DOI:
10.1039/C5FO00828J
(Paper)
Food Funct., 2016,
7, 294-300
The effects of the aqueous extract and residue of Matcha on the antioxidant status and lipid and glucose levels in mice fed a high-fat diet
Received
8th July 2015
, Accepted 14th September 2015
First published on 25th September 2015
Abstract
Matcha is a kind of powdered green tea produced by grinding with a stone mill. In the present study, the preventive effects of the aqueous extract (water-soluble) and residue (water-insoluble) of Matcha on the antioxidant status and lipid and glucose levels in mice fed a high-fat diet were investigated. Mice were fed seven different experimental diets for 4 weeks: a normal diet control (NC), a high-fat diet (HF), a high-fat diet with 0.025% Matcha (MLD), a high-fat diet with 0.05% Matcha (MMD), a high-fat diet with 0.075% Matcha (MHD), a high-fat diet with 0.05% Matcha aqueous extracts (ME), and a high-fat diet with 0.05% Matcha residues (MR). It was found that serum total cholesterol (TC) and triglyceride (TG) levels of the MHD group were significantly decreased compared to those of the HF group. Furthermore, in the MHD group, the level of high-density lipoprotein-cholesterol (HDL-C) was elevated, on the contrary the level of low-density lipoprotein-cholesterol (LDL-C) was suppressed. Moreover, Matcha could significantly lower the blood glucose levels, and improve the superoxide dismutase (SOD) activity and malondialdehyde (MAD) contents both in serum and liver; besides, the serum GSH-Px activity indicated that the oxidative stress caused by HF could be reversed by administration of Matcha. These findings suggest that Matcha has beneficial effects through the suppression of the blood glucose (BG) accumulation and promotion of the lipid metabolism and antioxidant activities. Moreover, the water-insoluble part of Matcha is suggested to play an important role in the suppression of diet-induced high levels of lipid and glucose.
1. Introduction
Nowadays, the rate of obesity has increased dramatically in the world, and obesity has become a big threat to human health, associated with many diseases such as diabetes,1,2 hypertension,3 hyperlipidemia4 and some types of cancer.5,6 Obesity is caused largely by excessive consumption of dietary fat that results in an imbalance between energy intake and expenditure.7 It is characterized by a series of lipid disturbances8 such as increased TG and TC levels, decreased HDL-C levels, and abnormal LDL-C composition.9,10
Epidemiological surveys have suggested that tea drinking could reduce the risk of diabetes,11,12 cardiovascular diseases13 and cancer.14 Furthermore, studies in cell cultures15–17 and animal models18–20 further supported the potentially beneficial effect of tea on human health. However, these studies all focused on the water-soluble part of tea.
Matcha originated in the Tang Dynasty China and widely spread in Japan during the 14th through 16th centuries. It is a kind of green tea and has distinct manufacturing processes: after removing the veins, stems, and impurities, the leaves of shade-grown tea trees are ground into powder. It's a unique form of tea that is produced by the whole leaf, and the harvested leaves are also briefly steamed to prevent the leaves from being oxidized.21 It means that when drinking Matcha or enjoying foods containing Matcha, you almost consume all the ingredients of tea leaves, including both water-soluble part and water-insoluble part. This is the main difference between Matcha and all other green tea. Nowadays, it has been suggested that Matcha has greater potential health benefits than other green tea. However, to date, information about the functional roles of water-insoluble parts of Matcha has been limited.
Therefore, the objective of the present study is to obtain the aqueous extract (water-soluble) and the residue (water-insoluble) of Matcha, to investigate and compare the effect of Matcha, aqueous extract and residue on body weight, serum/hepatic total cholesterol (TC), triglyceride (TG), malondialdehyde (MDA), superoxide dismutase (SOD) and glutathione peroxidase (GSH-Px) levels in mice fed by a high-fat (HF) diet, for the purpose of establishing a basis for its further application in the food industry.
2. Materials and methods
2.1 Materials
Matcha, a powdered green tea, was kindly supplied by Hangzhou Shandi Tea Industry Co., Ltd (Hangzhou, China). The Matcha extract (ME) was prepared by adding 3 g of Matcha dissolved in 150 mL of boiling distilled water in a boiling water bath for 45 min, then filtered and evaporated with a rotary evaporator and dried. The Matcha residue (MR) was dried in the drying oven for 24 h at a temperature of 70 °C. The compositions of ME and MR are shown in Table 1.
Table 1 Chemical analysis of Matcha, Matcha extract (ME) and Matcha residue (MR)
|
Matcha |
Matcha extract (ME) |
Matcha residue (MR) |
GC, (+)-gallocatechin; EGC, (−)-epigallocatechin; C, (+)-catechin; EC, (−)-epicatechin; EGCG, (−)-epigallocatechin gallate; GCG, (+)-gallocatechin gallate; ECG, (−)-epicatechin gallate; nd, not detected. |
Amino acid (%) |
2.52 |
16.78 |
0.81 |
Soluble saccharide (%) |
5.54 |
17.88 |
1.23 |
Polyphenol (%) |
7.70 |
17.35 |
3.22 |
Soluble protein (%) |
0.02 |
0.08 |
nd |
Fiber (%) |
38.50 |
nd |
85.32 |
Ash (%) |
7.60 |
16.00 |
3.45 |
Phenolic composition (%) |
GC (%) |
0.09 |
0.52 |
0.01 |
EGC (%) |
1.10 |
2.56 |
0.11 |
C (%) |
1.30 |
nd |
nd |
EC (%) |
0.49 |
0.90 |
0.04 |
EGCG (%) |
1.46 |
6.37 |
0.41 |
GCG (%) |
0.03 |
0.16 |
0.01 |
ECG (%) |
0.40 |
0.13 |
nd |
2.2 Animals and diets
Male ICR mice (Grade II, certificate no. SCXK 2009-0039), weighing approximately 15 g, were obtained from the Experimental Animal Center of Zhejiang Chinese Medical University. The mice were maintained in plastic cages at room temperature (22 ± 1 °C) under a 12 h/12 h (light/dark) cycle. The mice were allowed to acclimatize to diets and the experimental facility for 1 week before the experiment. All experiments were performed in compliance with the relevant laws and institutional guidelines, and the Ethical Committee of ZheJiang University approved the experiments.
In the experiment, a total of 70 mice, six weeks of age, were randomly divided into 7 groups of 10 each and were fed the following diets: a normal control diet (NC), a high-fat diet (HF), a high-fat diet with 0.025% Matcha (MLD), a high-fat diet with 0.05% Matcha (MMD), a high-fat diet with 0.075% Matcha (MHD), a high-fat diet with 0.05% Matcha extract (ME), and a high-fat diet with 0.05% Matcha residues (MR). They were allowed free access to water and food.
The high fat diet contained an ordinary mixed feed (88.5%, w/w) with lard (10%, w/w), cholesterol (1%, w/w) and ox bile extract (0.5%, w/w), which were purchased from Silaike Animal Laboratory Co., Ltd (Shanghai, China). To avoid the auto-oxidation of fat components, the high fat diet was stored at −4 °C.
2.3 Biochemical analysis
The experiment lasted for 4 weeks, and the body weights of the mice were recorded every other day. At the end of the experiment, the mice were fasted for 4 h and the blood glucose (BG) levels were measured with a blood glucosimeter. 12 hours after the beginning of the fasting, blood was collected from the eye socket of mice, and then sacrificed by cervical dislocation. Blood samples were centrifuged at 960 rpm for 15 min at 4 °C to separate the serum. Livers of mice were immediately excised from the mice and weighed. The blood serum and livers were stored at −70 °C for biochemical analysis.
The serum concentrations of total cholesterol (TC) and triglyceride (TG) were measured using a commercial kit (Jiancheng Bioengineering Institute, Nanjing, China). The livers were perfused with 0.9% NaCl, and then mashed with a tissue homogenizer in an ice water bath to prepare homogenates, according to the method from Jiancheng Bioengineering Institute, Nanjing, China. The liver homogenates were centrifuged at 2000 rpm for 15 min at 4 °C and the supernatant was collected for TC and TG levels’ measurement with test kits (Jiancheng Bioengineering Institute, Nanjing, China).
The superoxide dismutase (SOD) and glutathione peroxidase (GSH-Px) activities and lipid peroxidation (such as malondialdehyde, MDA) levels in serum and liver samples were measured using commercially available kits (Jiancheng Bioengineering Institute, Nanjing, China) and according to the kits’ manuals.
2.4 Statistical analysis
The results are expressed as means ± SD and evaluated by analysis of variance (ANOVA) followed by post hoc Tukey's test carried out on the SAS system for Windows V9 (SAS Inst., Cary, N.C., U.S.A), and p < 0.05 was regarded as statistically significant.
3. Results
3.1 Effects of Matcha on body weight
To evaluate whether Matcha had an anti-obesity effect, we measured the body weight of mice fed a high-fat diet every other day during the 4-week feeding period (Fig. 1). It was shown that, the body weight of the HF group was significantly higher than that of the NC group during the experiment, which meant a high-fat model was successfully built. An interesting result was found that the body weight of the ME group and the MR group didn't tend to prevent the body weight gain compared with the HF group during different periods of the experiment, while the body weight of the MLD and MMD groups shows a significant difference compared with that of the HF group during the experiment. Moreover, the body weight of the MMD group was evenly similar to that of the NC group.
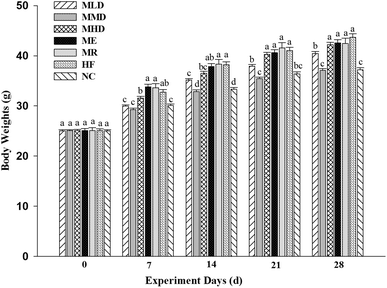 |
| Fig. 1 The body weight of mice fed a high-fat diet every other day during the 4-week feeding period. MLD, mice fed a high-fat diet with 0.025% Matcha; MMD, mice fed a high-fat diet with 0.05% Matcha; MHD, mice fed a high-fat diet with 0.075% Matcha; ME, mice fed a high-fat diet with 0.05% Matcha extract; MR, mice fed a high-fat diet with 0.05% Matcha residues; HF, mice fed a high-fat diet; NC, mice fed a normal control diet. a–d means different letters are significantly different at p < 0.05. | |
3.2 Effects of Matcha on lipid levels
The HF diet induces lipid metabolic indisposition and always results in hyperlipidemia. Firstly, obesity is strongly associated with the development of a fatty liver, distinguished by the accumulation of TC and TG in the liver. The MMD group and the MDH group showed an obvious decline in TC and TG levels as compared to the HF group (Fig. 2a and 3a). Secondly, obesity often shows an increase in the risk of cardiovascular disease and exhibits abnormally higher TC and TG levels in serum. As shown in Fig. 2b and 3b, the administration of Matcha could significantly decrease the serum TC and TG when the mice were challenged with the HF diet. Thirdly, the value of HDL-C and LDL-C more or less reflects the ability of lipid metabolism; therefore the higher HDL-C plus lower LDL-C contribute to keep away from the obesity. The results indicated that the parameters of the MMD group, MHD group and MR group had no significant difference compared with those of the NC group, which had a higher LDL-C level and a lower LDL-C level compared to those of the HF group (Fig. 4a and b). All these data suggested that the administration of Matcha promoted the lipid metabolism induced by HF.
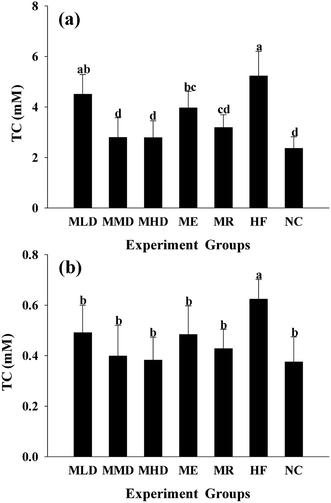 |
| Fig. 2 Effect of Matcha on serum (a) and liver (b) TC levels of mice. MLD, mice fed a high-fat diet with 0.025% Matcha; MMD, mice fed a high-fat diet with 0.05% Matcha; MHD, mice fed a high-fat diet with 0.075% Matcha; ME, mice fed a high-fat diet with 0.05% Matcha extract; MR, mice fed a high-fat diet with 0.05% Matcha residues; HF, mice fed a high-fat diet; NC, mice fed a normal control diet. a–d means different letters are significantly different at p < 0.05. | |
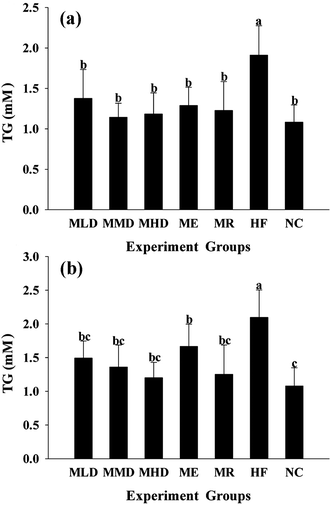 |
| Fig. 3 Effect of Matcha on serum (a) and liver (b) TG levels of mice. MLD, mice fed a high-fat diet with 0.025% Matcha; MMD, mice fed a high-fat diet with 0.05% Matcha; MHD, mice fed a high-fat diet with 0.075% Matcha; ME, mice fed a high-fat diet with 0.05% Matcha extract; MR, mice fed a high-fat diet with 0.05% Matcha residues; HF, mice fed a high-fat diet; NC, mice fed a normal control diet. a–c means different letters are significantly different at p < 0.05. | |
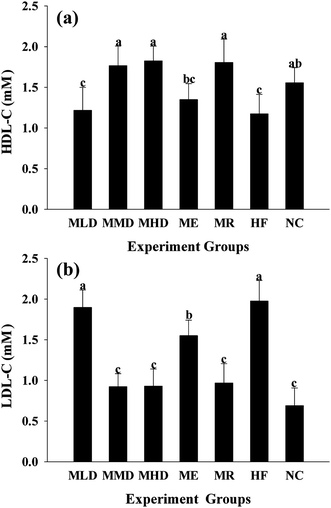 |
| Fig. 4 Effect of Matcha on HDL-C (a) and LDL-C (b) levels of mice. MLD, mice fed a high-fat diet with 0.025% Matcha; MMD, mice fed a high-fat diet with 0.05% Matcha; MHD, mice fed a high-fat diet with 0.075% Matcha; ME, mice fed a high-fat diet with 0.05% Matcha extract; MR, mice fed a high-fat diet with 0.05% Matcha residues; HF, mice fed a high-fat diet; NC, mice fed a normal control diet. a–c means different letters are significantly different at p < 0.05. | |
3.3 Effects of Matcha on blood glucose levels
Diet-induced obesity is a major cause of type 2 diabetes, and the increase in post-fasting blood glucose is normally associated with increased weight. As shown in Fig. 5, blood glucose levels of the HF group were increased by 158% compared to the NC group. Compared to the HF group, the blood glucose levels in the MLD, MMD and MHD groups decreased by 13.3%, 21.2%, and 23.6%, respectively. Surprisingly, the mice fed on a HF diet containing MR significantly reduced the blood glucose levels.
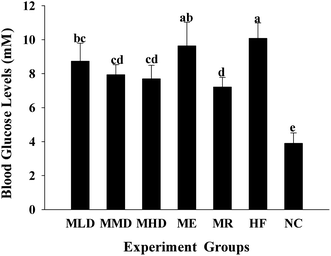 |
| Fig. 5 Effect of Matcha on blood glucose levels of mice. MLD, mice fed a high-fat diet with 0.025% Matcha; MMD, mice fed a high-fat diet with 0.05% Matcha; MHD, mice fed a high-fat diet with 0.075% Matcha; ME, mice fed a high-fat diet with 0.05% Matcha extract; MR, mice fed a high-fat diet with 0.05% Matcha residues; HF, mice fed a high-fat diet; NC, mice fed a normal control diet. a–d means different letters are significantly different at p < 0.05. | |
3.4 Effects of Matcha on antioxidant status
In order to determine the effect of Matcha administration on the antioxidant capacity and the markers of oxidative stress, including the MDA level, SOD and GSH-Px activity both in serum and liver were measured (Fig. 6–8). The results presented that the HF diet increased both serum and liver MDA (p < 0.01 vs. NC group) (Fig. 6), which were significantly reduced by the administration of Matcha in a dose dependent manner. Furthermore, co-fed Matcha prevented the reduction of SOD (Fig. 7) and GSH-Px (Fig. 8) activity both in serum and liver, caused by the HD diet. And meanwhile, the ME and MR groups tended to reverse the high antioxidant status caused by HF diet.
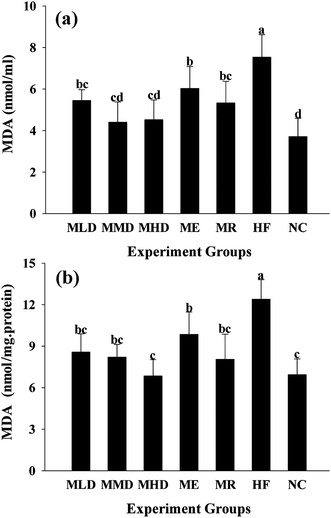 |
| Fig. 6 Effect of Matcha on serum (a) and liver (b) MDA level of mice. MLD, mice fed a high-fat diet with 0.025% Matcha; MMD, mice fed a high-fat diet with 0.05% Matcha; MHD, mice fed a high-fat diet with 0.075% Matcha; ME, mice fed a high-fat diet with 0.05% Matcha extract; MR, mice fed a high-fat diet with 0.05% Matcha residues; HF, mice fed a high-fat diet; NC, mice fed a normal control diet. a–d means different letters are significantly different at p < 0.05. | |
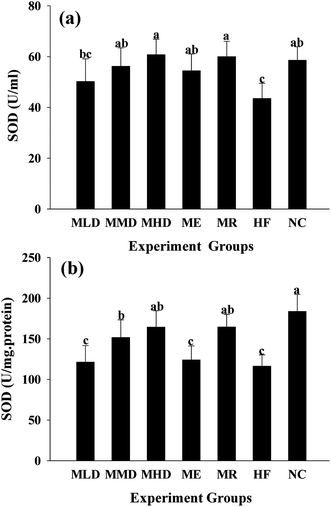 |
| Fig. 7 Effect of Matcha on serum (a) and liver (b) SOD level of mice. MLD, mice fed a high-fat diet with 0.025% Matcha; MMD, mice fed a high-fat diet with 0.05% Matcha; MHD, mice fed a high-fat diet with 0.075% Matcha; ME, mice fed a high-fat diet with 0.05% Matcha extract; MR, mice fed a high-fat diet with 0.05% Matcha residues; HF, mice fed a high-fat diet; NC, mice fed a normal control diet. a–d means different letters are significantly different at p < 0.05. | |
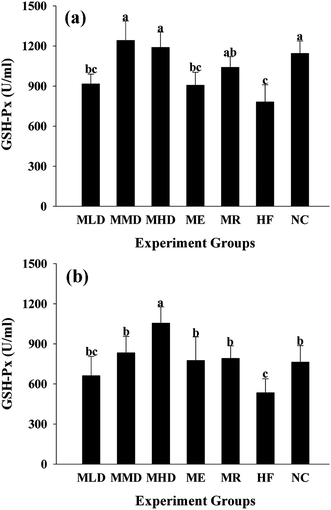 |
| Fig. 8 Effect of Matcha on serum (a) and liver (b) GSH-Px level of mice. MLD, mice fed a high-fat diet with 0.025% Matcha; MMD, mice fed a high-fat diet with 0.05% Matcha; MHD, mice fed a high-fat diet with 0.075% Matcha; ME, mice fed a high-fat diet with 0.05% Matcha extract; MR, mice fed a high-fat diet with 0.05% Matcha residues; HF, mice fed a high-fat diet; NC, mice fed a normal control diet. a–d means different letters are significantly different at p < 0.05. | |
4. Discussion
Nowadays, tea is consumed as a popular beverage worldwide and can be categorized into 4 types according to the degree of fermentation during manufacturing, namely green (unfermented) tea, oolong (semi-fermented) tea, black (fermented) tea and puerh (post-fermented) tea.22 Among them, epidemiological studies suggest that the consumption of green tea contributes to preventing metabolic diseases in humans.23–25 Also, diet-induced obesity,26,27 diabetes28,29 and cardiovascular disease14,30,31 in animal models are reduced by green tea. Green tea contains and is enriched with catechins, known for their antioxidant properties, including epigallocatechin gallate (EGCG), epigallocatechin (EGC) and epicatechin gallate (ECG).32,33 And further research studies have illustrated that catechins possess beneficial effects in the therapy and prevention of various human chronic diseases, such as cancer,34,35 heart disease,36,37 obesity,38,39 and neurodegenerative disease,14,40,41 and promote longevity.34,42
As an exceptional kind of green tea, Matcha's special planting pattern and way of drinking made it to be different from the other green tea. In the present study, the intervention of Matcha on parameters involving hyperglycemia, dyslipidemia, and oxidative stress was investigated.
As shown in Fig. 1, intake of both water-soluble and water-insoluble parts of Matcha was more helpful in controlling weight. Based on the outcomes f lipid and blood glucose levels, the groups that contained Matcha performed better than the HF group, which meant that all the investigated parts of Matcha could lower TC and TG levels of serum and liver, LDL-C levels, and blood glucose levels, and also raise HDL-C levels of mice. Mice fed with 0.075% Matcha (MHD) had a similar performance on lipid and blood glucose levels compared with mice fed with 0.05% Matcha (MMD), while mice fed with 0.025% Matcha had not so good performance which may be due to the low dosage of Matcha. Normally a water-soluble part was considered to be mainly responsible for the positive effects of tea on health, and the residue of tea was abandoned directly. However, in the present work, the water-insoluble part of Matcha (MR group) had the same outstanding effect as MMD and MHD groups on lipid and blood glucose levels. This might be explained by the fact that Matcha residues contained higher fiber compared to the Matcha extract (Table 1). Generally, a high-fiber diet could lower the levels of TC and TG in the blood,43 and also might be effective in controlling body weight, improving blood glucose and lipid levels and increasing the antioxidant status of mice and human beings.44–47 A semblable result was obtained from the effects of Matcha on the antioxidant status.
Normally, tea is steeped in hot water and then the soluble ingredients are consumed. In such a way of drinking, only a small proportion of the healthy nutrients of tea are consumed, and most of the benefits are wasted with the disposed tea. Our study provided further evidence supporting this opinion. The contents of the bioactive chemicals measured in Matcha (Table 1) suggested that most dietary fiber was water-insoluble. And it was observed that the modulation on hyperglycemia, dyslipidemia, and oxidative stress of the Matcha extract was not as effective as the equivalent amount of the entire Matcha. On the contrary, the Matcha residues displayed surprisingly preventive effects. In our opinion, Matcha not only has the health benefits of normal green tea, but also prompted us to take advantage of the water-insoluble nutrients including fibers of tea.
In conclusion, Matcha was found to have beneficial effects via suppression of diet-induced BG accumulation and promotion of the lipid metabolism and antioxidant activities where the water-insoluble part of Matcha was suggested to play an important role. Such results indicated that Matcha could be considered as an alternative to fiber-enriched beverages and is widely used in health foods.
Acknowledgements
This work was supported by the Science and Technology Department of Zhejiang Province, P. R. China (no. 2010LM201-15).
References
- A. H. Mokdad, E. S. Ford, B. A. Bowman, W. H. Dietz, F. Vinicor, V. S. Bales and J. S. Marks, J. Am. Med. Assoc., 2003, 289, 76–79 CrossRef PubMed.
- H. E. Resnick, P. Valsania, J. B. Halter and X. Lin, J. Epidemiol. Community Health, 2000, 54, 596–602 CrossRef CAS.
- J. E. Hall, Hypertension, 2003, 41, 625–633 CrossRef CAS PubMed.
- M. Charlton, Liver Transpl., 2009, 15, S83–S89 CrossRef PubMed.
- S. D. Hursting, N. P. Nunez, L. Varticovski and C. Vinson, Cancer Res., 2007, 67, 2391–2393 CrossRef CAS PubMed.
- H. Møller, A. Mellemgaard, K. Lindvig and J. H. Olsen, Eur. J. Cancer, 1994, 30, 344–350 CrossRef.
- B. M. Spiegelman and J. S. Flier, Cell, 2001, 104, 531–543 CrossRef CAS.
- L. F. Van Gaal, A. Zhang, M. M. Steijaert and I. H. De Leeuw, Int. J. Obes., 1995, 19, S21–S26 CAS.
- R. Franssen, H. Monajemi, E. S. Stroes and J. J. Kastelein, Med. Clin. North Am., 2011, 95, 893–902 CrossRef CAS PubMed.
- T. Repas, S. D. Med., 2011, 64, 241 Search PubMed.
- H. Iso, C. Date, K. Wakai, M. Fukui and A. Tamakoshi, Ann. Intern. Med., 2006, 144, 554–562 CrossRef.
- K. S. Stote and D. J. Baer, J. Nutr., 2008, 138, 1584S–1588S CAS.
- K. Nakachi, S. Matsuyama, S. Miyake, M. Suganuma and K. Imai, Biofactors, 2000, 13, 49–54 CrossRef CAS PubMed.
- N. T. Zaveri, Life Sci., 2006, 78, 2073–2080 CrossRef CAS PubMed.
- M. Z. Fang, Y. Wang, N. Ai, Z. Hou, Y. Sun, H. Lu, W. Welsh and C. S. Yang, Cancer Res., 2003, 63, 7563–7570 CAS.
- M. Sazuka, S. Murakami, M. Isemura, K. Satoh and T. Nukiwa, Cancer Lett., 1995, 98, 27–31 CrossRef CAS.
- F. Yang, H. S. Oz, S. Barve, W. J. de Villiers, C. J. McClain and G. W. Varilek, Mol. Pharmacol., 2001, 60, 528–533 CAS.
- M. Inagake, T. Yamane, Y. Kitao, K. Oya, H. Matsuraoto, N. Kikuoka, H. Nakatani, T. Takahashi, H. Nishimura and A. Iwashiraa, Cancer Sci., 1995, 86, 1106–1111 CrossRef CAS PubMed.
- R. Kuttan, J. Ethnopharmacol., 2002, 83, 109–116 CrossRef.
- T. Yokozawa, T. Nakagawa and K. Kitani, J. Agric. Food Chem., 2002, 50, 3549–3552 CrossRef CAS PubMed.
- N. Yamabe, K. S. Kang, J. M. Hur and T. Yokozawa, J. Med. Food, 2009, 12, 714–721 CrossRef CAS PubMed.
- J. K. Lin and S. Y. Lin-Shiau, Mol. Nutr. Food Res., 2006, 50, 211–217 CAS.
- A. L. Brown, J. Lane, J. Coverly, J. Stocks, S. Jackson, A. Stephen, L. Bluck, A. Coward and H. Hendrickx, Br. J. Nutr., 2009, 101, 886–894 CrossRef CAS PubMed.
- S. Kuriyama, T. Shimazu, K. Ohmori, N. Kikuchi, N. Nakaya, Y. Nishino, Y. Tsubono and I. Tsuji, JAMA, J. Am. Med. Assoc., 2006, 296, 1255–1265 CrossRef CAS PubMed.
- Y. C. Yang, F. H. Lu, J. S. Wu, C. H. Wu and C. J. Chang, Arch. Intern. Med., 2004, 164, 1534 CrossRef PubMed.
- M. Bose, J. D. Lambert, J. Ju, K. R. Reuhl, S. A. Shapses and C. S. Yang, J. Nutr., 2008, 138, 1677–1683 CAS.
- M. S. Lee, C. T. Kim and Y. Kim, Ann. Nutr. Metab., 2009, 54, 151–157 CrossRef CAS PubMed.
- H. Tsuneki, M. Ishizuka, M. Terasawa, J. B. Wu, T. Sasaoka and I. Kimura, BMC Pharmacol., 2004, 4, 18 CrossRef PubMed.
- L. Y. Wu, C. C. Juan, L. T. Ho, Y. P. Hsu and L. S. Hwang, J. Agric. Food Chem., 2004, 52, 643–648 CrossRef CAS PubMed.
- L. B. Tijburg, S. A. Wiseman, G. W. Meijer and J. A. Weststrate, Atherosclerosis, 1997, 135, 37–47 CrossRef.
- W. Young, R. L. Hotovec and A. G. Romero, Nature, 1967, 216, 1015–1016 CAS.
- J. V. Higdon and B. Frei, Crit. Rev. Food Sci. Nutr., 2003, 43, 89–143 CrossRef CAS PubMed.
-
T. L. Lunder
, Catechins of green tea: antioxidant activity, ACS symposium series., 1992, 507, 114–120.
- N. Sueoka, M. Suganuma, E. Sueoka, S. Okabe, S. Matsuyama, K. Imai, K. Nakachi and H. Fujiki, Ann. N. Y. Acad. Sci., 2001, 928, 274–280 CrossRef CAS PubMed.
- M. Zhang, C. A. J. Holman, J. P. Huang and X. Xie, Carcinogenesis, 2007, 28, 1074–1078 CrossRef CAS PubMed.
- H. Mukhtar and N. Ahmad, Am. J. Clin. Nutr., 2000, 71, 1698s–1702s CAS.
- J. A. Vinson, Biofactors, 2000, 13, 127–132 CrossRef CAS PubMed.
- M. Boschmann and F. Thielecke, J. Am. Coll. Nutr., 2007, 26, 389S–395S CrossRef CAS PubMed.
- K. C. Maki, M. S. Reeves, M. Farmer, K. Yasunaga, N. Matsuo, Y. Katsuragi, M. Komikado, I. Tokimitsu, D. Wilder and F. Jones, J. Nutr., 2009, 139, 264–270 CrossRef CAS PubMed.
- S. Mandel, Y. Avramovich-Tirosh, L. Reznichenko, H. Zheng, O. Weinreb, T. Amit and M. Youdim, Neurosignals, 2005, 14, 46–60 CrossRef CAS PubMed.
- O. Weinreb, S. Mandel, T. Amit and M. B. Youdim, J. Nutr. Biochem., 2004, 15, 506–516 CrossRef CAS PubMed.
- R. J. Ruch, S. J. Cheng and J. E. Klaunig, Carcinogenesis, 1989, 10, 1003–1008 CrossRef CAS PubMed.
- P. H. Knopp, H. R. Superko, M. Davidson, W. Insull, C. A. Dujovne, P. O. Kwiterovich, J. H. Zavoral, K. Graham, R. R. O'Connor and D. A. Edelman, Am. J. Prev. Med., 1999, 17, 18–23 CrossRef.
- J. Wang, Y. P. Cao, C. T. Wang and B. G. Sun, Carbohydr. Polym., 2011, 86, 1192–1197 CrossRef CAS PubMed.
- C. Wang, D. H. Catlin, B. Starcevic, D. Heber, C. Ambler, N. Berman, G. Lucas, A. Leung, K. Schramm, P. W. Lee, L. Hull and R. S. Swerdloff, J. Clin. Endocrinol. Metab., 2005, 90, 3550–3559 CrossRef CAS PubMed.
- C. A. Thomson, C. L. Rock, A. R. Giuliano, T. R. Newton, H. Cui, P. M. Reid, T. L. Green and D. S. Alberts, Eur. J. Nutr., 2005, 44, 18–25 CrossRef PubMed.
- K. Berger, P. Falck, C. Linninge, U. Nilsson, U. Axling, C. Grey, H. Stalbrand, E. Norderg Karlsson, M. Nyman, C. Holm and P. Adlercreutz, J. Agric. Food Chem., 2014, 62, 8169–8178 CrossRef CAS PubMed.
|
This journal is © The Royal Society of Chemistry 2016 |