DOI:
10.1039/C6CC03468C
(Communication)
Chem. Commun., 2016,
52, 9777-9780
C–H arylations of 1,2,3-triazoles by reusable heterogeneous palladium catalysts in biomass-derived γ-valerolactone†
Received
25th April 2016
, Accepted 4th July 2016
First published on 8th July 2016
Abstract
C–H arylations were accomplished with a user-friendly heterogeneous palladium catalyst in the biomass-derived γ-valerolactone (GVL) as an environmentally-benign reaction medium. The user-friendly protocol was characterized by ample substrate scope and high functional group tolerance in the C–H arylation of 1,2,3-triazoles, and the palladium catalyst could be recycled and reused in the C–H activation process.
Fully functionalized 1,2,3-triazoles1 constitute key structural motifs in various applied areas, such as medicinal chemistry, bioorganic chemistry, and material sciences, among others.2 The copper(I)-catalyzed azide–alkyne 1,3-dipolar cycloaddition3 (CuAAC)4,5 has emerged as the most valuable tool for the preparation of 1,2,3-triazoles with high levels of regio control.6,7 However, the CuAAC approach is largely8 limited to terminal alkynes and, hence, fails short in providing general access to fully trisubstituted triazoles. In recent years, catalyzed C–H activations have been identified as a transformative platform for the atom-9 and step-economical10 preparation of heterocyclic compounds.11 Particularly, the nexus of CuAAC and C–H functionalization technology proved instrumental for the efficient assembly of fully decorated 1,2,3-triazoles with excellent levels of positional selectivity.12 Hence, copper-13,14 and palladium-based15–22 catalysts were shown to enable the site-selective C–H arylation of 1,2,3-triazoles.23 Despite these undisputable advances, C–H arylations on 1,2,3-triazoles were thus far solely accomplished with homogeneous catalysts, rendering a recycling and reuse of the metal catalysts challenging, while, at the same time, leading to considerable amounts of undesired metal impurities in the target products. Moreover, the catalyzed C–H functionalizations of 1,2,3-triazoles were predominantly performed in dipolar aprotic solvents, such as dimethylformamide (DMF), N-methylpyrroldin-2-one (NMP) and N,N-dimethylacetamide (DMA). Unfortunately, these solvents face considerable environmental and safety issues, which is of particular relevance for the practitioner in academia and industries.24 Within our program directed towards sustainable C–H activation technology,25,26 we have developed the first triazole C–H arylation by the aid of a recyclable heterogeneous27–31 catalyst (Fig. 1). Thus, a versatile palladium catalyst was effectively reused in the C–H activation of synthetically meaningful 1,2,3-triazoles. Importantly, we herein also describe the use of bio-based γ-valerolactone (GVL)32,33 – available from renewable lignocellulosic biomass34,35 – as an environmentally-sound medium in direct C–H arylation.
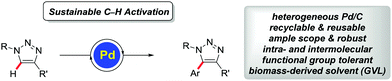 |
| Fig. 1 Sustainable heterogeneous C–H arylation in GVL. | |
At the outset of our studies, we optimized reaction conditions for the envisioned palladium-catalyzed C–H arylation of triazole 1a with aryl bromide 2a in the biomass-derived GVL as the solvent (Table 1). The C–H arylation occurred smoothly by means of palladium on charcoal catalysis in the presence of the carboxylic acid MesCO2H as the cocatalyst36 and with K2CO3 as the base, thereby delivering the desired product 3aa (entries 1–3). The C–H functionalization proceeded with excellent positional selectivity, and only trace amounts of the diarylated product 4aa were detected (entry 3). Among a representative set of bases (entries 3–9), K2CO3 and KTFA furnished optimal results (entries 7 and 9), with a slightly improved efficacy at a higher reaction temperature (entries 3 and 7).
Table 1 Optimization of palladium-catalyzed C–H arylations in GVLa

|
Entry |
Base |
3aa
(%) |
4aa
(%) |
Reaction conditions: 1a (0.25 mmol), 2a (0.75 mmol), Pd/C (5.0 mol%), MesCO2H (30 mol%), base (3 equiv.), GVL (1.0 mL), 150 °C, 16 h.
1H NMR conversion with CH2Br2 as internal standard, yields of isolated products are given in parentheses.
130 °C.
|
1 |
NEt3c |
— |
— |
2 |
Cs2CO3c |
— |
— |
3 |
K2CO3c |
70 |
8 |
4 |
NH4OAc |
— |
— |
5 |
Na2CO3 |
45 |
5 |
6 |
KHCO3 |
78 |
14 |
7
|
K
2
CO
3
|
82 (55)
|
16 (4)
|
8 |
KOAc |
66 (42) |
16 (4) |
9
|
KTFA
|
86 (63)
|
14 (8)
|
With the optimized reaction conditions in hand, we initially probed the catalyst's versatility in the C–H arylation of N-alkyl-substituted 1,2,3-triazoles 1a–1d in GVL (Scheme 1). Thus, both mono- and 1,4-di-substituted 1,2,3-triazoles 1a,b were efficiently converted. The triazole 1b displaying two alkyl-substituents delivered the corresponding products 3bb–3bh selectively as the sole products. Here, the robust nature of the heterogeneous palladium catalyst was reflected by fully tolerating valuable electrophilic functional groups, such as chloro, ester or enolizable ketone substituents. Likewise, the hindered 2-naphthyl electrophile 2h was transformed with high catalytic efficacy, as were alkyl-substituted 1,2,3-triazoles 1c,d.
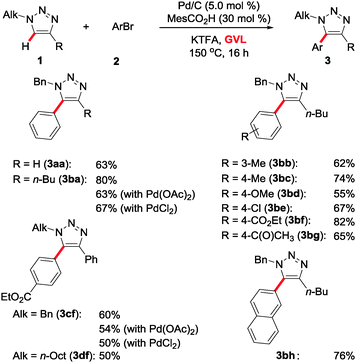 |
| Scheme 1 C–H arylation of N-alkyl triazoles 1 in GVL. | |
Subsequently, we evaluated the power of the Pd/C catalyst in the C–H functionalization of 1,2,3-triazole 1e–1k bearing N-aryl motifs (Scheme 2). Hence, differently decorated arenes were well tolerated by the user-friendly catalyst, enabling the synthesis of regio-selectively arylated products 3 with excellent positional control. Substrates 1f–1j with electron-withdrawing or electron-donating N-aryl groups furnished the desired tri-substituted 1,2,3-triazoles 3ef–3jf, again featuring good functional group tolerance. Thereby, our strategy provided atom-economical access to the selectively tri-arylated 1,2,3-triazole 3kf as well.
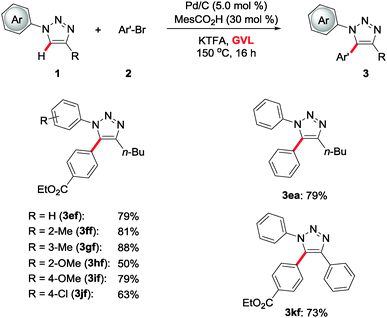 |
| Scheme 2 C–H arylation of N-arylated triazoles 1 in GVL. | |
The heterogeneous catalyst was not restricted to intermolecular C–H arylations in GVL. Indeed, the intramolecular C–H functionalization with substrate 4a proved viable with comparable levels of efficacy, thereby delivering the triazolo[1,5-a]isoindole 5a (Scheme 3).
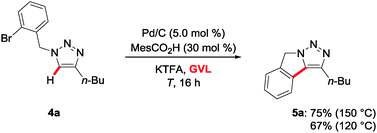 |
| Scheme 3 Intramolecular C–H arylation in GVL. | |
In consideration of the remarkable efficacy of the versatile palladium C–H activation catalyst, we became attracted to probing its recyclability and reusability. To this end, we developed an effective protocol for the recycle of the heterogeneous palladium catalyst (Table 2), thereby allowing for the robust reuse of the catalyst. It is noteworthy that only a minor amount of palladium was detected by detailed ICP-MS analysis of the crude product.37 This observation indicated only minor leaching,38 that is within the specifications for active pharmaceutical ingredients produced by palladium-catalyzed processes.39 Our findings were further in line with a hot-filtration test and mercury poisoning studies,37 which provided strong support for a heterogeneous mode of action. Likewise, the three-phase test suggested that no active homogeneous palladium species were formed.37
Table 2 Recovery and reuse of palladium catalysta
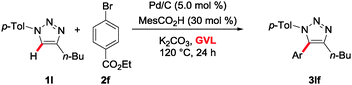
|
Run |
1st |
2nd |
3rd |
Reaction conditions: 1l (0.30 mmol), 2f (0.45 mmol), Pd/C (5.0 mol%), MesCO2H (30 mol%), K2CO3 (0.60 mmol), GVL (2.0 mL), 120 °C, 24 h.
By ICP-MS analysis.37
|
3lf (%) |
90 |
90 |
90 |
Pd-leachingb (ppm) |
5.5 |
4.1 |
3.6 |
In summary, we have developed the first C–H arylation of 1,2,3-triazoles by a heterogeneous catalyst in environmentally-sound γ-valerolactone (GVL)40 as the reaction medium. Thus, a broadly applicable palladium catalyst allowed for inter- as well as intramolecular C–H functionalizations with ample scope. The biomass-derived solvent further set the stage for the efficient reuse of the heterogeneous palladium catalyst in positional selective C–H activations. The use of the biomass-based GVL as environmentally-benign solvent in C–H functionalization technology should prove instrumental for the future development of sustainable processes.41
Generous support by the European Research Council under the European Community's Seventh Framework Program (FP7 2007–2013)/ERC Grant agreement no. 307535, the Alexander von Humboldt foundation (fellowship to X. T.), and the CSC (fellowship to F. Y.) is gratefully acknowledged. Further, we thank the Università degli Studi di Perugia, the EC 7th Framework Program project REGPOT-CT-2013-316149 Innovabalt, and the “Fondazione Cassa di Risparmio di Terni e Narni” for financial support.
References
- Illustrative reviews on 1,2,3-triazoles:
(a) K. D. Hanni and D. A. Leigh, Chem. Soc. Rev., 2010, 39, 1240–1251 RSC;
(b) M. Meldal and C. W. Tornoe, Chem. Rev., 2008, 108, 2952–3015 CrossRef CAS PubMed;
(c) Y. L. Angell and K. Burgess, Chem. Soc. Rev., 2007, 36, 1674–1689 RSC;
(d) H. C. Kolb and K. B. Sharpless, Drug Discovery Today, 2003, 8, 1128–1137 CrossRef CAS PubMed , and references cited therein.
- Illustrative reviews on syntheses and applications of 1,2,3-triazoles:
(a) E. Haldon, M. C. Nicasio and P. J. Pérez, Org. Biomol. Chem., 2015, 13, 9528–9550 RSC;
(b) F. Alonso, Y. Moglie and G. Radivoy, Acc. Chem. Res., 2015, 48, 2516–2528 CrossRef CAS PubMed;
(c) A. Qin, Y. Liu and B. Z. Tang, Macromol. Chem. Phys., 2015, 216, 818–828 CrossRef CAS;
(d) D. Astruc, L. Liang, A. Rapakousiou and J. Ruiz, Acc. Chem. Res., 2012, 45, 630–640 CrossRef CAS PubMed;
(e) A. H. El-Sagheer and T. Brown, Chem. Soc. Rev., 2010, 39, 1388–1405 RSC;
(f) A. Qin, J. W. Y. Lam and B. Z. Tang, Chem. Soc. Rev., 2010, 39, 2522–2544 RSC;
(g) H. Nandivada, X. Jiang and J. Lahann, Adv. Mater., 2007, 19, 2197–2208 CrossRef CAS , and references cited therein.
- R. Huisgen, Angew. Chem., 1963, 75, 604–637 CrossRef CAS.
- V. V. Rostovtsev, L. G. Green, V. V. Fokin and K. B. Sharpless, Angew. Chem., Int. Ed., 2002, 41, 2596–2599 CrossRef CAS.
- C. W. Tornoe, C. Christensen and M. Meldal, J. Org. Chem., 2002, 67, 3057–3064 CrossRef CAS PubMed.
- J. E. Hein and V. V. Fokin, Chem. Soc. Rev., 2010, 39, 1302–1315 RSC.
- M. Meldal and C. W. Tornøe, Chem. Rev., 2008, 108, 2952–3015 CrossRef CAS PubMed.
- For recent progress, see:
(a) W. Wang, X. Peng, F. Wei, C.-H. Tung and Z. Xu, Angew. Chem., Int. Ed., 2016, 55, 649–653 CrossRef CAS PubMed;
(b) S. Ding, G. Jia and J. Sun, Angew. Chem., Int. Ed., 2014, 126, 1908–1911 CrossRef;
(c) B. T. Worrell, J. E. Hein and V. V. Fokin, Angew. Chem., Int. Ed., 2012, 51, 11791–11794 CrossRef CAS PubMed.
- B. M. Trost, Acc. Chem. Res., 2002, 35, 695–705 CrossRef CAS PubMed.
- P. A. Wender, V. A. Verma, T. J. Paxton and T. H. Pillow, Acc. Chem. Soc., 2008, 41, 40–49 CrossRef CAS PubMed.
- Representative reviews on C–H activation:
(a) J. G. Kim, K. Shin and S. Chang, Top. Organomet. Chem., 2016, 55, 29–51 CrossRef;
(b) C. Borie, L. Ackermann and M. Nechab, Chem. Soc. Rev., 2016, 45, 1368–1386 RSC;
(c) O. Daugulis, J. Roane and L. D. Tran, Acc. Chem. Res., 2015, 48, 1053–1064 CrossRef CAS PubMed;
(d) Y. Segawa, T. Maekawa and K. Itami, Angew. Chem., Int. Ed., 2015, 54, 66–81 CrossRef CAS PubMed;
(e) N. Kuhl, N. Schroeder and F. Glorius, Adv. Synth. Catal., 2014, 356, 1443–1460 CrossRef CAS;
(f) S. A. Girard, T. Knauber and C.-J. Li, Angew. Chem., Int. Ed., 2014, 53, 74–100 CrossRef CAS PubMed;
(g) J. Wencel-Delord and F. Glorius, Nat. Chem., 2013, 5, 369–375 CrossRef CAS PubMed;
(h) K. M. Engle, T.-S. Mei, M. Wasa and J.-Q. Yu, Acc. Chem. Res., 2012, 45, 788–802 CrossRef CAS PubMed;
(i) T. Satoh and M. Miura, Chem. – Eur. J., 2010, 16, 11212–11222 CrossRef CAS PubMed;
(j) T. W. Lyons and M. S. Sanford, Chem. Rev., 2010, 110, 1147–1169 CrossRef CAS PubMed;
(k) L. Ackermann, R. Vicente and A. Kapdi, Angew. Chem., Int. Ed., 2009, 48, 9792–9826 CrossRef CAS PubMed;
(l) X. Chen, K. M. Engle, D.-H. Wang and J.-Q. Yu, Angew. Chem., Int. Ed., 2009, 48, 5094–5115 CrossRef CAS PubMed;
(m) R. G. Bergman, Nature, 2007, 446, 391–393 CrossRef CAS PubMed , and references cited therein.
- A review: L. Ackermann and H. K. Potukuchi, Org. Biomol. Chem., 2010, 8, 4503–4513 CAS.
- L. Ackermann, H. K. Potukuchi, D. Landsberg and R. Vicente, Org. Lett., 2008, 10, 3081–3084 CrossRef CAS PubMed.
- R. Jeyachandran, H. K. Potukuchi and L. Ackermann, Beilstein J. Org. Chem., 2012, 8, 1771–1777 CrossRef CAS PubMed.
- S. Chuprakov, N. Chernyak, A. S. Dudnik and V. Gevorgyan, Org. Lett., 2007, 9, 2333–2336 CrossRef CAS PubMed.
- M. Iwasaki, H. Yorimitsu and K. Oshima, Chem. – Asian J., 2007, 2, 1430–1435 CrossRef CAS PubMed.
- L. Ackermann, R. Vicente and R. Born, Adv. Synth. Catal., 2008, 350, 741–748 CrossRef CAS.
- L. Ackermann, A. Althammer and S. Fenner, Angew. Chem., Int. Ed., 2009, 48, 201–204 CrossRef CAS PubMed.
- L. Ackermann and R. Vicente, Org. Lett., 2009, 11, 4922–4925 CrossRef CAS PubMed.
- F. Wei, H. Li, C. Song, Y. Ma, L. Zhou, C.-H. Tung and Z. Xu, Org. Lett., 2015, 17, 2860–2863 CrossRef CAS PubMed.
- J. M. Schulman, A. A. Friedman, J. Panteleev and M. Lautens, Chem. Commun., 2012, 48, 55–57 RSC.
- B. T. Liégault, D. Lapointe, L. Caron, A. Vlassova and K. Fagnou, J. Org. Chem., 2009, 74, 1826–1834 CrossRef PubMed.
- For examples of directed ruthenium-catalyzed C–H arylations of 1,2,3-triazoles, see:
(a) C. Tirler and L. Ackermann, Tetrahedron, 2015, 71, 4543–4551 CrossRef CAS;
(b) X. G. Li, K. Liu, G. Zou and P. N. Liu, Eur. J. Org. Chem., 2014, 7878–7888 CrossRef CAS;
(c) L. Ackermann, R. Vicente, H. K. Potukuchi and V. Pirovano, Org. Lett., 2010, 12, 5032–5035 CrossRef CAS PubMed;
(d) L. Ackermann, P. Novák, R. Vicente, V. Pirovano and H. K. Potukuchi, Synthesis, 2010, 2245–2253 CrossRef CAS;
(e) L. Ackermann, R. Born and R. Vicente, ChemSusChem, 2009, 2, 546–549 CrossRef CAS PubMed;
(f) L. Ackermann, R. Vicente and A. Althammer, Org. Lett., 2008, 10, 2299–2302 CrossRef CAS PubMed.
- D. Prat, A. Wells, J. Hayler, H. Sneddon, C. R. McElroy, S. Abou-Shehadad and P. J. Dunn, Green Chem., 2016, 18, 288–296 RSC.
- L. Ackermann, Acc. Chem. Res., 2014, 47, 281–295 CrossRef CAS PubMed.
- L. Ackermann, Synlett, 2007, 507–526 CrossRef CAS.
- R. Cano, A. F. Schmidt and G. P. McGlacken, Chem. Sci., 2015, 6, 5338–5346 RSC.
- S. Santoro, S. Kozhushkov, L. Ackermann and L. Vaccaro, Green Chem., 2016, 18, 3471–3493 RSC.
- A. J. Reay and I. J. S. Fairlamb, Chem. Commun., 2015, 51, 16289–16307 RSC.
- L. Djakovitch and F.-X. Felpin, ChemCatChem, 2014, 6, 2175–2187 CrossRef CAS.
- For examples of palladium-catalyzed heterogenous C–H functionalizations:
(a) V. Pascanu, F. Carson, M. V. Solano, J. Su, X. Zou, M. J. Johansson and B. Martin-Matute, Chem. – Eur. J., 2016, 22, 3729–3737 CrossRef CAS PubMed;
(b) Z. Shu, W. Li and B. Wang, ChemCatChem, 2015, 7, 605–608 CrossRef CAS;
(c) J. Chen, L. He, K. Natte, H. Neumann, M. Beller and X.-F. Wu, Adv. Synth. Catal., 2014, 356, 2955–2959 CrossRef CAS;
(d) D.-T. D. Tang, K. D. Collins, J. B. Ernst and F. Glorius, Angew. Chem., Int. Ed., 2014, 53, 1809–1813 CrossRef CAS PubMed;
(e) D.-T. D. Tang, K. D. Collins and F. Glorius, J. Am. Chem. Soc., 2013, 135, 7450–7453 CrossRef CAS PubMed , and references cited therein. An early report: ;
(f) N. Nakamura, Y. Tajima and K. Sakai, Heterocycles, 1982, 19, 235–245 CrossRef.
- For recent examples, see:
(a) D. Rasina, A. Kahler-Quesada, S. Ziarelli, S. Warratz, H. Cao, S. Santoro, L. Ackermann and L. Vaccaro, Green Chem., 2016 10.1039/C6GC01393;
(b) G. G. Strappaveccia, E. Ismalaj, C. Petrucci, D. Lanari, A. Marrocchi, M. Drees, A. Facchetti and L. Vaccaro, Green Chem., 2015, 17, 365–372 RSC;
(c) G. Strappaveccia, L. Luciani, E. Bartollini, A. Marrocchi, F. Pizzo and L. Vaccaro, Green Chem., 2015, 17, 1071–1076 RSC , and cited references.
- For representative contributions, see:
(a) P. Pongrácz, L. Kollár and L. T. Mika, Green Chem., 2016, 18, 842–847 RSC;
(b) Z. Zhang, ChemSusChem, 2016, 9, 156–171 CrossRef CAS PubMed;
(c) L. Qi, Y. F. Mui, S. W. Lo, M. Y. Lui, G. R. Akien and I. T. Horváth, ACS Catal., 2014, 4, 1470–1477 CrossRef CAS;
(d) E. I. Gürbüz, J. M. R. Gallo, D. M. Alonso, S. G. Wettstein, W. Y. Lim and J. A. Dumesic, Angew. Chem., Int. Ed., 2013, 52, 1270–1274 CrossRef PubMed , see also: ;
(e) S. G. Wettstein, D. M. Alonso, Y. Chong and J. A. Dumesic, Energy Environ. Sci., 2012, 5, 8199–8203 RSC;
(f) Z.-Q. Duan and F. Hu, Green Chem., 2012, 14, 1581–1583 RSC;
(g) L. Qi and I. T. Horváth, ACS Catal., 2012, 2, 2247–2249 CrossRef CAS;
(h) I. T. Horváth, H. Mehdi, V. Fábos, L. Boda and L. T. Mika, Green Chem., 2008, 10, 238–242 RSC.
- I. T. Horváth, Green Chem., 2008, 10, 1024–1028 RSC.
- Y. Gu and F. Jérôme, Chem. Soc. Rev., 2013, 42, 9550–9570 RSC.
- L. Ackermann, Chem. Rev., 2011, 111, 1315–1345 CrossRef CAS PubMed.
- For detailed information, see the ESI†.
-
(a) R. H. Crabtree, Chem. Rev., 2012, 112, 1536–1554 CrossRef CAS PubMed;
(b) M. Pagliaro, V. Pandarus, R. Ciriminna, F. Béland and P. Demma Carà, ChemCatChem, 2012, 4, 432–445 CrossRef CAS.
-
(a) C. E. Garrett and K. Prasad, Adv. Synth. Catal., 2004, 346, 889–900 CrossRef CAS;
(b) Specification limits for residues of metal catalysts: http://www.ema.europa.eu/ema/.
- Under otherwise identical reaction conditions, a C–H arylation under air led to less effective catalysis.
-
(a) Syntheses via C–H Bond Functionalizations,
L. Ackermann, A. R. Kapdi, H. K. Potukuchi and S. I. Kozhushkov, in Handbook of Green Chemistry, ed. C.-J. Li, Wiley-VCH, Weinheim, 2012, pp. 259–305 Search PubMed;
(b) L. Vaccaro, D. Lanari, M. Assunta and G. Strappaveccia, Green Chem., 2014, 16, 3680–3704 RSC;
(c) J. Sherwood, M. D. Bruyn, A. Constantinou, L. Moity, C. R. McElroy, T. J. Farmer, T. Duncan, W. Raverty, A. J. Hunta and J. H. Clark, Chem. Commun., 2014, 50, 9650–9652 RSC.
Footnote |
† Electronic supplementary information (ESI) available: Experimental procedures, characterization data, and 1H and 13C NMR spectra for products. See DOI: 10.1039/c6cc03468c |
|
This journal is © The Royal Society of Chemistry 2016 |
Click here to see how this site uses Cookies. View our privacy policy here.