diseases.
In the present work, polysaccharides from the oyster C. hongkongensis were purified by graded ethanol precipitation and characterized. Their in vitro immunosuppressive activities were then screened in RAW264.7 mouse macrophages and T lymphocyte cells. The tumor response to a nutrition formula based on the oyster immunoregulatory polysaccharide and combined with 5-FU was investigated in vivo using a S180 tumor-bearing mouse model.
2. Materials and methods
2.1 Materials
C. hongkongensis was collected from an oyster culture farm in Hengqin of Zhuhai, China and was identified by Professor Zi-Niu Yu of the South China Sea Institute of Oceanology, Chinese Academy of Sciences. 5-FU was purchased from Shanghai Xudong Haipu Pharmaceutical Co., Ltd. Glycopeptides from Coriolus versicolor, which were used as a positive control, were purchased from Shanghai Xinkang Pharmaceutical Factory. The RAW 264.7 and mouse sarcoma S180 cell lines were obtained from the Laboratory Animal Medical Center of Sun Yat-Sen University, China.
2.2 Graded ethanol precipitation of polysaccharides
Fresh oyster meat was homogenized with a 6-fold volume of distilled water and then ultrasonically pretreated for 24 min under a power of 876 W and at a temperature of 49 °C. Then, the sample solution was hydrolyzed with alcalase at 3000 U per g, 55 °C, pH 8.0 for 4 h.16 The crude extract was centrifuged at 1800g for 15 min, and the supernatant was then concentrated. The polysaccharides were separated into three fractions by graded ethanol precipitation. Aliquots of ethanol were added to the hydrolysate with continuous stirring to a final concentration of 30% (v/v) ethanol, and the mixture was maintained at 4 °C for 12 h. The precipitate was recovered by centrifugation (1800g, 15 min), washed with anhydrous ethanol, acetone and ether in turns, deproteinized by the Savage method (chloroform/butanol: 5/1), and then vacuum dried to obtain the C0–30% fraction. Next, the ethanol concentration was further increased to 60% (v/v) and 90% (v/v) in the same manner to obtain the C30–60% and C60–90% fractions, respectively.17 The uronic acid content of the polysaccharides was measured by the sulfuric acid–carbazole method.18 The sulfate content was measured by the barium sulphate–gluten nephelometry method.19 The protein content was measured according to Bradford's method.20 Physicochemical properties were determined using color observations, solubility tests, Fehling's tests and iodination reactions.
2.3 Monosaccharide composition analysis
Polysaccharide hydrolysis and monosaccharide detection were performed using a method adapted from Grace et al.21 Briefly, the polysaccharides were hydrolyzed with 2.0 M H2SO4 by reflux heating for 6 h. After the supernatants were neutralized with saturated Ba(OH)2, they were collected and lyophilized. The dried sample was dissolved in 5 mL hydroxylamine hydrochloride in pyridine (14 mg mL−1) and incubated at 90 °C for 1 h. Then, the heated mixture was cooled to room temperature, and 5 mL acetic anhydride was added, and the mixture was incubated at 90 °C for 1 h. A mixture of water and chloroform was added to the sample, which was then concentrated under a N2 stream and analyzed by GC-MS.
The GC-MS analyses were performed using an Agilent 6890 Series GC System coupled with an Agilent 5973 MSD mass spectrometer with an SE-30 capillary column (15 m × 0.2 mm × 0.33 μm). The instrument was programmed to run from 100 °C to 280 °C at 10 °C per min with a hold time of 10 min. Helium (He) was used as the carrier gas. The carrier gas was set to a flow rate of 1 mL min−1, and the split ratio was set to 1
:
10. For MS detection, the ion source and transfer line temperatures were set to 230 °C, with the electron ionization (EI) mode at 70 eV, and the recording masses in the range of 29–500. Six monosaccharides, ribose, arabinose, xylose, mannose, glucose, and galactose, were used as reference substances.
2.4 Proliferative effects of the polysaccharides on T lymphocytes
Spleens collected from sacrificed mice under aseptic conditions were chopped into small pieces and passed through a fine steel mesh to obtain a homogeneous cell suspension. The cells were washed, and the lymphocytes were separated from the red blood cells and lysed with ammonium chloride (0.8%, w/v). After centrifugation, the pelleted cells were washed twice in phosphate-buffered saline (PBS) and re-suspended in RPMI-1640 complete medium. Splenocytes were seeded into four wells of a 96-well flat-bottom microtiter plate at 1 × 106 cells per mL in 100 μL complete medium. Then, 75 μL ConA (200 μg mL−1) or RPMI-1640 medium containing various concentrations of oyster polysaccharides was added, resulting in a final volume of 200 μL. The plates were incubated at 37 °C under a humidified atmosphere with 5% CO2. After 68 h, 20 μL MTT (5 mg mL−1) was added to each well, and the plates were incubated for another 4 h. The plates were centrifuged (1000g, 10 min), and the untransformed MTT was carefully removed by pipetting. To each well, 100 μL of a DMSO working solution (180 μL DMSO with 20 μL 1 N HCl) was added, and the absorbance at 570 nm was measured in a microplate reader (Thermo, USA) after 15 min. Glycopeptides from C. versicolor were used as a positive control sample. The splenocyte proliferation index (%) was calculated as (ODs/ODc-1) × 100, where ODs and ODc represent the OD value of the sample and control group, respectively.22
2.5 Proliferative effects of the polysaccharides on RAW264.7 macrophages
RAW264.7 cells were maintained in Dulbecco's modified Eagle's medium (DMEM, Invitrogen Co.) supplemented with 10% (v/v) heat-inactivated fetal bovine serum (FBS, Thermo), penicillin–streptomycin (100 IU per mL to 100 μg per mL), 2 mM glutamine, and 10 mM HEPES under a humidified atmosphere (5% CO2, 95% air) at 37 °C. The cells were fed every 2–3 days and harvested by brief treatment with 0.02% EDTA–0.25% trypsin. The cells were cultured in 96-well plates at a density of 2.5 × 105 cells per well in 100 μL medium with different final concentrations of oyster polysaccharides for 24 or 48 h at 37 °C and 5% CO2. MTT solution (20 μL; 5 mg mL−1 in phosphate buffered-saline) was added to each well 4 h prior to culture termination. Then, the supernatant was discarded, and 150 μL DMSO was added. After shaking to dissolve the formazan, the optical density at 570 nm was measured using a microplate reader (Thermo, USA). Each experimental condition was tested in triplicate.23
2.6 Antitumor effects on S180 solid tumors
Male BALB/C mice (6–8 weeks old, weighing 17–25 g, permit number: SCXK-2008-0002) were obtained from the Laboratory Animal Medical Center of Sun Yat-Sen University, China. The animals were housed in a storage room under the conditions of constant temperature (23 ± 1 °C) and relative humidity (50 ± 5%) and were maintained on a 12 h light–dark cycle (lights on at 07:00 AM) with free access to food and water until the start of the experiment. All procedures regarding animal care and use were carried out based on the guidelines of the animal ethics committee of Sun Yat-Sen University (Guangzhou, China).
Mouse sarcoma S180 cells, which were collected from the ascites of the tumor-bearing mice, were harvested and washed three times with aseptic PBS. The cells were pelleted by brief centrifugation at 3000g. The supernatant was aspirated, and the cells were resuspended in PBS at 2 × 106 cells per mL. Male BALB/C mice were used and were inoculated subcutaneously (s.c.) into the armpit at 0.2 mL per mouse to establish the S180 solid tumor model. Twenty-four hours after inoculation, the mice were randomly divided into 7 groups (10 mice per group). Then, treatments were administered to the normal and model mice as described below. The normal group (without inoculation) received 2 g per kg normal saline by gavage each day, the model group received 2 g per kg normal saline by gavage each day, the 5-FU group received intraperitoneal injections of 30 mg per kg 5-FU every other day, and the oyster polysaccharide-based nutrition fodder and the 5-FU (OPNF + 5-FU) group received 2 g per kg OPNF by gavage each day and intraperitoneal injections of 30 mg per kg 5-FU every other day. The OPNF contained 1.3 g oyster C30–60% polysaccharide powder, 1.2 g isolated soybean protein, 7.0 g soybean peptide and 8.0 g oyster peptide. The nutrition fodder and 5-FU (NF + 5-FU) group received 2 g per kg NF by gavage each day and intraperitoneal injections of 30 mg per kg 5-FU every other day. The NF contained 1.3 g maltodextrin, 1.2 g isolated soybean protein, 7.0 g soybean peptide and 8.0 g oyster peptide. The OPNF group received 2 g per kg OPNF by gavage each day, and the NF group received 2 g per kg NF by gavage each day. On day 16, after the animals were weighed and, blood was collected, the mice were sacrificed. The eyeballs were removed and placed into heparinized tubes to measure leucocyte and erythrocyte concentrations. The tumor, spleen and thymus were removed and weighed. The antitumor activity was expressed as an inhibition ratio and was calculated as:
Tumor inhibition rate = (1 − Wt/Wc) × 100%, |
where Wt is the average tumor weight of the test group and Wc is the average tumor weight of the model control group.
2.6 Statistical analysis
All experiments were repeated at least three times. The statistical values were presented as the mean ± standard deviation, and the data were analyzed by the Tukey's multiple comparison tests to evaluate significant differences. A value of p < 0.05 denoted a statistically significant difference.
3. Results
3.1 Fractionation and characteristics of polysaccharides from C. hongkongensis
The oyster hydrolysate was further precipitated by ultrasonic-assisted alcalase hydrolysis to yield three subfractions: C0–30% (13.24%, in weight), C30–60% (16.78%) and C60–90% (69.98%). The immunomodulatory activity of polysaccharides is known to be correlated with their chemical composition and physicochemical properties. The sulfate and uronic acid contents have been demonstrated to play an important role in the stimulation of the proliferation of macrophages and lymphocytes.24,25 As shown in Table 1, the C30–60% fraction exhibited the highest uronic acid and sulfate content and contained a small amount of proteins but lacked reducing sugars and starch. The analysis of the GC content of the C30–60% polysaccharides resulted in characterization as a branched polysaccharide with a high amount of D-glucose (96.76% of the total) in addition to D-mannose (2.91%), D-arabinose (0.24%), D-ribose (0.04%), D-galactose (0.04%) and D-xylose (0.01%) (Fig. 1).
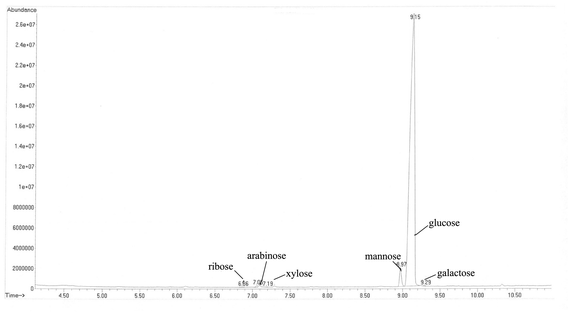 |
| Fig. 1 Monosaccharide composition analysis of the C30–60% polysaccharides. | |
Table 1 Preliminary chemical composition of the C0–30%, C30–60% and C60–90% fractions
Sample |
C0–30% |
C30–60% |
C60–90% |
Not detected.
|
Color |
White |
Brown |
Brown |
Solubility |
Water soluble |
Uronic acid (%) |
11.60 ± 0.95 |
18.06 ± 0.45 |
16.06 ± 0.90 |
Sulfate (%) |
(—)a |
1.25 ± 0.35 |
0.74 ± 0.21 |
Protein (%) |
0.47 ± 0.05 |
2.24 ± 0.09 |
4.67 ± 0.03 |
Fehling's test |
(—)a |
Iodination reaction |
(—)a |
3.2 Immunomodulatory activity of oyster polysaccharides in vitro
The polysaccharides from C. hongkongensis significantly promoted the proliferation of macrophages and splenocytes (Fig. 2). Over the range of concentrations from 0.01 to 0.08 mg mL−1, the proliferation rate of the C30–60% group of RAW 264.7 cells was significantly higher than the rate of the control group (p < 0.05, p < 0.01) and the group treated with the positive control glycopeptides from C. versicolor. The C30–60% polysaccharides had the greatest positive effect on proliferation at 0.0391 mg mL−1. When the concentration of C30–60% polysaccharides was higher than 0.0391 mg mL−1, the proliferative effect decreased (Fig. 2A). The proliferative effect of the C30–60% fraction on T lymphocytes in vitro was the similar (Fig. 2B). At a concentration of 0.0781 mg mL−1, the proliferative effect of the C30–60% fraction on T lymphocytes was maximal. In the RAW264.7 cells, a dose-dependent proliferative effect of the C60–90% fraction was observed at higher doses (0.1563–1.2500 mg mL−1), while a suppressive effect was observed at lower doses (0.0098–0.0781 mg mL−1). This effect may be related to the different molecular weights and physiochemical properties of the polysaccharides.12 Thus, the C30–60% group exhibited a greater positive effect on macrophage and lymphocyte activation in vitro.
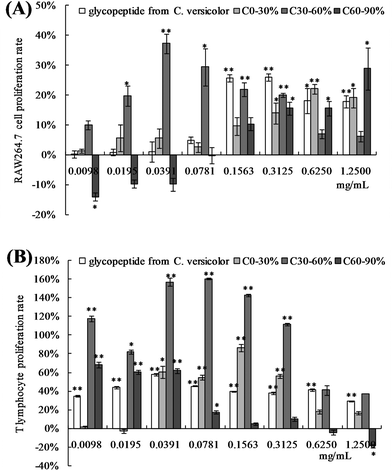 |
| Fig. 2 Proliferation effects of oyster polysaccharides on (a) raw 264.7 cells and (b) T lymphocytes. Significant differences were detected when compared with the blank control (*p < 0.05, **p < 0.01). | |
3.3 Effects of OPNF and/or 5-FU on tumor growth in S180 tumor-bearing mice
Tumor inhibition rates were calculated relative to the model control group. The results are shown in Fig. 3. Administration of 5-FU to the S180 tumor-bearing mice effectively inhibited tumor growth (p < 0.01). Interestingly, when OPNF was combined with 5-FU, a synergistic antitumor effect was observed in the model mice. The tumor inhibition rate of the OPNF + 5-FU group was 68.34%, which was 11.59% (p < 0.05) higher than that of the 5-FU group. There was no significant difference between the tumor inhibition rates of the NF + 5-FU and 5-FU alone groups. When the OPNF and NF alone groups were compared, only the OPNF group showed a significant decrease in tumor weight (p < 0.01).
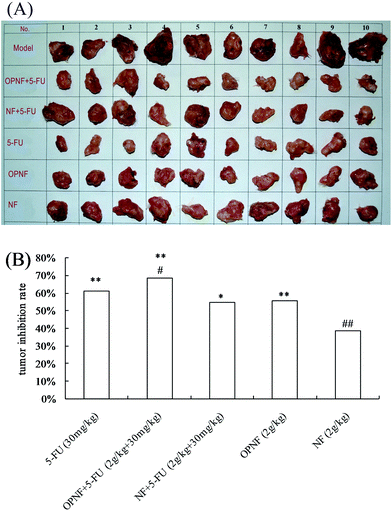 |
| Fig. 3 Effects of OPNF and/or 5-FU on tumor growth in S180 tumor-bearing mice. (a) Photo of a removed tumor. (b) Tumor inhibition rate. Significant differences were detected when compared with the model control (*p < 0.05, **p < 0.01). Significant differences were detected when compared with the 5-FU group (#p < 0.05, ##p < 0.01). | |
3.4 Effects of OPNF and/or 5-FU on spleen and thymus indices in S180 tumor-bearing mice
The spleen and thymus are among the primary immune organs and directly affect an organism's immune function. The effects of OPNF and/or 5-FU on the thymus and spleen indices in the S180 tumor-bearing mice are shown in Fig. 4. Compared to the normal and model groups, the thymus and spleen indices of the tumor-bearing mice that were treated with 5-FU were significantly decreased, suggesting that thymus and spleen function were probably impaired in these mice. Slightly decreased spleen and thymus indices were also observed in the OPNF group, but the decrease was not significant. In addition, the indices in the OPNF and NF alone groups were significantly higher than those of the 5-FU group, although the values did not significantly differ from those of the normal and model control groups. Taken together, these data indicate that the OPNF and NF enhanced the immunological function but did not obviously offset the damage to the spleen and thymus that occurred in the mice treated with 5-FU.
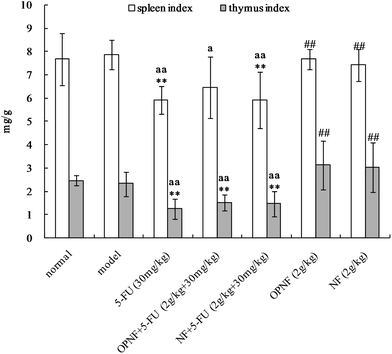 |
| Fig. 4 Effects of OPNF and/or 5-FU on the spleen and thymus indices in S180 tumor-bearing mice. Significant differences from the normal group were designated as **p < 0.01. Significant differences from the model control group were designated as ap < 0.05 and aap < 0.01. Significant differences from the 5-FU group were designated as ##p < 0.01. | |
3.5 Effects of OPNF and/or 5-FU on leucocyte and erythrocyte levels in S180 tumor-bearing mice
The effects of OPNF dietary supplementation on selected hematological parameters of the S180 tumor-bearing mice are presented in Tables 2 and 3. There was a significant increase in the leucocyte count (p < 0.01) and a significant decrease in the lymphocyte and monocyte counts (p < 0.05) in the model control group. The concentrations of erythrocytes, hemoglobin and hematocrit were also decreased in the S180 tumor-bearing mice (p < 0.05, p < 0.01), indicating that the S180 tumor-bearing mice were immunocompromised and exhibited increased inflammation and energy expenditure. However, the leucocyte and monocyte counts were significantly decreased by treatment with 5-FU alone (p < 0.01, p < 0.05), as demonstrated by the immune-suppression. However, the decreased leucocyte and lymphocyte counts induced by 5-FU were both rivaled to the high level by OPNF, compared with the model control and 5-FU groups. Moreover, the leucocyte and lymphocyte counts in the 5-FU + NF group were lower than those in the 5-FU + OPNF group (Table 2). In addition, a slight increase in the erythrocyte and hemoglobin values of the mice treated with OPNF + 5-FU was observed; these increases were not found to be statistically significant compared to the changes observed in the 5-FU groups (Table 3).
Table 2 Effects of OPNF and/or 5-FU on leucocyte subpopulations in S180 tumor-bearing mice
Group |
Leucocyte (×109 L−1) |
Lymphocyte (%) |
Neutrophil (%) |
Monocyte (%) |
Compared with the normal control group, *p < 0.05, **p < 0.01. Compared with the model control group, ap < 0.05, aap < 0.01. Compared with the 5-FU group, #p < 0.05, ##p < 0.01. |
Control |
3.69 ± 1.09 |
80.14 ± 8.81 |
19.21 ± 7.47 |
3.38 ± 0.75 |
Model |
6.44 ± 0.97** |
70.78 ± 7.38* |
22.97 ± 4.41 |
2.35 ± 0.67* |
5-FU (30 mg kg−1) |
2.74 ± 0.71*,aa |
69.11 ± 2.23** |
25.87 ± 3.79* |
1.70 ± 0.51**,a |
OPNF + 5-FU (2 g kg−1 + 30 mg kg−1) |
3.55 ± 0.88aa, # |
79.87 ± 6.46a, ## |
21.41 ± 7.13 |
2.01 ± 0.64** |
NF + 5-FU (2 g kg−1 + 30 mg kg−1) |
2.78 ± 0.87aa |
75.76 ± 7.58a, # |
24.71 ± 8.18 |
1.86 ± 0.46** |
OPNF (2 g kg−1) |
3.19 ± 0.71aa |
75.56 ± 7.55# |
25.14 ± 6.96 |
1.98 ± 0.75** |
NF (2 g kg−1) |
4.30 ± 0.62aa, ## |
64.24 ± 3.50**, a, ## |
33.29 ± 3.14**, aa, ## |
1.85 ± 0.93** |
Table 3 Effect of OPNF and/or 5-FU on erythrocyte concentrations in S180 tumor-bearing mice
Group |
Erythrocyte (×1012 L−1) |
Hemoglobin (g L−1) |
Hematocrit (%) |
Platelets (×109 L−1) |
Compared with the normal control group, *p < 0.05, **p < 0.01. Compared with the model control group, aap < 0.01. Compared with the 5-FU group, #p < 0.05, ##p < 0.01. |
Control |
9.41 ± 0.52 |
150.70 ± 6.70 |
46.35 ± 2.03 |
840.30 ± 226.15 |
Model |
8.79 ± 0.51* |
140.00 ± 7.72** |
43.58 ± 2.16** |
821.90 ± 140.86 |
5-FU (30 mg kg−1) |
8.37 ± 0.47** |
136.78 ± 7.43** |
42.56 ± 2.55** |
909.80 ± 129.11 |
OPNF + 5-FU (2 g/kg + 30 mg kg−1) |
8.71 ± 0.48** |
138.67 ± 7.28** |
42.66 ± 2.05** |
902.00 ± 164.01 |
NF + 5-FU (2 g/kg + 30 mg kg−1) |
8.17 ± 0.33**, aa |
135.11 ± 4.54** |
42.12 ± 1.26** |
875.10 ± 126.53 |
OPNF (2 g kg−1) |
9.14 ± 0.54## |
145.60 ± 8.45** |
44.94 ± 2.76 |
910.20 ± 121.78 |
NF (2 g kg−1) |
9.01 ± 0.49# |
144.60 ± 5.50*,# |
45.07 ± 1.57# |
911.80 ± 63.17 |
4. Discussion
The chemotherapeutic 5-FU, which has been used against cancer for approximately 40 years, acts in several ways; principally, 5-FU acts as a thymidylate synthase inhibitor.26 However, its anticancer activity is generally accompanied by undesirable side effects,27 including immunosuppression and malnutrition. These negative side effects reduce the response to cancer therapies and hamper further chemotherapy. Thus, it is important to determine how to protect the host from potential immunosuppression- and malnutrition-related complications. This question provides a practical opportunity for immunonutrition-based enteral nutrition to promote the tolerance of patients to chemotherapy.
Cell-mediated immunity plays an important role in antitumor defense. In addition to killing the tumor cells directly, T cells can produce a series of lymphocyte factors, which promote the proliferation and differentiation of immune cells, macrophage phagocytosis and the capacity of killing target cells. As a result, T cells play an important role in preventing tumor growth.28 Macrophages, which exhibit diverse functions, are important immune cells. Activated macrophages not only participate in both specific and non-specific immune reactions but also serve as a “bridge cell” for these two types of immune reactions.29 The results of these in vitro experiments showed that the C30–60% polysaccharide fraction exhibited significantly higher proliferative effects on T lymphocytes and RAW 264.7 macrophages than the other fractions. This result suggests that the C30–60% polysaccharides can modulate both innate and adaptive immune responses in vitro. Additionally, the immunostimulation mediated by the C30–60% fraction may be due to the higher uronic acid and sulfate content in the polysaccharide structure.30,31 Structure–activity relationships suggest that the backbone length, branching patterns, the molecular size and steric configuration all play a significant role in the bioactivity of polysaccharides. Therefore, structural characterization of the C30–60% polysaccharides is currently under further investigation.
The use of oyster polysaccharides as an immunonutrition supplement to chemotherapy is based on the premise that tumor and host responses to chemotherapy can be favorably influenced (i.e., sensitization of the tumor to chemotherapy and protection of the host against chemotherapy-related toxicity). The outcomes of the S180 tumor-bearing mice supplementated with OPNF were compared with those supplemented with the NF without oyster polysaccharides. In the present study, the antitumor rate of 5-FU was 61.24%, which confirms the reliability of the S180 tumor-bearing mouse model.32 With an antitumor rate of 68.34%, supplementation with the oyster C30–60% polysaccharide-based nutrition formula enhanced the responsiveness of the S180 tumors to 5-FU treatment.
Thus, the nutrition formula with the oyster polysaccharides reduced the severity of the immunosuppressive effect induced by 5-FU, thereby improving the immune status of the mice and their tolerance to chemotherapy. According to immunological and haematological results, the cancer-related abnormalities in the immune organs and in the peripheral blood leucocyte and erythrocyte concentrations were proved to be negatively correlated with clinical prognosis in terms of the response to 5-FU, the incidence of post-treatment infectious complications and survival. The present results also showed that the changes in immune organs and leucocyte concentration and populations that appeared prior to chemotherapy could be at least normalized by the co-treatment of OPNF with 5-FU. The obvious increase in the leucocyte count in the OPNF + 5-FU group was mainly due to a significant increase in lymphocytes in the peripheral blood. All of these results support the demonstration that OPNF improved peripheral immune system in balance in the model mouse.
Variation in the absolute number of leucocytes and other haematological parameters is attributed to differences in the nutritional state and disease status.33 The slight increase in the hemoglobin level observed in response to OPNF and 5-FU supplementation may be interpreted to mean that the oyster polysaccharides improved the utilization of digested iron and the regeneration efficiency of hemoglobin.34 Hematopoietic cells are important for the regulation of immune cell traffic and function and also provide the microenvironment that controls the survival, differentiation and migration of hematopoietic stem and progenitor cells in blood-forming tissues.35 The experimental results showed that OPNF could regulate the immune function and improve host tolerance and tumour responses to 5-FU chemotherapy in S180 tumor-bearing mice. Thus, these in vivo findings demonstrate the excellent therapeutic potential of oyster polysaccharide as an immunonutrition supplement for chemotherapy with 5-FU or similar drugs in tumor patients.
5. Conclusions
This paper suggests a promising role for the use of oyster polysaccharides as a nutritional factor in modulating the efficacy and toxicity of cancer chemotherapy. The in vitro cell models demonstrated the efficacy of the immunoenhancing activity of the C30–60% polysaccharide, which promoted the proliferation of RAW264.7 macrophages and T lymphocytes. All of these results suggest that C30–60% polysaccharides may be useful as an immune stimulant and may also have indirect antitumor effects by improving immune function. Additionally, results showed that a combination of 5-FU with OPNF could increase the tumor inhibition rate and spleen and thymus indices, as well as improve the leucocyte and erythrocyte levels in S180 tumor-bearing mice. Because the combination of OPNF and 5-FU exhibited positive antitumor activity, the oyster polysaccharides may counteract the side effects of the synthetic anticancer drug, in addition to enhancing immune system functions in the tumor-bearing mice.
Acknowledgements
This study was supported by the Project of National Key Technology Research and Development Program for the 12th Five-year Plan (no. 2012BAD33B10), the Public Science and Technology Research Funds Projects of Ocean (no. 201305018), the National Natural Science Foundation of Guangdong, China (no. 2014A030310351, no. 2014A030310338), the Innovative Development of Marine Economy Regional Demonstration Projects of Guangdong (no. SZHY2012-B01-004, no. GD2013-B03-001), and the National Science Foundation for Young Scientists of China (no. 31101271).
References
- A. Nicolini, P. Ferrari, M. C. Masoni, M. Fini, S. Pagani, O. Giampietro and A. Carpi, Biomed. Pharmacother., 2013, 67, 807–817 CrossRef CAS PubMed.
- J. W. Drover, R. Dhaliwal, L. Weitzel, P. E. Wischmeyer, J. B. Ochoa and D. K. Heyland, J. Am. Coll. Surg., 2011, 212, 385–U1401 CrossRef PubMed.
- J. A. Mauskopf, S. D. Candrilli, H. Chevrou-Severac and J. B. Ochoa, World J. Surg. Oncol., 2012, 10, 136 CrossRef PubMed.
- L. Mudge, E. Isenring and G. G. Jamieson, Dis. Esophagus, 2011, 24, 160–165 CrossRef CAS PubMed.
- E. B. Byun, N. Y. Sung, J. H. Kim, J. I. Choi, T. Matsui, M. W. Byun and J. W. Lee, Chem-Biol. Interact., 2010, 186, 90–95 CrossRef CAS PubMed.
- C. G. Kumar and P. Sujitha, Enzyme Microb. Technol., 2014, 55, 113–120 CrossRef CAS PubMed.
- D. O. Adams and T. A. Hamilton, Annu. Rev. Immunol., 1984, 2, 283–318 CrossRef CAS PubMed.
- P. Casas-Rodera, C. Gomez-Candela, S. Benitez, R. Mateo, M. Armero, R. Castillo and J. A. Culebras, Nutr. Hosp., 2008, 23, 105–110 CAS.
- S. C. Jeong, Y. T. Jeong, B. K. Yang and C. H. Song, J. Biochem. Mol. Biol., 2006, 39, 84–90 CrossRef CAS.
- S. Y. Sun, K. Zheng, H. Y. Zhao, C. Lu, B. Liu, C. Y. Yu, G. Zhang, Z. X. Bian, A. P. Lu and X. J. He, Molecules, 2014, 19, 15224–15236 CrossRef PubMed.
- J. F. Yang, B. W. Zhu, J. Zheng, L. M. Sun, D. Y. Zhou, X. P. Dong and C. X. Yu, Carbohydr. Polym., 2013, 94, 301–308 CrossRef CAS PubMed.
- L. Q. Sun, L. Wang and Y. Zhou, Carbohydr. Polym., 2012, 87, 1206–1210 CrossRef CAS.
- R. Pallela, Adv. Food Nutr. Res., 2014, 73, 183–195 Search PubMed.
- S. N. Fedorov, S. P. Ermakova, T. N. Zvyagintseva and V. A. Stonik, Mar. Drugs, 2013, 11, 4876–4901 CrossRef CAS PubMed.
- K. Lam and B. Morton, Aguaculture, 2003, 228, 1–13 CAS.
- B. N. Cai, J. Y. Pan, P. Wan, D. K. Chen, S. J. Long and H. L. Sun, Prep. Biochem. Biotechnol., 2014, 44, 708–724 CrossRef CAS PubMed.
- K. Swennen, C. M. Courtin, B. Van Der Bruggen, C. Vandecasteele and J. A. Delcour, Carbohydr. Polym., 2005, 62, 283–292 CrossRef CAS.
- T. Bitter and H. M. Muir, Anal. Biochem., 1962, 4, 330–334 CrossRef CAS.
- D. P. Cui, M. Z. Liu, B. Zhang, H. H. Gong and Y. H. Bi, Starch-Starke, 2011, 63, 354–363 CrossRef CAS.
- M. M. Bradford, Anal. Biochem., 1976, 72, 248–254 CrossRef CAS.
- O. M. Grace, A. Dzajic, A. K. Jager, N. T. Nyberg, A. Onder and N. Ronsted, Phytochemistry, 2013, 93, 79–87 CrossRef CAS PubMed.
- T. Mosmann, J. Immunol. Methods, 1983, 65, 55–63 CrossRef CAS.
- P. L. Li, X. K. Zhang, Y. N. Cheng, J. Li, Y. L. Xiao, Q. Zhang, A. Z. Zong, C. Q. Zhong and F. S. Wang, Carbohydr. Polym., 2014, 102, 852–861 CrossRef CAS PubMed.
- L. P. Teng, H. T. Fu, M. Wang, C. Deng, Z. X. Song and J. H. Chen, Carbohydr. Polym., 2015, 115, 643–650 CrossRef CAS PubMed.
- M. Cho, D. J. Lee, J. K. Kim and S. You, Carbohydr. Polym., 2014, 113, 507–514 CrossRef CAS PubMed.
- C. E. Myers, Pharmacol. Rev., 1981, 33, 1–15 CAS.
- T. Ouchi, H. Yuyama and O. Vogl, Makromol. Chem., Rapid. Commun, 1985, 6, 815–819 CrossRef CAS.
- K. D. Kim, S. C. Choi, A. Kim, Y. K. Choe, I. S. Choe and J. S. Kim, Int. Immunopharmacol., 2001, 1, 2117–2129 CrossRef CAS.
- Y. Chen, J. B. Tang, X. K. Wang, F. X. Sun and S. J. Liang, Int. J. Biol. Macromol., 2012, 50, 844–848 CrossRef CAS PubMed.
- H. R. Park, H. S. Lee, S. Y. Cho, Y. S. Kim and K. S. Shin, Int. J. Mol. Med., 2013, 31, 361–368 CAS.
- D. Flamm and W. Blaschek, Planta Med., 2014, 80, 1009–1016 CrossRef CAS PubMed.
- N. Takiguchi, N. Saito, M. Nunomura, K. Kouda, K. Oda, N. Furuyama and N. Nakajima, Cancer Chemother. Pharmacol., 2001, 47, 11–14 CrossRef CAS PubMed.
- D. D. Dias, M. V. De Stefani, C. M. Ferreira, F. M. Franca, M. J. T. Ranzani-Paiva and A. A. Santos, Aquacult. Res., 2010, 41, 1064–1071 Search PubMed.
- A. Haro, I. Lopez-Aliaga, F. Lisbona, M. Barrionuevo, M. J. M. Alferez and M. S. Campos, J. Agric. Food Chem., 2000, 48, 5715–5722 CrossRef CAS.
- L. H. Cary, D. Noutai, R. E. Salber, M. S. Williams, B. F. Ngudiankama and M. H. Whitnall, Radiat. Res., 2014, 181, 592–604 CrossRef CAS PubMed.
|
This journal is © The Royal Society of Chemistry 2016 |