DOI:
10.1039/C6CE01416J
(Communication)
CrystEngComm, 2016,
18, 5807-5810
Halogen bonded polypseudorotaxanes based on a pillar[5]arene host†
Received
22nd June 2016
, Accepted 1st July 2016
First published on 1st July 2016
Abstract
Two crystalline supramolecular polypseudorotaxanes were obtained by combining permethylated pillar[5]arene as a macrocyclic wheel with 1,4-bis(1-imidazolyl)butane and 1,4-bis(iodoethynyl)benzene or 1,4-diiodo-1,3-butadiyne linked by C–I⋯N halogen bonds and creating a polyrotaxane axis. The resulting highly ordered supramolecular arrays were characterized by X-ray crystallography.
The synthesis of mechanically interlocked catenane and rotaxane assemblies has become a recent challenge in supramolecular chemistry not only for their unique structures but also due to their potential applications as molecular devices and functional materials.1,2 Among numerous interlocked structures constructed and studied in last few decades, polymers comprising rotaxane units have attracted considerable attention in the fields of materials science and nanotechnology.2,3 Polyrotaxanes and polypseudorotaxanes are constructed by threading macrocyclic rings, including cyclodextrins, crown ethers, cucurbiturils, calixarenes or pillararenes onto covalent type linear chains like polyethers, polyesters or polyamides, employing a variety of synthetic protocols. On the other hand, considerable efforts have been directed to the design of supramolecular polymers bearing rotaxane units self-assembled by highly directional and cooperative non-covalent interactions such as hydrogen bonding, host–guest interaction, π–π stacking or metal–ligand coordination.3–8 Due to the reversible, self-repairing and tunable nature of these interactions more advanced and useful supramolecular structures can be obtained in a relatively simple way. However, the vast majority of these studies have been performed in solution, where the molecules are in a constant flux, and the degree of oligomerization and the structure of oligomers are strongly concentration dependent.3–6 Despite the fact that for future applications materials with high and controllable structural regularity are required, there are very few well-characterized examples of crystalline polyrotaxanes including 1D, 2D and 3D arrays assembled by hydrogen bonding, metal–ligand or π–π stacking interactions.8
In this paper, we report the synthesis and structural characterization of two crystalline supramolecular polypseudorotaxanes by combining permethylated pillar[5]arene (MeP5A) as a macrocyclic wheel with 1,4-bis(1-imidazolyl)butane (1) and 1,4-bis(iodoethynyl)benzene (2) or 1,4-diiodo-1,3-butadiyne (3) linked by halogen bonds and creating a polyrotaxane axis. Pillararenes, a new family of macrocyclic hosts, due to their symmetrical structure and rigid electron-rich cavity are ideal candidates as host molecules for the construction of rotaxanes or pseudorotaxanes and the research interest in this class of compounds has grown rapidly.9 The facile preparation and excellent binding abilities of peralkylated pillararenes towards neutral guest molecules in organic solvents make them superior to water soluble cyclodextrins or cucurbiturils, possessing nearly the same cavity sizes as the host molecules for the fabrication of new supramolecular architectures. Thus we selected MeP5A as a host and bisimidazolyl derivative 1 as a guest due to their reported high association constant in nonpolar solvents [Ka of (4.7 ± 0.3) × 103 M−1].10 The encapsulated by multiple C–H⋯π and C–H⋯O interactions molecule of 1 possessing two unscreened imidazole nitrogen atoms should be able to further interact with halogen bond donors 2 or 3 and create a polymeric rotaxane axis. Halogen bonding has promising potential in supramolecular chemistry, particularly, as a design element in crystal engineering and molecular recognition.11 Heavier halogens, due to an anisotropic distribution of electrostatic potential, exhibit electrophilic characteristics and can interact with electron-pair donating heteroatoms (O, N, S) or anions. Since iodine atoms connected to C(sp) atoms form the strongest halogen bonds12 the iodoalkynes 2 and 3 were chosen as components of the polypseudorotaxane axis. Furthermore, due to a lesser steric overcrowding they are better accessible to the imidazole nitrogen atoms of 1 than the iodine atoms in fluorinated iodobenzenes, most frequently used as halogen bond donors.13 Obtaining three component co-crystals with predictable connectivity is obviously an extremely difficult task.14 Indeed, our initial attempt to crystallize an equimolar mixture of MeP5A, 1 and 2 afforded only a binary complex 1·2 composed of infinite chains of the halogen bonded components. However, preparation of pseudorotaxane MeP5A·1 from equimolar amounts of MeP5A and 1 in toluene–chloroform followed by its cocrystallization with 2 from the 1
:
2 mixture of tetrachloromethane and dichloromethane gave solvated crystals of the polypseudorotaxane MeP5A·1·2·(CCl4)2. The second ternary complex MeP5A·1·3·(toluene)1.5 was obtained in an analogous way (Fig. 2).‡
Triclinic crystals of MeP5A·1·2§ and MeP5A·1·3¶ contain infinite chains of alternating 1 and 2 or 1 and 3 molecules, respectively, connected by halogen bonds forming the polypseudorotaxane axis with the threaded pillararene MeP5A beads (Fig. 1). The halogen bonds connecting 1 with 2 or 1 with 3 are nearly linear (the C–I⋯N angles are 172°) and quite strong as evidenced by the I⋯N distances of 2.734–2.794 Å (77–79% of the sum of the van der Waals radii of I and N).15 The diimidazolylbutane unit 1 is accommodated centrally within the macrocycle with the MeP5A mean plane passing through the middle of the aliphatic (CH2)4 chain. Nevertheless the conformation of 1 differs in the two crystal structures: in MeP5A·1·2 the aliphatic chain of 1 adopts a folded conformation, whereas in MeP5A·1·3 it is fully extended.||
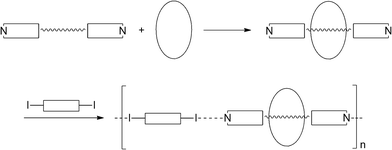 |
| Fig. 1 Halogen bonded polypseudorotaxane formation in the solid state. | |
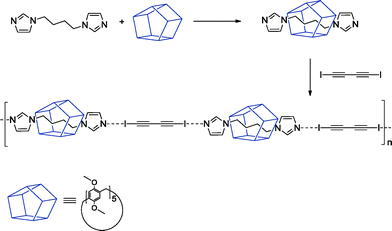 |
| Fig. 2 Schematic representation of the approach for the formation of designed polypseudorotaxanes. | |
It is noteworthy that pillararene molecules assume a planar chiral conformation and their racemization occurs by rotation of the hydroquinone units.9a,16 Obviously, the synthesized pillar[5]arenes are racemic mixtures. Thus a close inspection of the structure of MeP5A·1·2 reveals that the polyrotaxane thread is composed of alternating pR and pS enantiomers of the MeP5A units. Furthermore, the pillararene chirality induces chiral conformations of the included guest molecules of 1 (Fig. 3). In contrast, the MeP5A·1·2 threads are built from the homochiral MeP5A molecules and the neighboring threads contain the molecules of the opposite chirality.
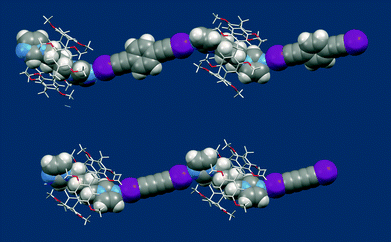 |
| Fig. 3 Molecular structures of polypseudorotaxanes MeP5A·1·2 (top) and MeP5A·1·3 (bottom) formed via halogen bonds. | |
It should be emphasized that the above polypseudorotaxane structures exist only in the solid state and their dissolution simply results in their dissociation and disassembly. This is due to the relatively low association constants of iodoethynyl derivatives and halogen bond acceptors in solution.17 The only interaction between the components that occurs in solution is a complexation equilibrium between pillararene MeP5A and bisimidazole 1 that is reflected by the corresponding 1H and 13C NMR spectra (see the ESI†).9a
In summary, we have presented the first two polypseudorotaxanes containing pillararene beads self-assembled with use of halogen bonds. A key to the success of the self-assembly of three-component supermolecules was the high affinity of pillararene MeP5A towards diimidazolylbutane derivative 1 which allowed the formation of a relatively stable pseudorotaxane. In addition, the highly symmetrical structure of the pillararene and its solubility in organic solvents facilitated the manipulation and crystallization of the complexes with structural regularity. Our work has demonstrated that with use of crystal engineering methods highly ordered and predictable complex supramolecular arrays may be readily accessible in a relatively simple way.
Acknowledgements
We are indebted to Dr. Casimir Antczak for critical reading of the manuscript.
Notes and references
-
(a) D. B. Amabilino and J. F. Stoddart, Chem. Rev., 1995, 95, 2725 CrossRef CAS;
(b) M. Xue, Y. Yang, X. Chi, X. Yan and F. Huang, Chem. Rev., 2015, 115, 7398 CrossRef CAS PubMed.
-
(a) T. Takata, Polym. J., 2006, 38, 1 CrossRef CAS;
(b) F. M. Raymo and J. F. Stoddart, Chem. Rev., 1999, 99, 1643 CrossRef CAS PubMed.
-
(a) A. Harada, A. Hashidzume, H. Yamaguchi and Y. Takashima, Chem. Rev., 2009, 109, 5974 CrossRef CAS PubMed;
(b) A. Harada, Y. Takashima and H. Yamaguchi, Chem. Soc. Rev., 2009, 38, 875 RSC;
(c) C. Li, Chem. Commun., 2014, 50, 12420 RSC;
(d) L. Yang, X. Tan, Z. Wang and X. Zhang, Chem. Rev., 2015, 115, 7196 CrossRef CAS PubMed.
-
(a) M. Miyauchi and A. Harada, J. Am. Chem. Soc., 2004, 126, 11418 CrossRef CAS PubMed;
(b) M. Miyauchi, T. Hoshino, H. Yamaguchi, S. Kamitori and A. Harada, J. Am. Chem. Soc., 2005, 127, 2034 CrossRef CAS PubMed;
(c) Y. Hasegawa, M. Miyauchi, Y. Takashima, H. Yamaguchi and A. Harada, Macromolecules, 2005, 38, 3724 CrossRef CAS;
(d) K. Ohga, Y. Takashima, H. Takahashi, Y. Kawaguchi, H. Yamaguchi and A. Harada, Macromolecules, 2005, 38, 5897 CrossRef CAS;
(e) P. Kuad, A. Miyawaki, Y. Takashima, H. Yamaguchi and A. Harada, J. Am. Chem. Soc., 2007, 129, 12630 CrossRef CAS PubMed;
(f) A. Miyawaki, M. Miyauchi, Y. Takashima, H. Yamaguchi and A. Harada, Chem. Commun., 2008, 456 RSC.
-
(a) Z. Zhang, Y. Luo, J. Chen, S. Dong, Y. Yu, Z. Ma and F. Huang, Angew. Chem., Int. Ed., 2011, 50, 1397 CrossRef CAS PubMed;
(b) N. L. Strutt, H. Zhang, M. A. Giesener, J. Leia and J. F. Stoddart, Chem. Commun., 2012, 48, 1647 RSC;
(c) T. Ogoshi, H. Kayama, D. Yamafuji, T. Aoki and T. Yamagishi, Chem. Sci., 2012, 3, 3221 RSC;
(d) Y. Guan, M. Ni, X. Hu, T. Xiao, S. Xiong, C. Lin and L. Wang, Chem. Commun., 2012, 48, 8529 RSC;
(e) B. Xia, B. Zheng, C. Han, S. Dong, M. Zhang, B. Hu, Y. Yub and F. Huang, Polym. Chem., 2013, 4, 2019 RSC;
(f) C. Han, B. Xia, J. Chen, G. Yu, Z. Zhang, S. Dong, B. Hu, Y. Yub and M. Xue, RSC Adv., 2013, 3, 16089 RSC;
(g) C. Li, K. Han, J. Li, Y. Zhang, W. Chen, Y. Yu and X. Jia, Chem. – Eur. J., 2013, 19, 11892 CrossRef CAS PubMed;
(h) S. Wang, Y. Wang, Z. Chen, Y. Lin, L. Weng, K. Han, J. Li, X. Jia and C. Li, Chem. Commun., 2015, 51, 3434 RSC;
(i) B. Shi, K. Jie, Y. Zhou, D. Xia and Y. Yao, Chem. Commun., 2015, 51, 4503 RSC.
-
(a) X.-Y. Hu, X. Wu, Q. Duan, T. Xiao, C. Lin and L. Wang, Org. Lett., 2012, 4826 CrossRef CAS PubMed;
(b) X.-Y. Hu, P. Zhang, X. Wu, W. Xia, T. Xiao, J. Jiang, C. Lin and L. Wang, Polym. Chem., 2012, 3, 3060 RSC;
(c) X.-Y. Hu, X. Wu, S. Wang, D. Chen, W. Xia, C. Lin, Y. Pan and L. Wang, Polym. Chem., 2013, 4, 4292 RSC.
-
(a) Y. Liu, Y.-L. Zhao, H.-Y. Zhang and H.-B. Song, Angew. Chem., Int. Ed., 2003, 42, 3260 CrossRef CAS PubMed;
(b) Y. Liu, S.-H. Song, Y. Chen, Y.-L. Zhao and Y.-W. Yang, Chem. Commun., 2005, 1702 RSC.
-
(a) M. Asakawa, P. R. Ashton, G. R. Brown, W. Hayes, S. Menzer, J. F. Stoddart, A. J. P. White and D. J. Williams, Adv. Mater., 1996, 8, 37 CrossRef CAS;
(b) D. Whang, Y.-M. Jeon, J. Heo and K. Kim, J. Am. Chem. Soc., 1996, 118, 11333 CrossRef CAS;
(c) D. Whang and K. Kim, J. Am. Chem. Soc., 1997, 119, 451 CrossRef CAS;
(d) E. Lee, J. Heo and K. Kim, Angew. Chem., Int. Ed., 2000, 39, 2699 CrossRef CAS;
(e) C. S. A. Fraser, M. C. Jennings and R. J. Puddephatt, Chem. Commun., 2001, 1310 RSC;
(f) G. J. E. Davidson and S. J. Loeb, Angew. Chem., Int. Ed., 2003, 42, 74 CrossRef CAS;
(g) D. J. Hoffart and S. J. Loeb, Angew. Chem., Int. Ed., 2005, 44, 901 CrossRef CAS PubMed;
(h) H.-Y. Gong, B. M. Rambo, W. Cho, V. M. Lynch, M. Oh and J. L. Sessler, Chem. Commun., 2011, 47, 5973 RSC;
(i) X.-S. Wu, J. Liang, X.-L. Hu, X.-L. Wang, B.-Q. Song, Y.-Q. Jiao and Z.-M. Su, Cryst. Growth Des., 2015, 15, 4311 CrossRef CAS.
-
(a) M. Xue, Y. Yang, X. Chi, Z. Zhang and F. Huang, Acc. Chem. Res., 2012, 47, 1294 CrossRef PubMed;
(b) T. Ogoshi and T. Yamagishi, Eur. J. Org. Chem., 2013, 2961 CrossRef CAS;
(c) N. L. Strutt, H. Zhang, S. T. Schneebeli and J. F. Stoddart, Acc. Chem. Res., 2014, 47, 2631 CrossRef CAS PubMed;
(d) L.-L. Tan and Y.-W. Yang, J. Inclusion Phenom. Macrocyclic Chem., 2015, 81, 13 CrossRef CAS.
-
(a) C. Li, S. Chen, J. Li, K. Han, M. Xu, B. Hu, Y. Yuc and X. Jia, Chem. Commun., 2011, 47, 11294 RSC;
(b) K. Han, Y. Zhang, J. Li, Y. Yu, X. Jia and C. Li, Eur. J. Org. Chem., 2013, 2057 CrossRef CAS;
(c) The perethylated pillar[5]arene (EtP5A) has a four times higher value of association constant compared with 1 but in the case of MeP5A the nitrogen atoms of the guest molecule are better accessible to halogen bond donors.
-
(a)
P. Metrangolo and G. Resnati, Halogen Bonding: Fundamentals and Applications (Stucture and Bonding), Springer, Heidelberg, 2010 Search PubMed;
(b) A. Priimagi, G. Cavallo, P. Metrangolo and G. Resnati, Acc. Chem. Res., 2013, 46, 2686 CrossRef CAS PubMed;
(c) L. C. Gilday, S. W. Robinson, T. A. Barendt, M. J. Langton, B. R. Mullaney and P. D. Beer, Chem. Rev., 2015, 115, 7118 CrossRef CAS PubMed;
(d) G. Cavallo, P. Metrangolo, R. Milani, T. Pilati, A. Priimagi, G. Resnati and G. Terraneo, Chem. Rev., 2016, 116, 2478 CrossRef CAS PubMed.
- E. Bosh, Cryst. Growth Des., 2014, 14, 126 Search PubMed.
- Our attempts to use 1,4-diiodotetrafluorobenzene (4) as the axis component afforded the crystals of MeP5A·1·42·2 (H2O), where the pseudorotaxane MeP5A·1 is halogen bonded to only one molecule of 4 (the C–I⋯N halogen bond is relatively weak; I⋯N 3.311 Å) and the water molecules are hydrogen bonded to the imidazole nitrogen of 1 and halogen bonded to the iodine atoms of 4 (I⋯O 2.885–2.978 Å). For more details see the ESI†.
-
(a) C. B. Aakeröy, A. M. Beatty and B. A. Helfrich, Angew. Chem., Int. Ed., 2001, 40, 3240 CrossRef;
(b) C. B. Aakeröy, J. Desper and J. F. Urbina, Chem. Commun., 2005, 2820 RSC.
- A. Bondi, J. Phys. Chem., 1966, 70, 3006 CrossRef CAS.
-
(a) T. Ogoshi, K. Masaki, R. Shiga, K. Kitajima and T. Yamagishi, Org. Lett., 2011, 13, 1264 CrossRef CAS PubMed;
(b) N. L. Strutt, D. Fairen-Jimenez, J. Iehl, M. B. Lalonde, R. Q. Snurr, O. K. Farha, J. T. Hupp and J. F. Stoddart, J. Am. Chem. Soc., 2012, 134, 17436 CrossRef CAS PubMed.
- O. Dumele, D. Wu, N. Trapp, N. Goroff and F. Diederich, Org. Lett., 2014, 16, 4722 CrossRef CAS PubMed.
-
CrysAlis PRO Software, Agilent Technologies, Yarnton, Oxfordshire, England, 2014 Search PubMed.
- M. C. Burla, R. Caliandro, M. Camalli, B. Carrozzini, G. L. Cascaran, L. De Caro, C. Giacovazzo, G. Polidori and R. J. Spagna, J. Appl. Crystallogr., 2005, 38, 381 CrossRef CAS.
- G. M. Sheldrick, Acta Crystallogr., Sect. C: Struct. Chem., 2015, 71, 3 CrossRef PubMed.
- O. V. Dolomanov, L. J. Bourhis, R. J. Gildea, J. A. K. Howard and H. J. Puschmann, J. Appl. Crystallogr., 2009, 42, 339 CrossRef CAS.
- C. F. Macrae, I. J. Bruno, J. A. Chisholm, P. R. Edgington, P. McCabe, E. Pidcock, L. Rodriguez-Monge, R. Taylor, J. van de Streek and P. A. Wood, J. Appl. Crystallogr., 2008, 41, 466 CrossRef CAS.
Footnotes |
† Electronic supplementary information (ESI) available: Experimental details, geometry of halogen and hydrogen bonds and crystal structure of MeP5A·1·42. CCDC 1465200, 1465201 and 1469513 contain the supplementary crystallographic data for this paper. For ESI and crystallographic data in CIF or other electronic format see DOI: 10.1039/c6ce01416j |
‡ X-Ray diffraction data were collected with an Oxford Diffraction Supernova diffractometer and processed with the CrysAlis software.18 The crystal structures were solved with SIR2004 (ref. 19) and refined by full-matrix least-squares with SHELXL-2014 (ref. 20) within Olex-2.21 Drawings were prepared with Mercury22 software. |
§ Crystal data for MeP5A·1·2: C45H50O10·C10H14N4·C10H4I2·2(CCl4) (M = 1626.65 g mol−1), crystal size 0.3 × 0.2 × 0.02 mm3, triclinic, space group P (no. 2), a = 12.6235(2) Å, b = 16.2250(4) Å, c = 18.6220(4) Å, α = 90.7497(18)°, β = 103.7900(18)°, γ = 102.4114(17)°, V = 3609.24(13) Å3, Z = 2, Dc = 1.497 g cm−3, μ(CuKα) = 10.036 mm−1, T = 130 K, Cu Kα radiation (λ = 1.54184 Å), 58 888 reflections measured (9.84° ≤ 2Θ ≤ 149.008°), 14 769 unique (Rint = 0.0590, Rsigma = 0.0489) which were used in all calculations. The final R1 was 0.0544 (I > 2σ(I)) and wR2 was 0.1513 (all data). One of the methoxy methyl groups and one of the imidazolyl groups were refined as disordered over two positions. |
¶ Crystal data for MeP5A·1·3: C45H50O10·C10H14N4·C4I2·1.5(C7H8) (M = 2762.28 g mol−1), crystal size 0.4 × 0.35 × 0.2 mm3, triclinic, space group P (no. 2), a = 11.6992(1) Å, b = 14.9802(2) Å, c = 20.2256(3) Å, α = 72.161(1)°, β = 83.489(1)°, γ = 73.806(1)°, V = 3238.85(7) Å3, Z = 2, Dc = 1.416 g cm−3, μ(CuKα) = 8.116 mm−1, T = 130 K, Cu Kα radiation (λ = 1.54184 Å), 80 767 reflections measured (4.59° ≤ 2Θ ≤ 153.03°), 13 528 unique (Rint = 0.0537, Rsigma = 0.0239) which were used in all calculations. The final R1 was 0.0359 (I > 2σ(I)) and wR2 was 0.0991 (all data). Both toluene molecules are disordered and one of them is disordered around an inversion center. |
|| Crystal data for MeP5A·1·42: C45H50O10·C10H14N4·2(C6F4I2)·2(H2O) (M = 1780.85 g mol−1): orthorhombic, space group Pbcn (no. 60), a = 20.04603(13) Å, b = 23.88917(14) Å, c = 14.22073(10) Å, V = 6810.07(8) Å3, Z = 4, Dc = 1.737 g cm−3, μ(CuKα) = 15.107 mm−1, T = 130 K, Cu Kα radiation (λ = 1.54184 Å), 66 932 reflections measured (13.10° ≤ 2Θ ≤ 149.00°), 6952 unique (Rint = 0.0498, Rsigma = 0.0213) which were used in all calculations. The final R1 was 0.0460 (I > 2σ(I)) and wR2 was 0.1041 (all data). The molecule of 1 and one of the water molecules have half occupancy and are disordered over a twofold symmetry axis. |
|
This journal is © The Royal Society of Chemistry 2016 |
Click here to see how this site uses Cookies. View our privacy policy here.