DOI:
10.1039/C5CC09446A
(Communication)
Chem. Commun., 2016,
52, 2027-2030
Sequential meta-C–H olefination of synthetically versatile benzyl silanes: effective synthesis of meta-olefinated toluene, benzaldehyde and benzyl alcohols†
Received
14th November 2015
, Accepted 2nd December 2015
First published on 2nd December 2015
Abstract
Tremendous progress has been made towards ortho-selective C–H functionalization in the last three decades. However, the activation of distal C–H bonds and their functionalization has remained fairly underdeveloped. Herein, we report sequential meta-C–H functionalization by performing selective mono-olefination and bis-olefination with late stage modification of the C–Si as well as Si–O bonds. Temporary silyl connection was found to be advantageous due to its easy installation, easy removal and wide synthetic diversification.
The transition metal catalyzed functionalization of unactivated C–H single bonds heralds the onset of a new era for the synthesis of complex molecular scaffolds, which being organic substances, display an undeniable presence of multiple C–H bonds.1 Recent advances in the field of the regioselective conversion of C–H bonds have widened the scope beyond the boundaries of classical catalytic cross-coupling reactions involving organohalides and the corresponding organometallic coupling partners. Moreover, a wise and prudential control of positional selectivity for C–H activation in a molecule containing multiple C–H bonds offers yet another outstanding challenge owing to its wide synthetic applications. An effective use of electronic bias2 and steric crowding3 for regioselectively functionalizing C–H bonds in a molecule suffers from its own limitations, because it prevents functionalization of a less reactive C–H bond.4 However, in an attempt to override such an intrinsic bias, different strategies have been undertaken, viz., σ-chelation-directed C–H activation, which has been used as a potent technique for achieving ortho-selectivity.5,6 Although ortho-selective C–H functionalization has been established in great depth over the last three decades, the selective functionalization of C–H bonds located further away from the coordinating functional group remains a noteworthy challenge,7–10 especially when their locations do not permit cyclometallation subjected to geometric strain. Similarly, meta-selective functionalization, which has been achieved to date primarily for disubstituted arenes using steric and electronic control,11,12 has emerged as a prolific area of scientific interest. Over last few years, an alternate template-based approach13,14 for position selective meta-C–H activation has been reported.15 During our recent studies into the template-assisted meta-C–H activation of benzyl sulfonic acid,14 we were intrigued by the possibility to introduce a linker, which could be easily attached, easily removed and be versatile towards different synthetic transformations.16 In pursuit of this objective,16 herein, we report selective mono-olefination and sequential bis-olefination at remote meta positions of benzyl silane using a nitrile-based template with post-synthetic diversification of silyl connection (Scheme 1).
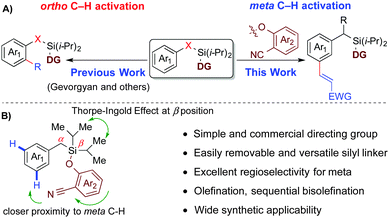 |
| Scheme 1 (A) Ortho and meta-C–H olefination of benzyl silane, (B) advantages of benzyl silyl ether. | |
Initially, this temporary silicon connection approach has been applied to restore superior regio- and stereo-selectivity by switching intermolecular reactions to intramolecular reactions.17 Later, Gevorgyan's group introduced these temporary silyl tethers for directed ortho-C–H activation followed by a high degree of diversification of the silyl moiety.18–20 We envisioned that the employment of a silyl-tethered directing group in place of a sulfonyl linker might be beneficial for meta-C–H activation of an appended arene in two ways. First, the silyl tether can be removed easily.21 Second, the silyl tether has advantage of wide applicability in different synthetic transformations.19,22,23 We hypothesized that the use of a simple 2-hydroxybenzonitrile ether of benzyl silanol may serve as an ideal choice for meta-C–H activation of the benzyl scaffold through linear coordination of the nitrile group (Schemes 1 and 2).
 |
| Scheme 2 Synthesis of directing template. | |
To test the hypothesis, benzyl diisopropylsilyl ether of 2-hydroxybenzonitrile was investigated with ethyl acrylate as a coupling partner. To our delight, the desired meta-olefinated product was observed in 72% yield with the meta selectivity of >20
:
1 (mono
:
di = 5
:
1).24 Upon extensive optimization and careful control of the electronic properties of cyanoarene derivatives, 2-hydroxy-5-methoxybenzonitrile was found to be the best directing group, producing 84% yield with a meta selectivity of >20
:
1 (Scheme 3, mono
:
di = 7
:
1).24
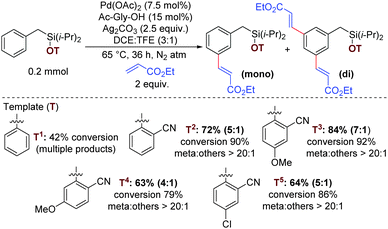 |
| Scheme 3 Optimization of directing group. | |
Initially, different benzyl derivatives were examined with methyl acrylate as the coupling partner in this optimized condition (Tables 1 and 2). The protocol was found to provide good to excellent yields, irrespective of the substituent position (ortho, meta, para or alpha). Diverse range of substituents, such as methyl, chloro, trifluoromethoxy, fluoro, bromo, trifluoromethyl, thiotrifluoromethyl, and methoxy are well tolerated under the reaction conditions (Table 1, entries 2a–2h). Interestingly, Heck coupling or protodehalogenation was not observed for the halogenated arenes (entries 2d and 2g). Electronic (entries 2c–2e) as well as steric bias (entry 2j–2k) can be successfully overridden by this template-assisted approach.
Table 1 Benzyl scope for meta-C–H olefination24,a
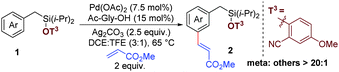
|
All the reactions were carried out using 1 (0.2 mmol), methyl acrylate (0.4 mmol) in DCE/TFE (1.2/0.4 mL) for 24 h at 65 °C.
2 mmol scale.
|
|
Table 2 Olefin scope for meta-C–H olefination24,a

|
All the reactions were carried out using 1 (0.2 mmol), olefin (0.4 mmol) in DCE/TFE (1.2/0.4 mL) for 24 h at 65 °C.
|
|
Subsequently, the scope for olefin coupling partners was contemplated by varying a wide range of electron deficient alkenes. Vinylsulphonates (Table 2, entries 3a and 3c) and alkyl vinyl ketone (entry 3f) readily reacted to give the desired products. Acrylamide (entry 3d) and vinylphosphonate (entry 3e) provided good yields with the in situ removal of the template. Different 1,2-disubstituted acrylates can be employed successfully to obtain thermodynamically favorable trans isomer (Table 2, entries 3g and 3h). Notably, cyclic tri-substituted olefins provided moderate yields with an expected allylic shift (entry 3i).25 High regioselectivity for meta isomer (>20
:
1) was obtained in all these cases, irrespective of the position and nature of the substituents and olefins.
During optimization and exploration of the substrate scope for meta-mono-olefination, we observed a high reactivity of this catalytic system because in some cases the diolefinated product was obtained in a minor amount. Encouraged by such observations, an attempt was made for sequential bis-olefination,26 because divinylbenzene derivatives are widely used as building blocks in materials research and synthetic chemistry.27 Accordingly, the mono-olefinated product was examined for further olefination at the remaining meta position (Table 3). We were pleased to confirm that sequential bis-olefination indeed can be achieved in good yields under modified reaction conditions. A diverse set of olefins, such as acrylates (Table 3, entries 4a and 4b), α,β-unsaturated ketone (entries 4d, 4i and 4k), acrylamide (entry 4e), and disubstituted internal olefins (entry 4f) were employed to provide moderate to good yields of bis-alkenylated products. Notably, sterically demanding ortho-substituted chlorobenzyl silyl ether also furnished the desired product (entry 4i). Even bulky tri-substituted cyclic olefin can be incorporated sequentially at both the meta positions of benzyl silyl ether (entry 4k).
Table 3 Scope for sequential meta-C–H bis-olefination24,a
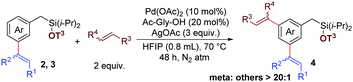
|
All the reactions were carried out using 2 or 3 (0.1 mmol), olefin (0.2 mmol) in HFIP (0.8 mL) for 48 h at 70 °C.
|
|
Next, to gain insights into the mode of action of this catalytic system, we performed an intermolecular competition experiment between 1a with two available meta positions and 1g with only one site available for olefination. The product ratio clearly indicated that the rates of reaction for both cases are similar (Scheme 4).
 |
| Scheme 4 Intermolecular competition experiment. | |
This newly developed methodology with an easily cleavable Si–O bond and a potentially modifiable C–Si bond could serve as a means to conduct a myriad of synthetic diversifications.20,28 Importantly, the silyl tether can be easily removed to provide olefinated toluene upon treatment of n-tetrabutyl ammonium fluoride (TBAF) in THF (Scheme 5, entry 5a). Meta-olefinated benzaldehyde can be obtained by modifying the same benzylic C–Si bond using nitrosobenzene as an oxidant (Scheme 5, entry 5b). Alternatively, the Fleming–Tamao oxidation of this C–Si bond can deliver meta-olefinated benzyl alcohol under mild conditions (Scheme 5, entry 5c).29 Mono-deutereted toluene derivatives can be obtained selectively by simply changing the solvent from THF to methanol-d4 at the time of the silyl deprotection (Scheme 5, entry 5d).
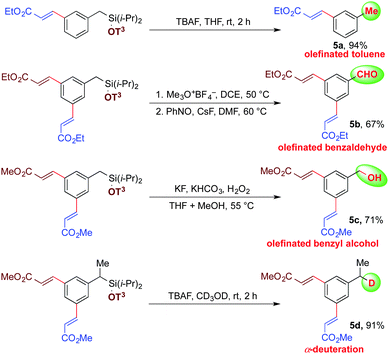 |
| Scheme 5 Post-synthetic modifications of meta-C–H olefinated benzyl silyl ether. | |
After meta-olefination, the cyanophenol template can be removed easily, providing meta-olefinated benzyl silanol 6a. Subsequent ortho-olefination23 can be achieved on meta-olefinated benzyl silanol to obtain 2,5-diolefinated benzyl silanol (Scheme 6, entry 6b). Notably, 3,5-hetero diolefinated benzyl silane has been synthesized successfully (Table 3). Therefore, position selective 2,5- and 3,5-hetero diolefinated isomers can be achieved by applying the required sequence judiciously.
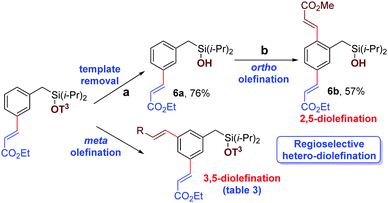 |
| Scheme 6 Sequential 2,5- and 3,5-hetero di-olefination of benzyl silane. [a] TsOH (10 mol%), ETOH/H2O = 1/1, 90 °C, 12 h. [b] Pd(OAc)2 (20 mol%), AgOAc (2 equiv.), KH2PO4 (2 equiv.), methyl acrylate (2 equiv.), CHCl3, 100 °C, 16 h. | |
In summary, the selective and efficient meta-C–H olefination of benzyl silane derivatives has been achieved with a simple and novel cyanophenol-based directing scaffold. Sequential olefination techniques were also developed for synthesizing valuable 2,5- and 3,5-hetero divinylbenzene derivatives exclusively. Meta-olefinated toluene, benzaldehyde and benzyl alcohol were also prepared easily upon removal of the silyl connection. Further synthetic applicability and detailed mechanistic investigations are currently underway.
This study was supported by SERB, India (EMR/2014/000164). Financial support received from UGC India (T. P.), IIT-B (R. W.) and CSIR India (S. A. and T. N.) are gratefully acknowledged.
Notes and references
-
(a) D. Y. K. Chen and S. W. Youn, Chem. – Eur. J., 2012, 18, 9452–9474 CrossRef CAS;
(b) J. Yamaguchi, A. D. Yamaguchi and K. Itami, Angew. Chem., Int. Ed., 2012, 51, 8960–9009 CrossRef CAS PubMed;
(c) P. Lu, Z. Gu and A. Zakarian, J. Am. Chem. Soc., 2013, 135, 14552–14555 CrossRef CAS.
- N. Kuhl, M. N. Hopkinson, J. Wencel-Delord and F. Glorius, Angew. Chem., Int. Ed., 2012, 51, 10236–10254 CrossRef CAS PubMed.
- For selected examples of C–H activation by steric bias, see:
(a) C. W. Liskey, X. Liao and J. F. Hartwig, J. Am. Chem. Soc., 2010, 132, 11389–11391 CrossRef CAS PubMed;
(b) Y. Saito, Y. Segawa and K. Itami, J. Am. Chem. Soc., 2015, 137, 5193–5198 CrossRef CAS PubMed.
- For C–H activation by other approaches, see:
(a) J. Wencel-Delord, T. Droge, F. Liu and F. Glorius, Chem. Soc. Rev., 2011, 40, 4740–4761 RSC;
(b) G. Menard and D. W. Stephan, Angew. Chem., Int. Ed., 2012, 51, 4409–4412 CrossRef CAS PubMed;
(c) G. Ménard, J. A. Hatnean, H. J. Cowley, A. J. Lough, J. M. Rawson and D. W. Stephan, J. Am. Chem. Soc., 2013, 135, 6446–6449 CrossRef PubMed;
(d) D. Winkelhaus and D. W. Stephan, Angew. Chem., Int. Ed., 2014, 53, 5414–5417 CrossRef CAS;
(e) M. Tobisu and N. Chatani, Science, 2014, 343, 850–851 CrossRef CAS;
(f) K. D. Collins, R. Honeker, S. Vasquez-Cespedes, D.-T. D. Tang and F. Glorius, Chem. Sci., 2015, 6, 1816–1824 RSC;
(g) S. Vásquez-Céspedes, A. Ferry, L. Candish and F. Glorius, Angew. Chem., Int. Ed., 2015, 54, 5772–5776 CrossRef PubMed.
- For selected reviews on ortho C–H activation, see:
(a)
C. G. Hartung and V. Snieckus, Modern Arene Chemistry, Wiley-VCH Verlag GmbH & Co, KGaA, 2004, pp. 330–367 Search PubMed;
(b) X. Chen, K. M. Engle, D.-H. Wang and J.-Q. Yu, Angew. Chem., Int. Ed., 2009, 48, 5094–5115 CrossRef CAS;
(c) O. Daugulis, H.-Q. Do and D. Shabashov, Acc. Chem. Res., 2009, 42, 1074–1086 CrossRef CAS PubMed;
(d) T. W. Lyons and M. S. Sanford, Chem. Rev., 2010, 110, 1147–1169 CrossRef CAS;
(e) Y. Aihara and N. Chatani, J. Am. Chem. Soc., 2013, 135, 5308–5311 CrossRef CAS;
(f) G. Rouquet and N. Chatani, Angew. Chem., Int. Ed., 2013, 52, 11726–11743 CrossRef CAS;
(g) L. Ackermann, Acc. Chem. Res., 2014, 47, 281–295 CrossRef CAS.
-
(a) K. M. Engle, T.-S. Mei, M. Wasa and J.-Q. Yu, Acc. Chem. Res., 2012, 45, 788–802 CrossRef CAS;
(b) Z. Chen, B. Wang, G. Zhu and J. Sun, Angew. Chem., Int. Ed., 2013, 52, 2027–2031 CrossRef CAS;
(c) W. Zhao, Z. Wang, B. Chu and J. Sun, Angew. Chem., Int. Ed., 2015, 54, 1910–1913 CrossRef CAS PubMed.
-
(a) X.-C. Wang, W. Gong, L.-Z. Fang, R.-Y. Zhu, S. Li, K. M. Engle and J.-Q. Yu, Nature, 2015, 519, 334–338 CrossRef CAS;
(b) Z. Dong, J. Wang and G. Dong, J. Am. Chem. Soc., 2015, 137, 5887–5890 CrossRef CAS.
-
(a) O. Saidi, J. Marafie, A. E. W. Ledger, P. M. Liu, M. F. Mahon, G. Kociok-Kohn, M. K. Whittlesey and C. G. Frost, J. Am. Chem. Soc., 2011, 133, 19298–19301 CrossRef CAS;
(b) N. Hofmann and L. Ackermann, J. Am. Chem. Soc., 2013, 135, 5877–5884 CrossRef CAS PubMed;
(c) C. J. Teskey, A. Y. W. Lui and M. F. Greaney, Angew. Chem., Int. Ed., 2015, 54, 11677–11680 CrossRef CAS.
-
(a) J. Cornella, M. Righi and I. Larrosa, Angew. Chem., Int. Ed., 2011, 50, 9429–9432 CrossRef CAS PubMed;
(b) F. Juliá-Hernández, M. Simonetti and I. Larrosa, Angew. Chem., Int. Ed., 2013, 52, 11458–11460 CrossRef;
(c) B. J. Groombridge, S. M. Goldup and I. Larrosa, Chem. Commun., 2015, 51, 3832–3834 RSC;
(d) J. Luo, S. Preciado and I. Larrosa, Chem. Commun., 2015, 51, 3127–3130 RSC.
- Y. Kuninobu, H. Ida, M. Nishi and M. Kanai, Nat. Chem., 2015, 7, 712–717 CrossRef CAS PubMed.
-
(a) J.-Y. Cho, M. K. Tse, D. Holmes, R. E. Maleczka and M. R. Smith, Science, 2002, 295, 305–308 CrossRef CAS;
(b) T. Ishiyama, J. Takagi, K. Ishida, N. Miyaura, N. R. Anastasi and J. F. Hartwig, J. Am. Chem. Soc., 2002, 124, 390–391 CrossRef CAS PubMed.
-
(a) Y.-H. Zhang, B.-F. Shi and J.-Q. Yu, J. Am. Chem. Soc., 2009, 131, 5072–5074 CrossRef CAS;
(b) R. J. Phipps and M. J. Gaunt, Science, 2009, 323, 1593–1597 CrossRef CAS PubMed.
-
(a) D. Leow, G. Li, T.-S. Mei and J.-Q. Yu, Nature, 2012, 486, 518–522 CrossRef CAS PubMed;
(b) H.-X. Dai, G. Li, X.-G. Zhang, A. F. Stepan and J.-Q. Yu, J. Am. Chem. Soc., 2013, 135, 7567–7571 CrossRef CAS PubMed;
(c) L. Wan, N. Dastbaravardeh, G. Li and J.-Q. Yu, J. Am. Chem. Soc., 2013, 135, 18056–18059 CrossRef CAS;
(d) R.-Y. Tang, G. Li and J.-Q. Yu, Nature, 2014, 507, 215–220 CrossRef CAS;
(e) G. Yang, P. Lindovska, D. Zhu, J. Kim, P. Wang, R.-Y. Tang, M. Movassaghi and J.-Q. Yu, J. Am. Chem. Soc., 2014, 136, 10807–10813 CrossRef CAS PubMed;
(f) Y. Deng and J.-Q. Yu, Angew. Chem., Int. Ed., 2015, 54, 888–891 CrossRef CAS;
(g) S. Lee, H. Lee and K. L. Tan, J. Am. Chem. Soc., 2013, 135, 18778–18781 CrossRef CAS PubMed;
(h) M. Bera, A. Modak, T. Patra, A. Maji and D. Maiti, Org. Lett., 2014, 16, 5760–5763 CrossRef CAS.
- M. Bera, A. Maji, S. K. Sahoo and D. Maiti, Angew. Chem., Int. Ed., 2015, 54, 8515–8519 CrossRef CAS.
-
(a) J. Schranck, A. Tlili and M. Beller, Angew. Chem., Int. Ed., 2014, 53, 9426–9428 CrossRef CAS PubMed;
(b) S. Li, H. Ji, L. Cai and G. Li, Chem. Sci., 2015, 6, 5595–5600 RSC.
- S. Bag, T. Patra, A. Modak, A. Deb, S. Maity, U. Dutta, A. Dey, R. Kancherla, A. Maji, A. Hazra, M. Bera and D. Maiti, J. Am. Chem. Soc., 2015, 137, 11888–11891 CrossRef CAS.
- G. Stork, H. S. Suh and G. Kim, J. Am. Chem. Soc., 1991, 113, 7054–7056 CrossRef CAS.
-
(a) C. Huang, B. Chattopadhyay and V. Gevorgyan, J. Am. Chem. Soc., 2011, 133, 12406–12409 CrossRef CAS;
(b) C. Huang, N. Ghavtadze, B. Chattopadhyay and V. Gevorgyan, J. Am. Chem. Soc., 2011, 133, 17630–17633 CrossRef CAS.
-
(a) A. V. Gulevich, F. S. Melkonyan, D. Sarkar and V. Gevorgyan, J. Am. Chem. Soc., 2012, 134, 5528–5531 CrossRef CAS;
(b) D. Sarkar, F. S. Melkonyan, A. V. Gulevich and V. Gevorgyan, Angew. Chem., Int. Ed., 2013, 52, 10800–10804 CrossRef CAS.
- C. Huang, N. Ghavtadze, B. Godoi and V. Gevorgyan, Chem. – Eur. J., 2012, 18, 9789–9792 CrossRef CAS.
- E. J. Corey and A. Venkateswarlu, J. Am. Chem. Soc., 1972, 94, 6190–6191 CrossRef CAS.
- N. Chernyak, A. S. Dudnik, C. Huang and V. Gevorgyan, J. Am. Chem. Soc., 2010, 132, 8270–8272 CrossRef CAS PubMed.
- C. Wang and H. Ge, Chem. – Eur. J., 2011, 17, 14371–14374 CrossRef CAS PubMed.
- See the Supporting Information for detailed description.
- G. T. Crisp, Chem. Soc. Rev., 1998, 27, 427–436 RSC.
-
(a) A. S. Carlstroem and T. Frejd, J. Org. Chem., 1991, 56, 1289–1293 CrossRef CAS;
(b) K. M. Engle, D.-H. Wang and J.-Q. Yu, Angew. Chem., Int. Ed., 2010, 49, 6169–6173 CrossRef CAS;
(c) A. Deb, S. Bag, R. Kancherla and D. Maiti, J. Am. Chem. Soc., 2014, 136, 13602–13605 CrossRef CAS.
-
(a) J. H. Burroughes, D. D. C. Bradley, A. R. Brown, R. N. Marks, K. Mackay, R. H. Friend, P. L. Burns and A. B. Holmes, Nature, 1990, 347, 539–541 CrossRef CAS;
(b) A. Kraft, A. C. Grimsdale and A. B. Holmes, Angew. Chem., Int. Ed., 1998, 37, 402–428 CrossRef;
(c) A. C. Grimsdale, K. Leok Chan, R. E. Martin, P. G. Jokisz and A. B. Holmes, Chem. Rev., 2009, 109, 897–1091 CrossRef CAS PubMed.
-
(a) A. Ricci, M. Fiorenza, M. A. Grifagni, G. Bartolini and G. Seconi, Tetrahedron Lett., 1982, 23, 5079–5082 CrossRef CAS;
(b) K. Itami, M. Mineno, T. Kamei and J.-i. Yoshida, Org. Lett., 2002, 4, 3635–3638 CrossRef CAS.
- K. Tamao, M. Kumada and K. Maeda, Tetrahedron Lett., 1984, 25, 321–324 CrossRef CAS.
Footnotes |
† Electronic supplementary information (ESI) available. See DOI: 10.1039/c5cc09446a |
‡ T. P. and R. W. contributed equally. |
|
This journal is © The Royal Society of Chemistry 2016 |