DOI:
10.1039/C5DT04429D
(Frontier)
Dalton Trans., 2016,
45, 1299-1305
NHC-based pincer ligands: carbenes with a bite†
Received
10th November 2015
, Accepted 8th December 2015
First published on 17th December 2015
Abstract
In this frontier article we overview the emergence and scope of NHC-based CCC and CNC pincer systems, i.e. complexes containing mer-tridentate ligands bearing two NHC donor groups, comment on their effectiveness in applications, and highlight areas for future development and exploitation.
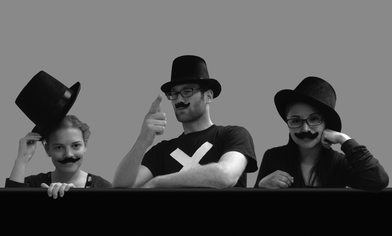 Rhiann, Adrian and Lucero (left – right) in customary Chaplin group poses | Rhiann graduated from the University of Liverpool in 2012 with a MChem with Research in industry, working at GlaxoSmithKline and in the research group of Prof. J. Xiao. Following award of a Chancellor's Scholarship in 2012, she has been a PhD student in the Chaplin group working on NHC-based macrocyclic pincers with a view to applications in supramolecular chemistry. |
| Lucero obtained BSc and MSc degrees from the National Autonomous University of Mexico (UNAM), remaining to work under the supervision of Prof. J. Garcia for her PhD (2013). Following postdoctoral work in the group of Prof. A. Paz at the Centre for Research and Advanced Studies of the National Polytechnic Institute (CINVESTAV) and award of a CONACYT postdoctoral stays abroad fellowship, she joined the Chaplin group in 2014 and has been working on the synthesis of imidazolinylidene-based pincers. |
| Born in New Zealand, Adrian obtained his undergraduate degree from Massey University (2003) before relocating to Switzerland, where he carried out his doctoral studies at the Ecole Polytechnique Fédérale de Lausanne (EPFL), graduating in 2007. He then spent four years as a postdoctoral researcher at the University of Oxford in the group of Prof. A. Weller, holding the R. J. P. Williams Junior Research Fellowship in Chemistry at Wadham College during the latter two years. Following award of a Royal Society University Research Fellowship, Adrian commenced his independent academic career at the University of Warwick in October 2011. Work in the Chaplin group involves synthetic organometallic chemistry of the late transition metals, focusing on the application of supramolecular inspired ligands (http://go.warwick.ac.uk/chaplingroup, @chaplinlab ). For his contributions to organometallic chemistry, he was awarded a 2015 RSC Harrison-Meldola Memorial Prize. |
Introduction
Forming adducts with elements throughout the periodic table, the applications of N-heterocyclic carbenes (NHCs) pervade modern synthetic chemistry.1,2 Rivalling more established phosphine ligands, these carbon-based donors have become ubiquitous in organometallic chemistry and, building on pioneering work by Herrmann in the late 90s, transformed homogeneous transition metal catalysis.1–4 The evolution of ruthenium catalysts for olefin metathesis is the quintessential example (e.g. Grubbs II pre-catalysts), but the emergence of many other keystone organic transformations, such as palladium catalysed cross coupling reactions (e.g. PEPPSI pre-catalysts), ‘click chemistry’, and gold catalysed reactions of alkynes, are also associated with the ever increasing application of NHCs.3,4 Most commonly employed imidazolylidene and imidazolinylidene NHCs are stronger sigma donors (and weaker pi-acceptors) in comparison to alkyl phosphines, although enhancing their appeal as ancillary ligands a wide range of donor properties can be accessed within the broader ligand class.5,6 The steric characteristics of NHC ligands further distinguish them from their phosphine counterparts; the combination of shorter metal–ligand bonds and flanking substituents that are directed towards the bound metal, permit NHC ligands to encroach deep into the metal coordination sphere.5 Underpinning these hallmarks, the ability to tune the electronic and steric environment of the metal coordination sphere using NHC ligands is facilitated by a wide range of straightforward synthetic protocols for the respective pro-ligands [NHC·H]+.7
Alongside the meteoric rise in the use of NHC ligands, mer-tridentate “pincer” ligand architectures pervade contemporary homogeneous catalysis, conferring high thermal stability and supporting a broad range of metal-based reactivity.8 Phosphine-based pincers with central pyridine or aryl donors in particular have been widely investigated, enabling excellent catalytic performance under forcing reaction conditions, such as those associated with alkane dehydrogenation,9 and fine reaction control, for example, through cooperative metal–ligand reactivity mediated by pyridine dearomatisation.10 Although significantly less developed, NHC-based CCC and CNC variants – combining the favorable characteristics of NHC donors with mer-tridentate ligand architectures – therefore represent a potentially powerful amalgamation of modern ligand design concepts. Supplementing earlier commentaries,11 in this frontier article we overview the literature to gauge the emergence of NHC-based pincer systems, comment on their effectiveness in applications, and highlight areas for future development and exploitation.
Discussion
Current ligand scope and synthetic procedures
Imidazolylidene-based systems of the type A (C–E–C) and B (C^E^C) were the first examples of NHC-based pincers and remain heavily investigated.12 Indeed, from a survey of the literature, these systems account for 84% of publications and 78% of solid-state structures associated with this bourgeoning ligand class (as of 09/2015; Fig. 1).13 As a consequence of the change in backbone composition, A and B display significantly different ligand characteristics: the presence of bridging methylene groups in the pincer backbone of B leads to characteristically twisted C2 conformations, which project the NHC wingtips in opposite directions out of the coordination plane, and results in the adoption of more ideal metal coordination geometries in comparison to A (C–M–C = 172(2)° vs. 155(6)°).14 This simple structural adaption of the ligand therefore results in systems with complementary steric profiles, and has significant implications for the nature of the constituent metal-NHC bonding.15 Often dynamic in solution,16,17 atropisomers of B can be resolved through incorporation of chiral substituents (i.e.D), and such species could be of future interest for applications in asymmetric catalysis.18 Subsequent variations of A and B have included modification of the central donor group to produce ligands with different electronic profiles (E–H). Curiously, pincers that incorporate significantly different backbone geometries (C, I–K, S) or NHC donors (L–T) have received little attention, suggesting the full capacity of the wider ligand class is yet to be exploited. Amongst the reported CCC and CNC systems, the coordination chemistry of late transition metals, and particularly palladium and ruthenium, has been most frequently explored (Fig. 1). Helping to set them apart from their phosphine-based analogues, a significant body of work has also emerged based on NHC-based pincer complexes of both the early transition metals and f-block elements, and their applications (16 articles, 44 crystal structures, vide infra).13
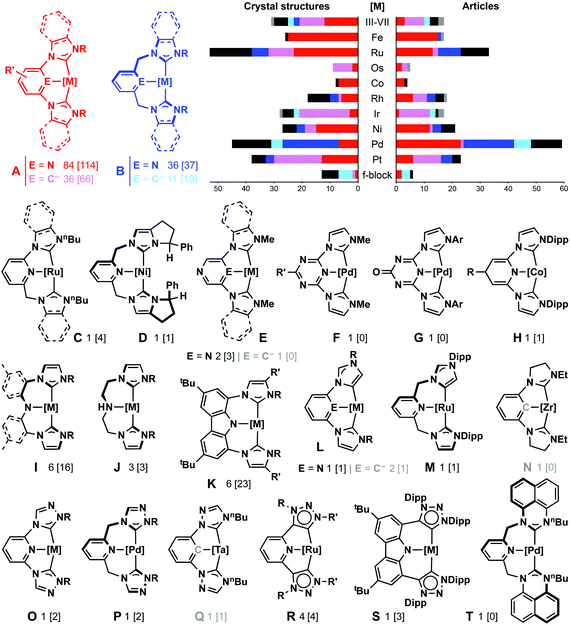 |
| Fig. 1 Reported NHC-based pincer systems: article count from Scifinder™ with deposits in CSD given in square brackets (up until 09/2015 and only for ligands shown to adopt mer-configurations).13 | |
Although preparation of the necessary pro-ligands is generally straightforward, the subsequent complexation is not always, providing a potential barrier to wider investigation. Scheme 1 gives representative methods that have been employed.17,19–26 Direct coordination of the singlet carbene species, generated by pro-ligand deprotonation with a strong base, is conceptually the simplest means, although the high reactivity of these species often precludes their isolation. Indeed, well-defined free-carbenes of this type are limited to a handful of examples, primarily based on 2,6-functionalised pyridine and benzene backbones.19,27–30 Avoiding issues associated with these highly reactive intermediates, ‘accessible syntheses’31 involving equilibrium reactions with weak (e.g. Et3N, Cs2CO3) or coordinated bases ([M]OAc, [M]NR2) can be used and account for approximately half of the reported pincer systems. Transmetallation is another common method; with silver transfer agents, generated by reaction of the respective pro-ligands with Ag2O, the most popular. Coordination of anionic CCC-pincer ligands typically proceeds with concomitant C–H or C–Br bond cyclometalation of the backbone, although in situ preparation of anionic free-carbenes or use of zirconium transfer agents, generated by reaction with Zr(NMe2)4, have also proved to be valuable alternatives. Formation of multi-nuclear20,32–35 or abnormal carbene complexes (i.e.M, N)23,36–38 both represent potentially detrimental outcomes of the aforementioned methodology: metal precursors bearing non-chelating ligands and use of benzimidazolium-based pro-ligands, respectively, are possible means to avoid such outcomes.
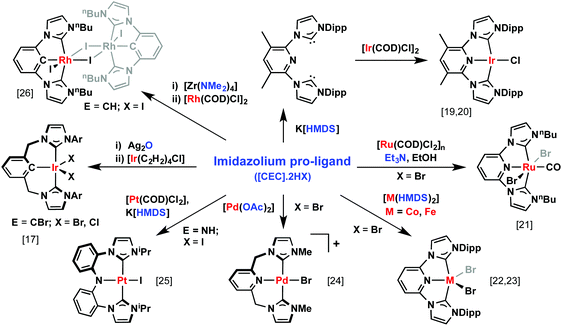 |
| Scheme 1 Synthesis of NHC-based pincer complexes. | |
Applications
Fuelled by the successful application of NHC ligands in palladium-catalysed transformations, the emergence of NHC-based pincer systems was closely associated with applications in C–C coupling reactions.4,12 A variety of well-defined CCC- and CNC-Pd(II) pre-catalysts have been reported to promote the Suzuki–Miyaura and Heck–Mizoroki reactions with high efficiency using low metal loadings, however, increasingly reactions are being performed with pincer systems generated in situ from pro-ligand and [Pd(OAc)2].39 Targeted for increased thermal stability under the forcing reactions conditions typically associated with such reactions, the exact role of the pincer remains unclear. For instance, although pre-catalysts 1 (Fig. 2) show high activity in Heck–Mizoroki reaction, as do mono-dentate NHC systems,2 they were noted to thermally degrade via reductive methyl-NHC coupling.40 A product of methyl group migration from palladium(II) to a coordinated NHC donor group of a CNC pincer has been crystallographically characterised and helps corroborate such reactivity.41 A significant recent advance in this area is the development of active Ni(II) CNC pre-catalysts.42
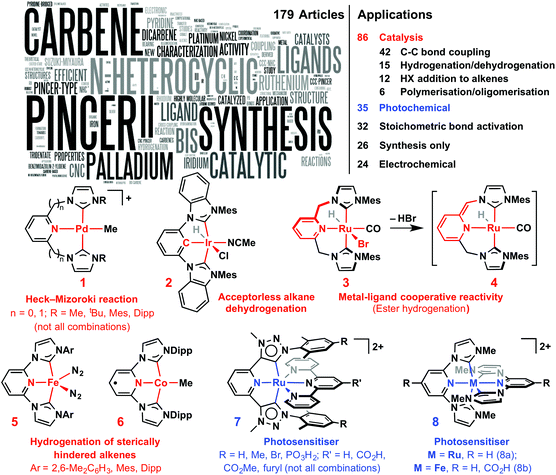 |
| Fig. 2 Applications of NHC-based pincer systems (text cloud generated from article titles).43 | |
Well defined platinum-metal-based CNC and CCC pincers have been used to promote a variety of other transformations, including hydrogenation (Ru, Rh),14,20,30,33,44,45 hydrosilylation (Rh, Pd),33,35 hydroamination (Rh, Ir, Pd, Pt),28,46 hydrovinylation (Pt),47 acceptorless alkane dehydrogenation (Ir, e.g.2),48,49 and aryl C–H bond borylation (Ir).49 As an interesting comparison to robust and highly active iridium phosphine-based PCP catalysis,9 CCC-Ir analogues reported to date are notable for low activity in transfer dehydrogenation reactions performed at high temperature – typically achieving <20 catalytic turnovers (cf. >1000).34,37,49,50 Interestingly though, 2 displays comparable activity to PCP analogues for the acceptorless dehydrogenation of cyclooctane, displaying a high initial activity (TOF 12 h−1 at 150 °C) and no product inhibition. These desirable characteristics could be a result of steric effects associated with the metal orientated N-aryl substituents, although ultimately the usefulness of 2 is limited by catalyst decomposition that prevents catalytic turnover greater than ca. 100 TON.48 A particularly noteworthy development is the demonstration of metal–ligand cooperative reactivity within C^N^C ligand scaffolds.36,44,51 For example, unlike a structurally analogous pyridine-based Ru-PNP pincer, 3 is an active pre-catalyst for the selective hydrogenation of esters.36 Complex 4 could be characterised in situ and re-aromatises on reaction with hydrogen, carbon dioxide and nitriles.36,51 Isolation of a structurally similar rhodium complex was recently demonstrated in our laboratories.52
The propensity for NHC ligands to form a wide range of metal adducts allows for unique opportunities for exploitation in catalysis and differentiates these pincer ligands from other donor-based variants. Indeed, complexes of first row and early transition metals have been showing promising activity in a range of organic transformations. For instance, Fe(0) and Co(II) complexes 5 and 6 bearing bulky C–N–C ligands are highly active catalysts for the hydrogenation of sterically hindered alkenes, such as trans-methylstilbene, 1-methyl-1-cyclohexene and 2,3-dimethyl-2-butene; substrates that typically can not be hydrogenated using platinum group metal catalysts.53 Other notable examples include, C–C bond coupling reactions (Ni),42 hydroamination (Ti, Zr, Hf),54 ethylene dimerisation (Cr) and polymerization (Ti, V).55 Investigation of f-block adducts also raises intriguing future prospects for the application of NHC-based pincer ligands.29,56
Other than applications in catalysis, NHC-pincer complexes, and in particular Ru(II) adducts, are increasingly being recognised for their useful photophysical properties. Ruthenium(II) complexes 7, for example, are notable for microsecond 3MLCT excited-state lifetimes, four orders of magnitude higher than [Ru(terpyridine)2]2+ and, lending themselves towards practical implantation, the capacity for robust immobilisation on TiO2 (i.e. R = PO3H2; R′ = CO2H).57 Interestingly, while both homoleptic C^N^C and terpyridine Ru(II) complexes are practically non luminescent at room temperature,58 ruthenium(II) bis(C–N–C) complex 8a displays long lifetime photoluminescence in solution.59 Significantly the first row congener 8b displays an exceptionally long 3MLCT lifetime for Fe(II) (9 ps; R = H) – two orders longer than the related bis(terpyridine) complex – raising the exciting possibility for use of earth-abundant-metal-based photosensitisers.60 Outside complexes of the group 8 transition metals, Pt(II) C–C–C complexes have been reported that exhibit blue light emission under ambient conditions, while analogous C–N–C complexes show aqua- and vapo-chromic behaviour.61 Moreover, Ir(III) bis(C–C–C) complexes that emit in the near-UV have also been described recently.62
Outlook
The incorporation of NHC donors into pincer ligand topologies is a means for synergistically combining two of the most successful developments in contemporary ligand design. As a ligand class, NHC-based pincer ligands have vast scope for variation/tuning of donor characteristics and steric profiles. However, this potential is yet to be fully realised with the overwhelming majority of systems reported to date based on imidazolylidene donor groups. Systems bearing imidazolinylidene, pyrrolidinylidene, abnormal imidazolylidene and triazolylidene donor groups, widely employed as mono-dentate ligands in their own right, proffer further capacity for fruitful variation of the pincer donor properties. As a means to systematically quantify such variation, we suggest measurement of carbonyl stretching frequencies in [Rh(CEC)(CO)]+ (i.e. in CH2Cl2 solution) or redox potentials of [Ru(CEC)2]2+ (E = N, C−). Conveniently the respective pro-ligands should be readily accessed from established protocols from the respective mono-dentate analogues,7 however, while a number of synthetic procedures are becoming established for the subsequent coordination to metal centers (free carbene, weak/coordinated bases, silver transmetallation reagents), further consolidation and evolution of this methodology is required. For instance, increased use of zirconium transmetallation reagents or implementation of decarboxylation reactions of CEC·2(CO2) (E = N, CH, CBr).2 Other potentially useful ligand variations could include incorporation of boryl or silyl donors in the pincer backbone.63
Increased structural variation would help support growing uses of NHC-based pincers as ancillary ligands in homogeneous catalysis. The ability of NHC donors to form adducts with metals outside of the platinum group is in particular an area for future exploration, especially using earth abundant metals such as Fe, Co and Ni. Under forcing reactions conditions, such as palladium catalysed C–C coupling reactions and alkane dehydrogenation, NHC-based pincer complexes show promising potential but also suffer from non-negligible decomposition; representing an important consideration in future ligand design. To this end some of us been have developing macrocyclic C–N–C and C^N^C ligands, which may enable not only more robust pincer ligand binding, but additional scope for reaction control.16,52,64 With promising advances being made exploiting the photophysical properties of both CCC and CNC pincers, the ability to tune the absorption and emission characteristics of bound metals, through variation of the ligand composition, also makes for exciting future applications of NHC-based pincers in materials science.
Acknowledgements
We gratefully acknowledge financial support from the University of Warwick (R. E. A.), CONACYT (L. G. S) and the Royal Society (A. B. C.).
Notes and references
-
(a) M. N. Hopkinson, C. Richter, M. Schedler and F. Glorius, Nature, 2014, 510, 485–496 CrossRef CAS PubMed
;
(b) D. Martin, M. Melaimi, M. Soleilhavoup and G. Bertrand, Organometallics, 2011, 30, 5304–5313 CrossRef CAS PubMed
.
- F. E. Hahn and M. C. Jahnke, Angew. Chem., Int. Ed., 2008, 47, 3122–3172 CrossRef CAS PubMed
.
-
(a) J. D. Egbert, C. S. J. Cazin and S. P. Nolan, Catal. Sci. Technol., 2013, 3, 912–926 RSC
;
(b) S. P. Nolan, Acc. Chem. Res., 2011, 44, 91–100 CrossRef CAS PubMed
;
(c) S. Díez-González, N. Marion and S. P. Nolan, Chem. Rev., 2009, 109, 3612–3676 CrossRef PubMed
;
(d) C. Samojłowicz, M. Bieniek and K. Grela, Chem. Rev., 2009, 109, 3708–3742 CrossRef PubMed
.
-
(a) G. C. Fortman and S. P. Nolan, Chem. Soc. Rev., 2011, 40, 5151–5169 RSC
;
(b) E. A. B. Kantchev, C. J. O'Brien and M. G. Organ, Angew. Chem., Int. Ed., 2007, 46, 2768–2813 CrossRef CAS PubMed
.
- T. Dröge and F. Glorius, Angew. Chem., Int. Ed., 2010, 49, 6940–6952 CrossRef PubMed
.
- R. Tonner, G. Heydenrych and G. Frenking, Chem. – Asian J., 2007, 2, 1555–1567 CrossRef CAS PubMed
.
- L. Benhamou, E. Chardon, G. Lavigne, S. Bellemin-Laponnaz and V. Cesar, Chem. Rev., 2011, 111, 2705–2733 CrossRef CAS PubMed
.
-
(a)
G. van Koten and D. Milstein, Topics in Organometallic Chemistry Vol. 40 – Organometallic Pincer Chemistry, Springer, 2013 Search PubMed
;
(b) M. Albrecht, Dalton Trans., 2011, 40, 8733–8744 RSC
;
(c) M. E. van der Boom and D. Milstein, Chem. Rev., 2003, 103, 1759–1792 CrossRef CAS PubMed
;
(d) M. Albrecht and G. van Koten, Angew. Chem., Int. Ed., 2001, 40, 3750–3781 CrossRef CAS
.
-
(a) W. Yao, Y. Zhang, X. Jia and Z. Huang, Angew. Chem., Int. Ed., 2014, 53, 1390–1394 CrossRef CAS PubMed
;
(b) J. Choi, A. H. R. MacArthur, M. Brookhart and A. S. Goldman, Chem. Rev., 2011, 111, 1761–1779 CrossRef CAS PubMed
.
-
(a) T. Zell and D. Milstein, Acc. Chem. Res., 2015, 48, 1979–1994 CrossRef CAS PubMed
;
(b) C. Gunanathan and D. Milstein, Chem. Rev., 2014, 114, 12024–12087 CrossRef CAS PubMed
;
(c) J. I. van der Vlugt and J. N. H. Reek, Angew. Chem., Int. Ed., 2009, 48, 8832–8846 CrossRef CAS PubMed
.
-
(a) M. Poyatos, J. A. Mata and E. Peris, Chem. Rev., 2009, 109, 3677–3707 CrossRef CAS PubMed
;
(b) D. Pugh and A. A. Danopoulos, Coord. Chem. Rev., 2007, 251, 610–641 CrossRef CAS
;
(c) E. Peris and R. H. Crabtree, Coord. Chem. Rev., 2004, 248, 2239–2246 CrossRef CAS
.
-
(a) E. Peris, J. Mata, J. A. Loch and R. H. Crabtree, Chem. Commun., 2001, 201–202 RSC
;
(b) A. A. D. Tulloch, A. A. Danopoulos, G. J. Tizzard, S. J. Coles, M. B. Hursthouse, R. S. Hay-Motherwell and W. B. Motherwell, Chem. Commun., 2001, 1270–1271 RSC
.
- For brevity references for A–T are provided in the ESI.† Cumulative article counts given per unique metal grouping-pincer ligand type combination (plot) and pincer ligand type (diagrams).
- Excludes one crystallographically characterised example of type B system bearing a fac-coordinated pincer ligand: M. Hernández-Juárez, M. Vaquero, E. Álvarez, V. Salazar and A. Suárez, Dalton Trans., 2013, 42, 351–354 RSC
.
- J.-N. Luy, S. A. Hauser, A. B. Chaplin and R. Tonner, Organometallics, 2015, 34, 5099–5112 CrossRef CAS
.
-
(a) R. E. Andrew, D. W. Ferdani, C. A. Ohlin and A. B. Chaplin, Organometallics, 2015, 34, 913–917 CrossRef CAS
;
(b) S. Saito, I. Azumaya, N. Watarai, H. Kawasaki and R. Yamasaki, Heterocycles, 2009, 79, 531 CrossRef
;
(c) J. R. Miecznikowski, S. Gründemann, M. Albrecht, C. Mégret, E. Clot, J. W. Faller, O. Eisenstein and R. H. Crabtree, Dalton Trans., 2003, 831–838 RSC
.
- K. M. Schultz, K. I. Goldberg, D. G. Gusev and D. M. Heinekey, Organometallics, 2011, 30, 1429–1437 CrossRef CAS
.
- K. Yoshida, S. Horiuchi, T. Takeichi, H. Shida, T. Imamoto and A. Yanagisawa, Org. Lett., 2010, 12, 1764–1767 CrossRef CAS PubMed
.
- D. Pugh, A. Boyle and A. A. Danopoulos, Dalton Trans., 2008, 1087–1094 RSC
.
- A. A. Danopoulos, D. Pugh and J. A. Wright, Angew. Chem., Int. Ed., 2008, 47, 9765–9767 CrossRef PubMed
.
- M. Poyatos, J. A. Mata, E. Falomir, R. H. Crabtree and E. Peris, Organometallics, 2003, 22, 1110–1114 CrossRef CAS
.
- A. A. Danopoulos, J. A. Wright, W. B. Motherwell and S. Ellwood, Organometallics, 2004, 23, 4807–4810 CrossRef CAS
.
- A. A. Danopoulos, N. Tsoureas, J. A. Wright and M. E. Light, Organometallics, 2004, 23, 166–168 CrossRef CAS
.
- S. Gründemann, M. Albrecht, J. A. Loch, J. W. Faller and R. H. Crabtree, Organometallics, 2001, 20, 5485–5488 CrossRef
.
- W. B. Cross, C. G. Daly, R. L. Ackerman, I. R. George and K. Singh, Dalton Trans., 2011, 40, 495–505 RSC
.
- R. J. Rubio, G. T. S. Andavan, E. B. Bauer, T. K. Hollis, J. Cho, F. S. Tham and B. Donnadieu, J. Organomet. Chem., 2005, 690, 5353–5364 CrossRef CAS
.
-
(a) E. M. Matson, G. Espinosa Martinez, A. D. Ibrahim, B. J. Jackson, J. A. Bertke and A. R. Fout, Organometallics, 2015, 34, 399–407 CrossRef CAS
;
(b) D. S. McGuinness, J. A. Suttil, M. G. Gardiner and N. W. Davies, Organometallics, 2008, 27, 4238–4247 CrossRef CAS
;
(c) J. A. Wright, A. A. Danopoulos, W. B. Motherwell, R. J. Carroll, S. Ellwood and J. Saßmannshausen, Chem. Ber., 2006, 2006, 4857–4865 Search PubMed
;
(d) D. S. McGuinness, V. C. Gibson and J. W. Steed, Organometallics, 2004, 23, 6288–6292 CrossRef CAS
.
- J. Houghton, G. Dyson, R. E. Douthwaite, A. C. Whitwood and B. M. Kariuki, Dalton Trans., 2007, 3065–3073 RSC
.
- D. Pugh, J. A. Wright, S. Freeman and A. A. Danopoulos, Dalton Trans., 2006, 775–782 RSC
.
- A. A. Danopoulos, S. Winston and W. B. Motherwell, Chem. Commun., 2002, 1376–1377 RSC
.
- D. J. Nelson, Eur. J. Inorg. Chem., 2015, 2015, 2012–2027 CrossRef CAS
.
- R. S. Simons, P. Custer, C. A. Tessier and W. J. Youngs, Organometallics, 2003, 22, 1979–1982 CrossRef CAS
.
- M. Poyatos, E. Mas-Marzá, J. A. Mata, M. Sanau and E. Peris, Eur. J. Inorg. Chem., 2003, 2003, 1215–1221 CrossRef
.
- M. Raynal, R. Pattacini, C. S. J. Cazin, C. Vallée, H. Olivier-Bourbigou and P. Braunstein, Organometallics, 2009, 28, 4028–4047 CrossRef CAS
.
- G. T. S. Andavan, E. B. Bauer, C. S. Letko, T. K. Hollis and F. S. Tham, J. Organomet. Chem., 2005, 690, 5938–5947 CrossRef CAS
.
- G. A. Filonenko, E. Cosimi, L. Lefort, M. P. Conley, C. Copéret, M. Lutz, E. J. M. Hensen and E. A. Pidko, ACS Catal., 2014, 4, 2667–2671 CrossRef CAS
.
- W. Zuo and P. Braunstein, Organometallics, 2012, 31, 2606–2615 CrossRef CAS
.
- W. Zuo and P. Braunstein, Dalton Trans., 2012, 41, 636–643 RSC
.
- For recent examples see:
(a) C. Gao, H. Zhou, S. Wei, Y. Zhao, J. You and G. Gao, Chem. Commun., 2013, 49, 1127–1129 RSC
;
(b) T. Tu, J. Malineni, X. Bao and K. H. Dötz, Adv. Synth. Catal., 2009, 351, 1029–1034 CrossRef CAS
.
- D. J. Nielsen, K. J. Cavell, B. W. Skelton and A. H. White, Inorg. Chim. Acta, 2006, 359, 1855–1869 CrossRef CAS
.
- A. A. Danopoulos, N. Tsoureas, J. C. Green and M. B. Hursthouse, Chem. Commun., 2003, 756–757 RSC
.
-
(a) M. Xu, X. Li, Z. Sun and T. Tu, Chem. Commun., 2013, 49, 11539–11541 RSC
;
(b) T. Tu, H. Mao, C. Herbert, M. Xu and K. H. Dötz, Chem. Commun., 2010, 46, 7796–7793 RSC
;
(c) K. Inamoto, J.-I. Kuroda, E. Kwon, K. Hiroya and T. Doi, J. Organomet. Chem., 2009, 694, 389–396 CrossRef CAS
;
(d) K. Inamoto, J.-I. Kuroda, K. Hiroya, Y. Noda, M. Watanabe and T. Sakamoto, Organometallics, 2006, 25, 3095–3098 CrossRef CAS
.
- Text cloud generated using article titles using Wordle™ (http://www.wordle.net), with the words ‘complex’ and ‘complexes’ removed.
- M. Hernández-Juárez, J. López-Serrano, P. Lara, J. P. Morales-Cerón, M. Vaquero, E. Álvarez, V. Salazar and A. Suárez, Chem. – Eur. J, 2015, 21, 7540–7555 CrossRef PubMed
.
- G. A. Filonenko, M. J. B. Aguila, E. N. Schulpen, R. van Putten, J. Wiecko, C. Müller, L. Lefort, E. J. M. Hensen and E. A. Pidko, J. Am. Chem. Soc., 2015, 137, 7620–7623 CrossRef CAS PubMed
.
-
(a) P. Cao, J. Cabrera, R. Padilla, D. Serra, F. Rominger and M. Limbach, Organometallics, 2012, 31, 921–929 CrossRef CAS
;
(b) E. B. Bauer, G. T. S. Andavan, T. K. Hollis, R. J. Rubio, J. Cho, G. R. Kuchenbeiser, T. R. Helgert, C. S. Letko and F. S. Tham, Org. Lett., 2008, 10, 1175–1178 CrossRef CAS PubMed
.
- D. Serra, P. Cao, J. Cabrera, R. Padilla, F. Rominger and M. Limbach, Organometallics, 2011, 30, 1885–1895 CrossRef CAS
.
- A. R. Chianese, M. J. Drance, K. H. Jensen, S. P. McCollom, N. Yusufova, S. E. Shaner, D. Y. Shopov and J. A. Tendler, Organometallics, 2014, 33, 457–464 CrossRef CAS
.
- A. R. Chianese, A. Mo, N. L. Lampland, R. L. Swartz and P. T. Bremer, Organometallics, 2010, 29, 3019–3026 CrossRef CAS
.
- A. R. Chianese, S. E. Shaner, J. A. Tendler, D. M. Pudalov, D. Y. Shopov, D. Kim, S. L. Rogers and A. Mo, Organometallics, 2012, 31, 7359–7367 CrossRef CAS
.
- G. A. Filonenko, D. Smykowski, B. M. Szyja, G. Li, J. Szczygieł, E. J. M. Hensen and E. A. Pidko, ACS Catal., 2015, 5, 1145–1154 CrossRef CAS
.
- R. E. Andrew and A. B. Chaplin, Inorg. Chem., 2015, 54, 312–322 CrossRef CAS PubMed
.
-
(a) R. P. Yu, J. M. Darmon, C. Milsmann, G. W. Margulieux, S. C. E. Stieber, S. DeBeer and P. J. Chirik, J. Am. Chem. Soc., 2013, 135, 13168–13184 CrossRef CAS PubMed
;
(b) R. P. Yu, J. M. Darmon, J. M. Hoyt, G. W. Margulieux, Z. R. Turner and P. J. Chirik, ACS Catal., 2012, 2, 1760–1764 CrossRef CAS PubMed
.
-
(a) W. D. Clark, J. Cho, H. U. Valle, T. K. Hollis and E. J. Valente, J. Organomet. Chem., 2014, 751, 534–540 CrossRef CAS
;
(b) T. R. Helgert, T. K. Hollis and E. J. Valente, Organometallics, 2012, 31, 3002–3009 CrossRef CAS
;
(c) J. Cho, T. K. Hollis, E. J. Valente and J. M. Trate, J. Organomet. Chem., 2011, 696, 373–377 CrossRef CAS
;
(d) J. Cho, T. K. Hollis, T. R. Helgert and E. J. Valente, Chem. Commun., 2008, 5001–5003 RSC
.
-
(a) D. S. McGuinness, J. A. Suttil, M. G. Gardiner and N. W. Davies, Organometallics, 2008, 27, 4238–4247 CrossRef CAS
;
(b) D. S. McGuinness, V. C. Gibson and J. W. Steed, Organometallics, 2004, 23, 6288–6292 CrossRef CAS
;
(c) D. S. McGuinness, V. C. Gibson, D. F. Wass and J. W. Steed, J. Am. Chem. Soc., 2003, 125, 12716–12717 CrossRef CAS PubMed
.
-
(a) X. Gu, X. Zhu, Y. Wei, S. Wang, S. Zhou, G. Zhang and X. Mu, Organometallics, 2014, 33, 2372–2379 CrossRef CAS
;
(b) K. Lv and D. Cui, Organometallics, 2010, 29, 2987–2993 CrossRef CAS
.
-
(a) D. G. Brown, P. A. Schauer, J. Borau-Garcia, B. R. Fancy and C. P. Berlinguette, J. Am. Chem. Soc., 2013, 135, 1692–1695 CrossRef CAS PubMed
;
(b) D. G. Brown, N. Sanguantrakun, B. Schulze, U. S. Schubert and C. P. Berlinguette, J. Am. Chem. Soc., 2012, 134, 12354–12357 CrossRef CAS PubMed
;
(c) B. Schulze, D. Escudero, C. Friebe, R. Siebert, H. Görls, U. Köhn, E. Altuntas, A. Baumgaertel, M. D. Hager, A. Winter, B. Dietzek, J. Popp, L. González and U. S. Schubert, Chem. – Eur. J, 2011, 17, 5494–5498 CrossRef CAS PubMed
.
- J. Dinda, S. Liatard, J. Chauvin, D. Jouvenot and F. Loiseau, Dalton Trans., 2011, 40, 3683–3688 RSC
.
- S. U. Son, K. H. Park, Y.-S. Lee, B. Y. Kim, C. H. Choi, M. S. Lah, Y. H. Jang, D.-J. Jang and Y. K. Chung, Inorg. Chem., 2004, 43, 6896–6898 CrossRef CAS PubMed
.
-
(a) T. C. B. Harlang, Y. Liu, O. Gordivska, L. A. Fredin, C. S. Ponseca, P. Huang, P. Chábera, K. S. Kjaer, H. Mateos, J. Uhlig, R. Lomoth, R. Wallenberg, S. Styring, P. Persson, V. Sundström and K. Wärnmark, Nat. Chem., 2015, 7, 883–889 CrossRef CAS PubMed
;
(b) T. Duchanois, T. Etienne, C. Cebrián, L. Liu, A. Monari, M. Beley, X. Assfeld, S. Haacke and P. C. Gros, Eur. J. Inorg. Chem., 2015, 2015, 2469–2477 CrossRef CAS
;
(c) L. A. Fredin, M. Pápai, E. Rozsályi, G. Vankó, K. Wärnmark, V. Sundström and P. Persson, J. Phys. Chem. Lett., 2014, 5, 2066–2071 CrossRef CAS PubMed
; Y. Liu, T. Harlang, S. E. Canton, P. Chábera, K. Suárez-Alcántara, A. Fleckhaus, D. A. Vithanage, E. Göransson, A. Corani, R. Lomoth, V. Sundström and K. Wärnmark, Chem. Commun., 2013, 49, 6412–6413 Search PubMed
.
-
(a) X. Zhang, A. M. Wright, N. J. DeYonker, T. K. Hollis, N. I. Hammer, C. E. Webster and E. J. Valente, Organometallics, 2012, 31, 1664–1672 CrossRef CAS
;
(b) C.-S. Lee, R. R. Zhuang, S. Sabiah, J.-C. Wang, W.-S. Hwang and I. J. B. Lin, Organometallics, 2011, 30, 3897–3900 CrossRef CAS
;
(c) C.-S. Lee, S. Sabiah, J.-C. Wang, W.-S. Hwang and I. J. B. Lin, Organometallics, 2010, 29, 286–289 CrossRef CAS
.
- N. Darmawan, C.-H. Yang, M. Mauro, M. Raynal, S. Heun, J. Pan, H. Buchholz, P. Braunstein and L. De Cola, Inorg. Chem., 2013, 52, 10756–10765 CrossRef CAS PubMed
.
- See for example in related phosphine based pincers:
(a) L. S. H. Dixon, A. F. Hill, A. Sinha and J. S. Ward, Organometallics, 2014, 33, 653–658 CrossRef CAS
;
(b) T.-P. Lin and J. C. Peters, J. Am. Chem. Soc., 2013, 135, 15310–15313 CrossRef CAS PubMed
;
(c) M. Hasegawa, Y. Segawa, M. Yamashita and K. Nozaki, Angew. Chem., Int. Ed., 2012, 51, 6956–6960 CrossRef CAS PubMed
;
(d) Y. Segawa, M. Yamashita and K. Nozaki, J. Am. Chem. Soc., 2009, 131, 9201–9203 CrossRef CAS PubMed
;
(e) E. Morgan, D. F. MacLean, R. McDonald and L. Turculet, J. Am. Chem. Soc., 2009, 131, 14234–14236 CrossRef CAS PubMed
.
- R. E. Andrew and A. B. Chaplin, Dalton Trans., 2014, 43, 1413–1423 RSC
.
Footnote |
† Electronic supplementary information (ESI) available: Classification of articles by type of NHC-based pincer, metal, and application. See DOI: 10.1039/c5dt04429d |
|
This journal is © The Royal Society of Chemistry 2016 |