DOI:
10.1039/C5FO00853K
(Paper)
Food Funct., 2016,
7, 104-109
Phenolic compounds from red wine and coffee are associated with specific intestinal microorganisms in allergic subjects
Received
14th July 2015
, Accepted 21st September 2015
First published on 25th September 2015
Abstract
The dietary modulation of gut microbiota, suggested to be involved in allergy processes, has recently attracted much interest. While several studies have addressed the use of fibres to modify intestinal microbial populations, information about other components, such as phenolic compounds, is scarce. The aim of this work was to identify the dietary components able to influence the microbiota in 23 subjects suffering from rhinitis and allergic asthma, and 22 age- and sex-matched controls. The food intake was recorded by means of an annual food frequency questionnaire. Dietary fibre tables were obtained from Marlett et al., and the Phenol-Explorer database was used to assess the phenolic compound intake. The quantification of microbial groups was performed using an Ion Torrent 16S rRNA gene-based analysis. The results showed a direct association between the intake of red wine, a source of stilbenes, and the relative abundance of Bacteroides, and between the intake of coffee, rich in phenolic acids, and the abundance of Clostridium, Lactococcus and Lactobacillus genera. Despite epidemiological analyses not establishing causality, these results support the association between polyphenol-rich beverages and faecal microbiota in allergic patients.
Introduction
Large amounts of data have suggested the involvement of intestinal bacteria in the initiation and amplification of inflammatory processes, allergies and autoimmune diseases.1 In the last few years, there has been increasing interest in the study of gut microbiota, using high throughput techniques, in order to establish associations between the gut microbes and these pathologies.2,3 Allergy is a disorder of the immune system characterized by a hypersensitive reaction induced by certain types of antigens referred to as allergens. Lifestyle changes in western countries may be interfering in the mutualistic relationship between bacteria and host, leading to an increase in the incidence of this disease.4 Although it has been proposed that some food components, such as probiotics, prebiotics and antioxidants, are critical players in the correct maintenance of the immune system, their association with the microbiota in immunological disorders has not yet been adequately described.5 Apart from probiotics and prebiotics, other bioactive compounds from the diet, such as phenolic compounds, are able to modulate intestinal microbiota.6 Evidence from animal and human studies has shown that supplementing a diet with polyphenol-rich food, such as red wine,7 tea,8 cocoa9 or blueberries,10,11 produces modifications in the intestinal bacterial populations. Despite the unclear impact of these microbial changes on health, polyphenols have shown promising results in different trials with animal models for allergy12 and autoimmunity.13 It could be considered that some of the potential health effects of polyphenols on these pathologies may be due to their impact on the gut microbiota composition due to the microbial bio-conversion of polyphenolic compounds into other bioactive compounds with more potent anti-oxidant and/or anti-inflammatory activities.14
Given this evidence, the aim of our work was the identification of the dietary components associated with the faecal microbiota in samples from allergic patients. The data resulting from this work could be useful for generating hypotheses that can be used, in the future, for the design of interventional studies aimed to test the effect of specific diets on the symptoms or the course of this disease or for the design of new functional foods targeted at this group.
Subjects and methods
Participants
Twenty-three subjects suffering from rhinitis and allergic asthma were randomly selected according to the clinical criteria recommended by the European Community Respiratory Health Survey,15 functional criteria (spirometry and bronchial challenge tests with methacholine) and immunological criteria (the determination of specific IgE to some key antigens and positive cutaneous tests for those key antigens). Subjects diagnosed as having autoimmune diseases, inflammatory bowel disease (IBD) or other diseases known to affect the intestinal function, as well as subjects who had undergone medical treatment with oral corticoids, immunosuppressive agents, monoclonal antibodies, antibiotics or immunotherapy during the previous 6 months were not considered for this study. Twenty-two age- and sex-matched subjects from the same population were recruited as controls.
Ethical approval for this study (reference code AGL2010-14952; grant title “Towards a better understanding of gut microbiota functionality in some immune disorders”) was obtained from the Bioethics Committee of CSIC (Consejo Superior de Investigaciones Científicas) and from the Regional Ethics Committee for Clinical Research (Servicio de Salud del Principado de Asturias) in compliance with the Declaration of Helsinki. All determinations were performed with fully informed written consent from all participants involved in the study.
Nutritional assessment
The dietary intake of the previous year was assessed by means of a semi-quantitative FFQ referring to 160 items. During a personal interview, the subjects were asked item-by-item whether they usually ate a certain type of food and, if so, how much they usually ate. For this purpose, 3 different serving sizes of each cooked food were presented in pictures to the participants, so that they could choose from up to 7 serving sizes (from “less than the small one” to “more than the large one”). For some of the foods consumed, the amounts were recorded in household units, as a volume, or measured with a ruler. Special attention was paid to cooking practices and the number and amount of ingredients used in each recipe, as well as questions concerning menu preparation (e.g., type of oil, type of milk used) and other relevant information for the study, such as the consumption of fruit skin. The food intake was analysed to determine the energy content using the nutrient Food Composition Tables developed by CESNID,16 and the dietary fibre (total and subtypes) from the Marlett food composition tables,17 and the phenolic compound content in foods was completed using the Phenol Explorer database.18
Anthropometric measurements
The body mass index (BMI) was calculated using the formula: weight (kg)/height (m)2. The height was measured using a stadiometer with an accuracy of ±1 mm (Año-Sayol, Barcelona, Spain). The subjects stood barefoot, in an upright position and with the head positioned in the Frankfort horizontal plane. The weight was measured on a scale with an accuracy of ±100 g (Seca, Hamburg, Germany).
Microbiological analyses
Faeces were collected in an interval of 7 days after the nutritional interviews. Fresh faecal material (between 10 and 50 g per person) was collected in a sterile container and immediately manipulated and homogenized within a maximum of 3 h after defecation. During the waiting period, from defecation to homogenization, the samples were kept at 4 °C. Thirty millilitres of RNAlater solution (Applied Biosystems, Foster City, CA) was added to 10 g of the sample, and the mixture was homogenized in a sterile bag, using a Stomacher apparatus (IUL Instruments, Barcelona, Spain) with three cycles at high speed, 1 min per cycle. The homogenized samples were then stored at −80 °C until use.
Faecal DNA extraction, 16S rRNA amplification sequencing of 16S rRNA gene-based amplicons and the sequence-based microbiota analysis were performed according to Hevia et al.19 The raw sequences reported in this article have been deposited in the NCBI Short Read Archive (SRA) (study accession number: SRP028162).
Statistical analysis
The statistical analysis was performed using IBM-SPSS version 19.0 (SPSS-Inc., Chicago). For descriptive purposes, the mean values were presented on untransformed variables. A linear regression analysis was used to investigate the association between the intake of dietary fibre (total and subtypes) and the classes of phenolic compounds with faecal microbial genera. We also introduced sex, energy intake and age as covariates. The main food sources of the dietary components previously related to microbiota were selected and placed in a multiple stepwise regression analysis to explore whether their association with microbial groups remained independent from covariates and other related variables included in the model. The statistical parameters employed were β (the standardized regression coefficient) and R2 (the coefficient of multiple determinations). The conventional probability value for significance (0.05) was used in the interpretation of the results.
Results
The general characteristics of the sample, the mean intake of energy, dietary fibre (total and subtypes) and polyphenol classes in the allergic patients and the controls are compared in Table 1. No significant differences were found for any of the variables under study, with the exception of the lignan intake, which was higher in the control group.
Table 1 General description of the studied variables in the allergic patients and the controls
|
Allergic (N = 23) |
Control (N = 22) |
Multivariate analysis adjusted by age and gender.
Multivariate analysis adjusted by energy intake. The results are presented as an estimated marginal mean ± SD and percentage (%). * p ≤ 0.05.
|
Age (year) |
39.39 ± 11.28 |
39.18 ± 9.50 |
Male sex (%) |
43.5 |
31.8 |
BMI (kg m−2) |
26.27 ± 3.94 |
25.00 ± 3.63 |
Energya (kcal d−1) |
1995.80 ± 429.14 |
2187.52 ± 565.21 |
Total fibrea,b (g d−1) |
15.85 ± 6.88 |
17.53 ± 8.05 |
Soluble fibrea,b (g d−1) |
2.65 ± 1.24 |
2.62 ± 0.96 |
Insoluble fibrea,b (g d−1) |
13.21 ± 5.72 |
14.91 ± 7.14 |
|
Phenolic compounds:
|
Flavonoidsa,b (mg d−1) |
428.32 ± 259.88 |
383.39 ± 350.66 |
Phenolic acidsa,b (mg d−1) |
333.21 ± 210.46 |
307.37 ± 262.16 |
Lignansa,b (mg d−1) |
0.78 ± 0.23 |
1.04 ± 0.50* |
Stilbenesa,b (mg d−1) |
1.59 ± 2.79 |
0.63 ± 0.77 |
The results from the linear regression analysis between the intake of dietary components and microbial genera, in patients and controls, are presented in Tables 2 and 3. Positive associations were identified between the intake of total and insoluble fibre and the relative abundance of Clostridium in allergic subjects (Table 2). Also, Clostridium, Lactococcus and Lactobacillus, were directly associated with phenolic acids, and Bacteroides with stilbenes (Table 3). Given the high correlation between the phenolic compounds and fibres from the foods, an additional stepwise regression analysis was conducted to explore the relative importance of the total and insoluble fibre and phenolic acid intake on Clostridium. The phenolic acid intake was found to be an independent contributor to this microbial group (R2 = 0.338; β = 0.581; p = 0.004; data not shown).
Table 2 Linear regression analysis between the dietary intake of fibre (total and subtypes) and the dominant microbial genera, in patients with an allergy and controlsa
|
|
Total fibreb |
Soluble fibreb |
Insoluble fibreb |
A = Allergy (N = 23); C = Control (N = 22).
Derived from a linear regression analysis including age, sex and energy intake as covariates. The results are expressed as β (standardized regression coefficient). Units: microbial genera (%), dietary components (g d−1).* p ≤ 0.05.
|
Bacteroides
|
A |
−0.238 |
−0.228 |
−0.229 |
C |
0.135 |
0.262 |
0.117 |
Bifidobacterium
|
A |
−0.022 |
0.006 |
−0.028 |
C |
−0.178 |
−0.220 |
−0.171 |
Blautia
|
A |
−0.272 |
−0.116 |
−0.301 |
C |
−0.023 |
−0.055 |
−0.018 |
Lactococcus
|
A |
0.099 |
−0.107 |
0.149 |
C |
−0.067 |
−0.091 |
−0.064 |
Lactobacillus
|
A |
0.564 |
0.252 |
0.549 |
C |
−0.029 |
−0.039 |
−0.028 |
Clostridium
|
A |
0.777* |
0.524 |
0.809* |
C |
−0.290 |
−0.287 |
−0.289 |
Faecalibacterium
|
A |
−0.488 |
−0.516 |
−0.459 |
C |
−0.334 |
−0.377 |
−0.327 |
Streptococcus
|
A |
−0.178 |
−0.294 |
−0.139 |
C |
−0.021 |
−0.063 |
−0.016 |
Table 3 Linear regression analysis between the dietary intake of phenolic compounds and the microbial genera in patients with an allergy and controlsa
|
|
Flavonoidsb |
Phenolic acidsb |
Lignansb |
Stilbenesb |
A = Allergy (N = 23); C = Control (N = 22).
Derived from a linear regression analysis including age, sex and energy intake as covariates. The results are expressed as β (standardized regression coefficient). Units: microbial genera (%), dietary components (mg d−1).* p ≤ 0.05.
|
Bacteroides
|
A |
−0.037 |
−0.333 |
0.073 |
0.631* |
C |
0.093 |
0.047 |
−0.314 |
0.184 |
Bifidobacterium
|
A |
−0.443 |
0.146 |
0.053 |
−0.023 |
C |
−0.300 |
−0.265 |
−0.240 |
−0.038 |
Blautia
|
A |
−0.141 |
−0.136 |
−0.024 |
0.317 |
C |
0.106 |
−0.006 |
−0.166 |
0.202 |
Lactococcus
|
A |
−0.031 |
0.635* |
−0.015 |
−0.193 |
C |
−0.155 |
−0.159 |
−0.173 |
−0.240 |
Lactobacillus
|
A |
0.162 |
0.567* |
−0.250 |
−0.349 |
C |
−0.115 |
−0.005 |
−0.521 |
−0.098 |
Clostridium
|
A |
0.125 |
0.630* |
−0.150 |
0.024 |
C |
−0.067 |
−0.090 |
0.109 |
−0.005 |
Faecalibacterium
|
A |
0.229 |
0.096 |
0.094 |
0.294 |
C |
−0.111 |
−0.139 |
0.570 |
−0.082 |
Streptococcus
|
A |
−0.211 |
0.289 |
−0.151 |
−0.272 |
C |
−0.173 |
0.203 |
−0.105 |
0.011 |
With the aim of exploring the associations observed in allergic subjects, the main food sources of phenolic acids and stilbenes were calculated (Fig. 1). Coffee, identified as one of the top contributors of phenolic acids, was found to be an independent contributor to Lactococcus, Lactobacillus and Clostridium variation. Also, red wine, accounting for 95% of the intake of stilbenes, was positively associated with the relative abundance of Bacteroides in faeces (Table 4).
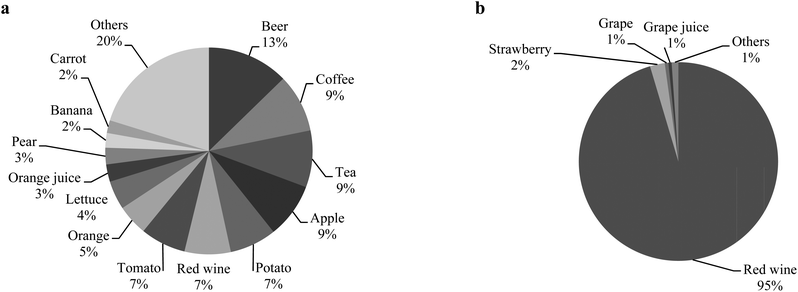 |
| Fig. 1 (a) Main food sources of phenolic acids in allergic subjects. (b) Main food sources of stilbenes in allergic subjects. | |
Table 4 Multiple stepwise regression analysis for the prediction of the bacterial genera relative abundance according to the intake of the main food sources of phenolic acids and stilbenes in allergic patientsa
|
Predictors |
Intake (g d−1) |
R
2
|
β
|
p
|
(N = 23) β: standardized regression coefficient; and R2: coefficient of multiple determinations.
Variables included in the model: age, gender, and energy, red wine, strawberry, grape and grape juice intake.
Variables included in the model: age, gender, and energy, beer, coffee, tea, apple, potato, red wine, tomato, orange, lettuce, orange juice, pear, banana and carrot intake. Only significant results are presented.
|
Bacteroides
|
Red wine |
45.34 ± 79.64 |
0.325 |
0.570 |
0.004 |
Lactococcus
|
Coffee |
60.65 ± 56.08 |
0.434 |
0.659 |
0.001 |
Lactobacillus
|
Coffee |
|
0.221 |
0.470 |
0.024 |
Clostridium
|
Coffee |
|
0.336 |
0.579 |
0.004 |
Discussion
The importance of a well-balanced colonic microbiota as a key factor in the modulation of human immunity has been more and more recognized in the last few years. Our results represent a first step in broadening the knowledge of the association between diet and microbiota in allergic patients, supporting the interaction between phenolic compounds and microbiota, and pointing to a specificity between them, to the extent that only certain microbial groups have been associated with the intake of these compounds, and because the observed associations in allergic patients were not extrapolated to the controls. Though a possible explanation could be the existence of differences in the intake of these compounds, we have not found any such difference, except for lignans which represented a low proportion of the total polyphenol intake and were not associated with any microbial genera. Thus, it seems more probable that the intra-group variability in the microbiota composition may involve the different diet-microbiota associations observed in the allergic subjects with respect to those of the controls.20,21
From all the evaluated dietary components previously associated with microbiota,22,23 phenolic acids and stilbenes were independently associated with some bacterial genera in the allergic patients. Despite the fact that the benefits of increasing the levels of Lactococcus, Lactobacillus, Clostridium and Bifidobacterium in allergic patients are not well documented, studies using animal models have proposed that the administration of some of these bacteria is able to modulate the allergic response, by means of T cell response regulation.24 In relation to this, it has been shown that the oral administration of a mix of several Clostridium strains attenuated the disease in a mice model for allergic diarrhoea through the activation of T regulatory cells.25
Although a positive association between the intake of fibre and Clostridium was also detected, in accordance with the scientific evidence about the interaction of this component with microbiota modulation,26 this appeared to be linked to phenolic acid consumption, since this association disappeared when the model was controlled by these phenolic compounds. In this regard, the nutritional assessment of the whole diet, carried out in this work, may have some advantages with respect to interventional studies, since the mixture of phenolic compounds provided by the diet, together with other dietary components contained in the phenol-containing foods, such as fibres, may improve the faecal environment, interacting with the behaviour of some bacterial groups.27
In spite of the low coffee intake in our samples, in comparison with that in other European countries (mean 60.7 ml d−1vs. 270 ml d−1),28 our results pointed to a positive association between this beverage and Lactococcus, Lactobacillus and Clostridium. The effect of coffee on intestinal microbiota is not yet clear. Results from an animal model indicate that this drink could limit the growth of some bacterial groups, such as Clostridium and Escherichia coli and, at the same time, encourage others, such as Bifidobacterium.29 This bifidogenic effect of coffee has also been found in interventional studies with humans,30 in accordance with our results. However, given the nature of this study, we are not able to analyse factors such as the variety of coffee, or its degree of roasting or processing, that could have an impact on its polyphenol content.31
In relation to red wine, it has been suggested that the intake of one of its major stilbenes, resveratrol, could prevent the development of some allergies.32 Therefore, apart from the antioxidant, anti-inflammatory and anti-allergic properties widely described for red wine phenolics,33–35 our results support a potential role for this beverage in microbiota modulation, by means of its association with Bacteroides, as has previously been suggested.7 At this point, it should be taken into account that the statistical power of our study may be limited by the relatively small sample size, and that the intake of phenolic compounds in the sample could be insufficient, when compared with that in interventional studies, to have an impact on other members of the intestinal microbiota. Longitudinal studies considering the changes in the microbiota structure from the first ages of allergic individuals could be interesting to complement this work.
Despite epidemiological analyses not establishing causality, these results support the association between polyphenol-rich beverages, such as coffee and red wine, on faecal microbiota in allergic patients. These descriptive results will be useful for future research focused on the relationship between the diet and microbiota, although further investigation is needed in order to corroborate these data before making dietary recommendations.
Conflict of interest
The authors declare no conflict of interest.
Acknowledgements
This study was financed by the European Union FEDER funds and the Spanish Plan Nacional de I+D (grant AGL2010-14952). Adriana Cuervo is in receipt of a grant from the Alimerka Foundation. We show our greatest gratitude to all the volunteers participating in the study.
References
- F. Sommer and F. Backhed, The gut microbiota–masters of host development and physiology, Nat. Rev. Microbiol., 2013, 11(4), 227–238 CrossRef CAS PubMed.
- P. J. Turnbaugh, V. K. Ridaura, J. J. Faith, F. E. Rey, R. Knight and J. I. Gordon, The effect of diet on the human gut microbiome: a metagenomic analysis in humanized gnotobiotic mice, Sci. Transl. Med., 2009, 1(6), 6ra14 CrossRef PubMed.
- H. Sokol, B. Pigneur, L. Watterlot, O. Lakhdari, L. G. Bermudez-Humaran, J. J. Gratadoux, S. Blugeon, C. Bridonneau, J. P. Furet, G. Corthier, C. Grangette, N. Vasquez, P. Pochart, G. Trugnan, G. Thomas, H. M. Blottiere, J. Dore, P. Marteau, P. Seksik and P. Langella, Faecalibacterium prausnitzii is an anti-inflammatory commensal bacterium identified by gut microbiota analysis of Crohn disease patients, Proc. Natl. Acad. Sci. U. S. A., 2008, 105(43), 16731–16736 CrossRef CAS PubMed.
- H. Okada, C. Kuhn, H. Feillet and J. F. Bach, The ‘hygiene hypothesis’ for autoimmune and allergic diseases: an update, Clin. Exp. Immunol., 2010, 160(1), 1–9 CrossRef CAS PubMed.
- A. M. Faria, A. C. Gomes-Santos, J. L. Goncalves, T. G. Moreira, S. R. Medeiros, L. P. Dourado and D. C. Cara, Food components and the immune system: from tonic agents to allergens, Front. Immunol., 2013, 4, 102 Search PubMed.
- A. Cuervo, L. Valdes, N. Salazar, C. G. de los Reyes-Gavilan, P. Ruas-Madiedo, M. Gueimonde and S. Gonzalez, Pilot study of diet and microbiota: interactive associations of fibers and polyphenols with human intestinal bacteria, J. Agric. Food Chem., 2014, 62(23), 5330–5336 CrossRef CAS PubMed.
- M. I. Queipo-Ortuno, M. Boto-Ordonez, M. Murri, J. M. Gomez-Zumaquero, M. Clemente-Postigo, R. Estruch, D. F. Cardona, C. Andres-Lacueva and F. J. Tinahones, Influence of red wine polyphenols and ethanol on the gut microbiota ecology and biochemical biomarkers, Am. J. Clin. Nutr., 2012, 95(6), 1323–1334 CrossRef CAS PubMed.
- H. C. Lee, A. M. Jenner, C. S. Low and Y. K. Lee, Effect of tea phenolics and their aromatic fecal bacterial metabolites on intestinal microbiota, Res. Microbiol., 2006, 157(9), 876–884 CrossRef CAS PubMed.
- M. Massot-Cladera, T. Perez-Berezo, A. Franch, M. Castell and F. J. Perez-Cano, Cocoa modulatory effect on rat faecal microbiota and colonic crosstalk, Arch. Biochem. Biophys., 2012, 527(2), 105–112 CrossRef CAS PubMed.
- S. Guglielmetti, D. Fracassetti, V. Taverniti, B. C. Del, S. Vendrame, D. Klimis-Zacas, S. Arioli, P. Riso and M. Porrini, Differential modulation of human intestinal bifidobacterium populations after consumption of a wild blueberry (Vaccinium angustifolium) drink, J. Agric. Food Chem., 2013, 61(34), 8134–8140 CrossRef PubMed.
- S. Vendrame, S. Guglielmetti, P. Riso, S. Arioli, D. Klimis-Zacas and M. Porrini, Six-week consumption of a wild blueberry powder drink increases bifidobacteria in the human gut, J. Agric. Food Chem., 2011, 59(24), 12815–12820 CrossRef CAS PubMed.
- A. Singh, S. Holvoet and A. Mercenier, Dietary polyphenols in the prevention and treatment of allergic diseases, Clin. Exp. Allergy, 2011, 41(10), 1346–1359 CrossRef PubMed.
- L. J. Schoenroth, D. A. Hart, K. M. Pollard and M. J. Fritzler, The effect of the phytoestrogen coumestrol on the NZB/W F1 murine model of systemic lupus, J. Autoimmun., 2004, 23(4), 323–332 CrossRef CAS PubMed.
- L. M. Bode, D. Bunzel, M. Huch, G. S. Cho, D. Ruhland, M. Bunzel, A. Bub, C. M. Franz and S. E. Kulling, In vivo and in vitro metabolism of trans-resveratrol by human gut microbiota, Am. J. Clin. Nutr., 2013, 97(2), 295–309 CrossRef CAS PubMed.
- P. G. Burney, C. Luczynska, S. Chinn and D. Jarvis, The European Community Respiratory Health Survey, Eur. Respir. J., 1994, 7(5), 954–960 CrossRef CAS PubMed.
-
Centro de Enseñanza Superior de Nutrición Humana y Dietética (CESNID), Tablas de composición de alimentos por medidas caseras de consumo habitual en España, McGraw-Hill: Publicaciones y Ediciones de la Universidad de Barcelona, Barcelona, 2008 Search PubMed.
- J. A. Marlett and T. F. Cheung, Database and quick methods of assessing typical dietary fiber intakes using data for 228 commonly consumed foods, J. Am. Diet. Assoc., 1997, 97(10), 1139–1148 CrossRef CAS PubMed.
- V. Neveu, J. Perez-Jimenez, F. Vos, V. Crespy, C. L. du, L. Mennen, C. Knox, R. Eisner, J. Cruz, D. Wishart and A. Scalbert, Phenol-Explorer: an online comprehensive database on polyphenol contents in foods, Database, 2010, 2010, bap024 CrossRef CAS PubMed.
- A. Hevia, C. Milani, P. López, A. Cuervo, S. Arboleya, S. Duranti, F. Turroni, S. González, A. Suárez, M. Gueimonde, M. Ventura, B. Sánchez and A. Margolles, Intestinal dysbiosis associated with Systemic Lupus Erythematosu, mBio, 2014, 5(5), e01548–e01514 CrossRef CAS PubMed.
- B. Cerda, F. A. Tomas-Barberan and J. C. Espin, Metabolism of antioxidant and chemopreventive ellagitannins from strawberries, raspberries, walnuts, and oak-aged wine in humans: identification of biomarkers and individual variability, J. Agric. Food Chem., 2005, 53(2), 227–235 CrossRef CAS PubMed.
- G. Gross, D. M. Jacobs, S. Peters, S. Possemiers, D. J. van, E. E. Vaughan and T. van de Wiele, In vitro bioconversion of polyphenols from black tea and red wine/grape juice by human intestinal microbiota displays strong interindividual variability, J. Agric. Food Chem., 2010, 58(18), 10236–10246 CrossRef CAS PubMed.
- U. Etxeberria, A. Fernandez-Quintela, F. I. Milagro, L. Aguirre, J. A. Martinez and M. P. Portillo, Impact of polyphenols and polyphenol-rich dietary sources on gut microbiota composition, J. Agric. Food Chem., 2013, 61(40), 9517–9533 CrossRef CAS PubMed.
- I. B. Jeffery and P. W. O'Toole, Diet-microbiota interactions and their implications for healthy living, Nutrients, 2013, 5(1), 234–252 CrossRef CAS PubMed.
- M. M. Kosiewicz, A. L. Zirnheld and P. Alard, Gut microbiota, immunity, and disease: a complex relationship, Front. Microbiol., 2011, 2, 180 Search PubMed.
- K. Atarashi, T. Tanoue, K. Oshima, W. Suda, Y. Nagano, H. Nishikawa, S. Fukuda, T. Saito, S. Narushima, K. Hase, S. Kim, J. V. Fritz, P. Wilmes, S. Ueha, K. Matsushima, H. Ohno, B. Olle, S. Sakaguchi, T. Taniguchi, H. Morita, M. Hattori and K. Honda, Treg induction by a rationally selected mixture of Clostridia strains from the human microbiota, Nature, 2013, 500(7461), 232–236 CrossRef CAS PubMed.
- M. Schantz, T. Erk and E. Richling, Metabolism of green tea catechins by the human small intestine, Biotechnol. J., 2010, 5(10), 1050–1059 CrossRef CAS PubMed.
- S. Sembries, G. Dongowski, G. Jacobasch, K. Mehrlander, F. Will and H. Dietrich, Effects of dietary fibre-rich juice colloids from apple pomace extraction juices on intestinal fermentation products and microbiota in rats, Br. J. Nutr., 2003, 90(3), 607–615 CrossRef CAS PubMed.
- V. K. Dik, H. B. Bueno-de-Mesquita, M. G. Van Oijen, P. D. Siersema, C. S. Uiterwaal, C. H. Van Gils, F. J. Van Duijnhoven, S. Cauchi, L. Yengo, P. Froguel, K. Overvad, B. H. Bech, A. Tjonneland, A. Olsen, M. C. Boutron-Ruault, A. Racine, G. Fagherazzi, T. Kuhn, D. Campa, H. Boeing, K. Aleksandrova, A. Trichopoulou, E. Peppa, E. Oikonomou, D. Palli, S. Grioni, P. Vineis, R. Tumino, S. Panico, P. H. Peeters, E. Weiderpass, D. Engeset, T. Braaten, M. Dorronsoro, M. D. Chirlaque, M. J. Sanchez, A. Barricarte, R. Zamora-Ros, M. Arguelles, K. Jirstrom, P. Wallstrom, L. M. Nilsson, I. Ljuslinder, R. C. Travis, K. T. Khaw, N. Wareham, H. Freisling, I. Licaj, M. Jenab, M. J. Gunter, N. Murphy, D. Romaguera-Bosch and E. Riboli, Coffee and tea consumption, genotype based CYP1A2 and NAT2 activity, and colorectal cancer risk - results from the EPIC cohort study, Int. J. Cancer, 2014, 135, 401–412 CrossRef CAS PubMed.
- T. Nakayama and K. Oishi, Influence of coffee (Coffea arabica) and galacto-oligosaccharide consumption on intestinal microbiota and the host responses, FEMS Microbiol. Lett., 2013, 343(2), 161–168 CrossRef CAS PubMed.
- M. Jaquet, I. Rochat, J. Moulin, C. Cavin and R. Bibiloni, Impact of coffee consumption on the gut microbiota: a human volunteer study, Int. J. Food Microbiol., 2009, 130(2), 117–121 CrossRef CAS PubMed.
- C. E. Mills, M. J. Oruna-Concha, D. S. Mottram, G. R. Gibson and J. P. Spencer, The effect of processing on chlorogenic acid content of commercially available coffee, Food Chem., 2013, 141(4), 3335–3340 CrossRef CAS PubMed.
- Y. Okada, K. Oh-oka, Y. Nakamura, K. Ishimaru, S. Matsuoka, K. Okumura, H. Ogawa, M. Hisamoto, T. Okuda and A. Nakao, Dietary resveratrol prevents the development of food allergy in mice, PLoS One, 2012, 7(9), e44338 CAS.
- M. Holmes-McNary and A. S. Baldwin Jr., Chemopreventive properties of trans-resveratrol are associated with inhibition of activation of the IkappaB kinase, Cancer Res., 2000, 60(13), 3477–3483 CAS.
- M. Kimata, M. Shichijo, T. Miura, I. Serizawa, N. Inagaki and H. Nagai, Effects of luteolin, quercetin and baicalein on immunoglobulin E-mediated mediator release from human cultured mast cells, Clin. Exp. Allergy, 2000, 30(4), 501–508 CrossRef CAS PubMed.
- S. A. Van Acker, M. N. Tromp, G. R. Haenen, W. J. van der Vijgh and A. Bast, Flavonoids as scavengers of nitric oxide radical, Biochem. Biophys. Res. Commun., 1995, 214(3), 755–759 CrossRef CAS PubMed.
|
This journal is © The Royal Society of Chemistry 2016 |
Click here to see how this site uses Cookies. View our privacy policy here.