DOI:
10.1039/C4TX00079J
(Paper)
Toxicol. Res., 2015,
4, 152-159
An unexpected increase of toxicity of amino acid-containing ionic liquids†
Received
22nd July 2014
, Accepted 23rd September 2014
First published on 24th September 2014
Abstract
Functionalization of ionic liquids (ILs) with natural amino acids is usually considered as a convenient approach to decrease their toxicity and find new areas of chemical application as sustainable solvents, reagents or catalysts. In the present study, the cytotoxicity of several amino acid-containing ionic liquids (AAILs) with amino acid-based cations and anions was studied towards NIH/3T3 and CaCo-2 cell cultures and compared with the toxicity of conventional imidazolium-based ILs. The presence of an amino acid in the anion did not lead to a significant decrease in toxicity, whereas in the cation it unexpectedly increased the toxicity, as compared with conventional ILs. Exposure to 1-butyl-3-methylimidazolium chloride or 1-butyl-3-methylimidazolium glycinate induced apoptosis in NIH/3T3 cells. The present study gives new insights into biological effects of AAILs and shows that an amino acid residue may make ILs more biologically active. Special attention should be paid to the plausible synergetic effect of a combination of ILs with natural biologically active molecules. The results suggest possible medical application of AAILs rather than involvement as a green and sustainable tool to carry out chemical reactions.
Introduction
Ionic liquids (ILs) are unique non-volatile and non-flammable reaction media with ‘tunable’ physicochemical properties1 induced by their specific structure at molecular and supramolecular levels.2 ILs are ubiquitously used in various fields of modern chemistry and biology, including electrochemistry,3 organic synthesis,1,4 catalysis,5 extraction,6 biomass conversion,7 biological8 and medical research.8,9 Amino acid-functionalized ILs were developed as reagents and catalysts to mediate chemical transformations.10 Due to their valuable advantages, ILs in many cases have been considered as ‘green’ chemicals and possible substitutes for traditional organic solvents and reactants.1–11
However, after the initial burst of enthusiasm, the toxicity of many ILs has become obvious, and assessment of their ecological and biological impact has attracted much attention. For this purpose, various biological systems were employed, and the toxicity of ILs has been studied on diverse biological levels, from enzymes to vertebrates.8,12 It is now clear that ILs possess antibacterial, antifungal and herbicidal activities and may be toxic for both invertebrate and vertebrate animals and cytotoxic for different cell cultures. It has been proposed that toxicity of ILs depends on several structural factors: (1) the length of a cation alkyl side chain; (2) the presence of a functionalized cation side chain; and intrinsic properties of the nature of (3) anions and (4) cations.8 Therefore, it seems possible to ‘adjust’ biological properties of an IL by choosing appropriate cation and anion structures. According to numerous pieces of evidence, IL toxicity also depends on the nature of the biological object being affected, and molecular mechanisms of the toxic effects have not been fully elucidated yet. Thus, 1-butyl-3-methylimidazolium bis(trifluoromethylsulfonyl)imide demonstrated high toxicity towards the crustacean Daphnia magna13 and the springtail Folsomia candida,14 but was significantly less toxic for bacteria and fungi.15 For studying the cytotoxicity of ILs, cell cultures of various organisms – humans, rats, mice, Chinese hamsters, and others – have been used.8 Particularly, the toxicity of imidazolium-, guanidinium-, ammonium-, phosphonium-, pyridinium-, pyrrolidinium-, and choline-based ILs was investigated in CaCo-2 (colorectal adenocarcinoma) cells,16 and the toxicity of quinolinium-based ILs was investigated in NIH/3T3 (mouse fibroblasts) cells.17 It was supposed that long alkyl side chains of ILs may perturb the outer lipid membrane of the cell,18 and it was demonstrated that IL exposure induced DNA damage and apoptosis in PC12 (rat pheochromocytoma) and HeLa (human cervical cancer) cell lines.19
Therefore, the ‘green’ impact of ILs has been re-visited in the recent years, and the search for non-toxic, ecologically safe applications of ILs has been stimulated.1,8,9,11,12,20 Fukumoto and Ohno suggested that natural amino acids may become the basis of eco-friendly ILs21 and the field is rapidly developing now.10a,f,22 Ferlin and colleagues demonstrated that tetrabutylammonium prolinate-based ILs possessed low antimicrobial toxicity.10f However, no systematic attempts to discover the biological activity of amino acid-containing ILs (AAILs) have been made so far.
In the present study, we addressed cytotoxicity of several AAILs with amino acid-based cations and anions towards NIH/3T3 and CaCo-2 cell cultures and compared these data with toxicity of conventional imidazolium-based ILs. Both types of ionic liquid functionalization – incorporation of amino acids into cations and anions – were investigated towards their influence on cytotoxicity. Apoptosis in NIH/3T3 cells induced by the ILs and their biological activities are discussed in terms of cellular molecular processes.
Results and discussion
The structures of synthesized ILs ([BMIM][Gly], [BMIM][Ala], [BMIM][Val], [Gly-OMe][BF4], [Ala-OMe][BF4], [Val-OMe][BF4], [GlyO-EMIM][BF4]) and conventional imidazolium-based ILs ([EMIM][Cl], [BMIM][Cl], [HMIM][Cl], [EMIM][HSO4], [BMIM][HSO4], [BMIM][BF4], [BMIM][PF6], [BMIM][(L)-lac], [EMIMOH][BF4]) are shown in Fig. 1. To reveal the role of ionic cores and the influence of cation–anion interactions it was important to involve amino acids with non-functionalized side-chains (i.e. Gly, Ala and Val do not contain functional groups and reactive centres in the side-chain).
 |
| Fig. 1 Structures of ionic liquids used in the study (cation and anion structures, names and abbreviations). | |
Three amino acid salts – glycine hydrochloride (Gly·HCl), alanine hydrochloride (Ala·HCl), and valine hydrochloride (Val·HCl) – as well as pure amino acids – glycine, L-alanine and L-valine – were included in the test for comparison. Two cell lines, NIH/3T3 (mouse fibroblasts) and CaCo-2 (colorectal adenocarcinoma), were utilized in the study. The results of toxicity evaluation are shown in Table 1 (CaCo-2) and Table 2 (NIH/3T3). Smaller IC50 values correspond to more toxic compounds, with large variations in the measured values being observed.
Table 1 Cytotoxicity of studied ILs towards CaCo-2 cell line
|
IL |
M
w (g mol−1) |
24 h IC50a (mM) |
Standard error of the mean is shown.
|
1 |
[BMIM][Gly] |
213.28 |
16.31 ± 0.42 |
2 |
[BMIM][Ala] |
227.31 |
19.35 ± 1.03 |
3 |
[BMIM][Val] |
255.36 |
16.86 ± 0.58 |
4 |
[EMIM][Cl] |
146.62 |
32.10 ± 1.84 |
5 |
[BMIM][Cl] |
174.67 |
18.85 ± 1.27 |
6 |
[HMIM][Cl] |
202.72 |
6.21 ± 0.08 |
7 |
[EMIM][HSO4] |
208.24 |
17.64 ± 1.51 |
8 |
[BMIM][HSO4] |
236.29 |
13.70 ± 0.78 |
9 |
[BMIM][BF4] |
226.02 |
11.19 ± 0.63 |
10 |
[BMIM][PF6] |
284.18 |
11.50 ± 1.08 |
11 |
[BMIM][(L)-lac] |
228.29 |
19.16 ± 0.31 |
Table 2 Cytotoxicity of studied ILs and amino acids towards NIH/3T3 cell line
|
IL |
M
w (g mol−1) |
24 h IC50a (mM) |
Standard error of the mean is shown.
|
1 |
[BMIM][Gly] |
213.28 |
22.34 ± 0.93 |
2 |
[BMIM][Ala] |
227.31 |
30.23 ± 0.53 |
3 |
[BMIM][Val] |
255.36 |
25.18 ± 0.64 |
4 |
[EMIM][Cl] |
146.62 |
51.82 ± 0.45 |
5 |
[BMIM][Cl] |
174.67 |
25.93 ± 0.07 |
6 |
[HMIM][Cl] |
202.72 |
6.95 ± 0.14 |
7 |
[EMIM][HSO4] |
208.24 |
23.06 ± 0.33 |
8 |
[BMIM][HSO4] |
236.29 |
18.66 ± 0.20 |
9 |
[BMIM][BF4] |
226.02 |
11.30 ± 1.15 |
10 |
[BMIM][PF6] |
284.18 |
16.65 ± 0.11 |
11 |
[BMIM][(L)-lac] |
228.29 |
33.65 ± 0.53 |
12 |
[EMIMOH][BF4] |
213.97 |
25.45 ± 0.07 |
13 |
[Gly-OMe][BF4] |
176.91 |
9.94 ± 0.60 |
14 |
[Ala-OMe][BF4] |
190.93 |
9.56 ± 0.37 |
15 |
[Val-OMe][BF4] |
218.99 |
6.57 ± 0.10 |
16 |
[GlyO-EMIM][BF4] |
271.02 |
17.90 ± 0.58 |
17 |
Gly·HCl |
111.53 |
30.07 ± 0.25 |
18 |
Ala·HCl |
125.55 |
30.27 ± 0.47 |
19 |
Val·HCl |
153.61 |
30.07 ± 0.56 |
20 |
Gly |
75.07 |
>80 |
21 |
Ala |
89.09 |
>80 |
22 |
Val |
117.15 |
>80 |
First of all, when comparing IL effects in the two cell lines, we observed the differences in IC50 values. In almost all cases, the ILs showed a lower toxicity towards fibroblasts NIH/3T3, with the exception of [BMIM][BF4] and [HMIM][Cl] that demonstrated a similar toxicity in both CaCo-2 and NIH/3T3 cells (IC50 11.19 and 6.21 mM in CaCo-2 and 11.30 and 6.95 mM in NIH/3T3, respectively). Still, for some other ILs this difference was more prominent, e.g. IC50 32.10 and 51.82 mM for [EMIM][Cl] or 19.16 and 33.65 mM for [BMIM][(L)-lac] in CaCo-2 and NIH/3T3, respectively.
ILs with longer cation alkyl side chains proved to be more toxic (IC50 32.10, 18.85, and 6.21 mM or 51.82, 25.93, and 6.95 mM for [EMIM][Cl], [BMIM][Cl], and [HMIM][Cl] in CaCo-2 or NIH/3T3 cells, respectively). Similar results were obtained for imidazolium-based hydrogen sulfates (Tables 1 and 2, entries 7 and 8). Our data followed a similar trend reported by other groups that investigated the cytotoxicity of imidazolium-based ILs in CaCo-2 cells.16a,b
In our test system, IL toxicity was influenced by the anion moiety. Among the 1-butyl-3-methylimidazolium-based ILs tested, [BMIM][Ala], [BMIM][Cl], and [BMIM][(L)-lac] demonstrated the lowest toxicity in CaCo-2 cells (IC50 19.35, 18.85, and 19.16 mM, respectively), whereas [BMIM][Ala] and [BMIM][(L)-lac] were the least toxic in NIH/3T3 cells (30.23 and 33.65 mM, respectively). The highest toxicity was shown for [BMIM][BF4] and [BMIM][PF6] in CaCo-2 cells (IC50 11.19 and 11.50 mM, respectively) and for [BMIM][BF4] in NIH/3T3 cells (IC50 11.30 mM). Gly and Val as anions were less toxic than BF4, PF6, and HSO4, but were more toxic than L-lactate (Tables 1 and 2, entries 1, 3, 8–11).
Unexpected results were obtained for the AAILs containing amino acid cations, [Gly-OMe][BF4], [Ala-OMe][BF4], and [Val-OMe][BF4]. They showed highest toxicities among the ILs studied. In NIH/3T3 cells, IC50 of [Gly-OMe][BF4], [Ala-OMe][BF4], and [Val-OMe][BF4] were 9.94, 9.56, and 6.57 mM, respectively, and were comparable to and even higher than the toxicities of [HMIM][Cl] and [BMIM][BF4] (6.95 and 11.30 mM, respectively). In the case of [Val-OMe][BF4] and [BMIM][BF4], the difference was statistically significant (p < 0.05); in addition, IC50 of all three AAILs tested were significantly lower than that of [EMIMOH][BF4] (p < 0.05). We can possibly explain this by the following observations.
Amino acids enter the cells preferentially via special membrane transporter proteins.23 5′-Amino acid-linked prodrugs demonstrated higher membrane permeability than the parent drugs, and it was shown that the transport was realized via the PEPT1 transporter;24 in the past few years, transport of other amino acid-based prodrugs has also been investigated.25 As for ILs, they are thought to behave not as simple salt solutions consisting of separate anions and cations but as a network of anions and cations bound by hydrogen bonds and possessing a heterogeneous nanostructure.2 Recently we demonstrated that ILs could ‘distinguish’ slight changes in peptide structures, possibly due to the nanoscale organization of the former.26 Therefore, we suggest that amino acid cations may enhance the entrance of potentially harmful tetrafluoroborate anions into the cell, thus increasing the overall toxicity of such ILs.
The tetrafluoroborate anion demonstrated a relatively high toxicity being a part of [BMIM][BF4], which in NIH/3T3 was more toxic than all other BMIM-containing ILs tested in this study, implying the dominant effect of the anion. The formation of harmful fluoride ions from tetrafluoroborate anions was observed by Cho and colleagues when studying [BMIM][BF4] toxicity towards the alga Selenastrum capricornutum.27 Later Freire and colleagues studied the hydrolysis of imidazolium-based ILs with tetrafluoroborate and hexafluorophosphate anions and showed that tetrafluoroborate ions underwent hydrolysis even at room temperature.28
The observation that the presence of an amino acid might increase the biological activity of ILs was also confirmed by studying cytotoxicity of [GlyO-EMIM][BF4]. It was found to be moderate (IC50 17.9 mM), as compared with [BMIM][BF4], [Gly-OMe][BF4], [Ala-OMe][BF4], and [Val-OMe][BF4] (Table 2, entries 9, 13–15). However, toxicity of [GlyO-EMIM][BF4] seemed to exceed that of [EMIMOH][BF4] (IC50 25.45 mM), though the difference was not statistically significant. An overview of the cytotoxicity of the studied ILs is shown in Fig. 2.
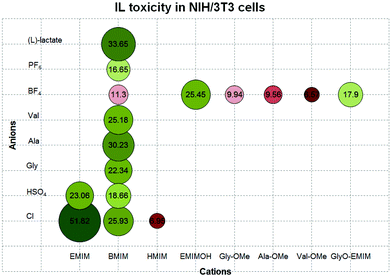 |
| Fig. 2 An overview of IL cytotoxicity in NIH/3T3 cells. Cations are shown in the X-axis, anions – in the Y-axis; the bubble size corresponds to the IC50 value (the bigger the safer); the five most toxic ILs are presented in red hues. | |
The amino acid salts Gly·HCl, Ala·HCl, and Val·HCl demonstrated low toxicities, in comparison with the other substances tested (IC50 30.07, 30.27, and 30.07 mM, respectively), but still were not nontoxic for NIH/3T3 cells. Amino acids Gly, Ala, and Val demonstrated no significant toxicity in the concentration range used (IC50 > 80 mM). According to the Toxicology Data Network,29 there are no available data on cytotoxicity of these amino acids; it was also shown that treatment with 100 mM L-valine did not affect the viability of HT-29 (human colorectal adenocarcinoma) cells.30
The evidence of increased biological activity of AAILs suggests their negative impact on the environment but also indicates their possible applications in biological and medical research. It was shown that 1-ethyl-3-methylimidazolium tetrafluoroborate induced apoptosis in HeLa cells,19a whereas 1-octyl-3-methylimidazolium bromide and chloride may cause apoptosis in PC12 cells.19b,c To assess the influence of ILs on NIH/3T3 cells, we used the annexin apoptosis assay. The results are shown in Fig. 3. After 24 h treatment, [BMIM][Cl] caused apoptosis in at least 45% of the cells (in the control, only 6% of cells underwent apoptosis). Similar results were obtained with [BMIM][Gly].
 |
| Fig. 3 [BMIM][Cl] induces apoptosis in NIH/3T3 cells. (A) Control (DMEM), (B) cells treated with 25 mM [BMIM][Cl] for 24 h. Apoptosis was assessed by flow cytometry analysis of cells stained with annexin (AnnexinV-FITC) and propidium iodide (PI). The field sections are interpreted as the following: upper left – necrotic cells (annexin V-negative, PI-positive); lower left – alive cells (annexin V-negative, PI-negative); upper right – cells undergoing late apoptosis/dead cells (annexin V-positive, PI-positive); lower right – cells undergoing early apoptosis (annexin V-positive, PI-negative). Axes show fluorescence intensity in arbitrary units (a.u.). | |
To visualize morphological changes occurring during the IL treatment, NIH/3T3 cells were stained with the Hoechst reagent. In [BMIM][Cl]-treated cells, nuclear fragmentation typical for apoptosis was clearly observed (Fig. 4).
 |
| Fig. 4 Nuclear morphological changes in NIH/3T3 cells treated with [BMIM][Cl]. Fluorescence microphotographs of Hoechst-stained nuclei of cells grown in the normal medium (A) or in the presence of 25 mM [BMIM][Cl] (B) for 24 h. Several representative nuclei are shown. Nuclear fragmentation is clearly seen in the IL-treated cells. | |
Targeting apoptosis and developing optimized targeted antitumor drugs underlie some of the leading concepts of antitumor therapies.31 The ability to cause apoptosis together with high biological activity and possibility of fine-tuning for cell targeting imply possible anticancer application of AAILs. Among other plausible fields of AAIL application are modulation of virulence of pathogens with the view of obtaining vaccinal strains;32 development of new systems of drug delivery for chemotherapy in cancer;9b,c,33 and modification of tumor cells for increasing the efficiency of the present approaches to the cancer immunotherapy.34 It has been established that the presence of ionic cores improves drug delivery and anticancer implications of various molecular and nano-sized systems.35
Conclusions
We assessed cytotoxicity of several AAILs and conventional ILs and demonstrated that the presence of an amino acid did not necessarily lead to lower toxicity. ILs with amino acid-based anions did not show biologically safe behaviour and their toxicity was comparable with conventional ILs, such as [BMIM][Cl] or [BMIM][(L)-lac]. Moreover, when combined with a toxic anion, amino acids could increase the ILs biological activity. Both conventional and amino acid-containing ILs induced apoptosis in NIH/3T3 cells. Therefore, AAILs should not be seen as ‘green’ compounds by initial design. The finding also suggests AAILs to be evaluated for possible medical applications. Functionalization of AAILs with drugs or biologically active substances may facilitate the entrance of the latter into the cell, which, in turn, could enhance the ability of the former to impose cell death.
Experimental
General procedures and reagents
1-Ethyl-3-methylimidazolium chloride ([EMIM][Cl]), 1-butyl-3-methylimidazolium chloride ([BMIM][Cl]), 1-ethyl-3-methylimidazolium hydrogen sulfate ([EMIM][HSO4]), and 1-butyl-3-methylimidazolium hydrogen sulfate ([BMIM][HSO4]) were obtained from ABCR, Germany; 1-hexyl-3-methylimidazolium chloride ([HMIM][Cl]), 1-butyl-3-methylimidazolium tetrafluoroborate ([BMIM][BF4]), 1-butyl-3-methylimidazolium hexafluorophosphate ([BMIM][PF6]), and 1-butyl-3-methylimidazolium L-lactate ([BMIM][(L)-lac]) were obtained from Acros, Belgium. Amino acids were obtained from Sigma-Aldrich. The structure and purity of the synthesized AAILs were confirmed using NMR spectroscopy and ESI mass-spectrometry measurements as described below. The corresponding spectral data are provided in the ESI.†
General synthetic procedure for amino acid ILs
1-Butyl-3-methylimidazolium glycinate ([BMIM][Gly]), 1-butyl-3-methylimidazolium alaninate ([BMIM][Ala]), and 1-butyl-3-methylimidazolium valinate ([BMIM][Val]) were prepared by the neutralization method, according to the earlier reported procedure.36 Aqueous solution of 1-butyl-3-methylimidazolium hydroxide ([BMIM][OH]) was prepared from [BMIM][HSO4] with the strontium hydroxide metathesis.37
Strontium hydroxide octahydrate (1.2 mmol) was dissolved in hot double-distilled water (95–100 °C, 3 mL) and added to 1-butyl-3-methylimidazolium hydrogen sulfate dissolved in hot double-distilled water (95–100 °C, 3 mL). The reaction mixture was stirred for 30 min at r.t. with an overhead stirrer and was left to cool (4 °C, 16 h). The white precipitate of strontium sulfate was discarded via vacuum filtration and was washed with water.
A slight excess of amino acid aqueous solution (1.2 mmol) was added dropwise to the previously obtained highly basic solution of [BMIM][OH]. The mixture was stirred at 0 °C for 17 h, followed by water evaporation under vacuum at 50 °C. The solvent mixture (acetonitrile–methanol, 9
:
1) was added to the residue and stirred vigorously. After 30 min of stirring, the mixture was filtered to remove the excess of amino acids and the filtrate was evaporated to give a crude product, which was dried from the solvent and water traces under vacuum at 80 °C (rotary evaporator followed by vacuum pump). Yield: 72–78%.
General synthetic procedure for 1-(2-hydroxyethyl)-3-methylimidazolium tetrafluoroborate
1-(2-Hydroxyethyl)-3-methylimidazolium tetrafluoroborate ([EMIMOH][BF4]) was synthesized according to the previously published procedure.38 A mixture of 1-methylimidazole (0.164 mL, 2 mmol), toluene (5 mL) and 2-bromoethanol (0.274 mL, 2.2 mmol) was flushed with argon and stirred for 24 h at 80 °C. After the reaction mixture was cooled down to r.t., the white solid formed rapidly. The solid was filtered off, washed with diethyl ether (3 × 5 mL) and MeCN (3 × 5 mL) and dried under vacuum at 60 °C. Then a mixture of 1-(2-hydroxyethyl)-3-methylimidazolium bromide (414 mg, 2 mmol), sodium tetrafluoroborate (440 mg, 4 mmol) and dry acetonitrile (5 mL) was heated to 82 °C for 48 h. Upon cooling to r.t., the white precipitate was filtered off and washed with acetonitrile (2 × 2 mL). The organic filtrate was concentrated under vacuum (rotary evaporator followed by vacuum pump) to obtain the product. Yield: 92%.
General synthetic procedure for amino acid hydrochloride salts
Glycine hydrochloride (Gly·HCl), alanine hydrochloride (Ala·HCl), and valine hydrochloride (Val·HCl) were prepared according to the earlier reported procedure.39 Yield: 94–95%.
General synthetic procedure for O-methyl amino acid tetrafluoroborates
O-Methylglycinate tetrafluoroborate ([Gly-OMe][BF4]), O-methylalaninate tetrafluoroborate ([Ala-OMe][BF4]), and O-methylvalinate tetrafluoroborate ([Val-OMe][BF4]) were synthesized according to the earlier reported procedure.39 Amino acid (1 mmol) was dissolved in methanol (2 mL), flushed with argon, and then placed into an ice bath. Thionyl chloride (3 mmol) was added dropwise to the amino acid solution; after completing the addition, the residual solution was stirred for additional 10 min, then the cooling bath was removed and stirring was continued at r.t. overnight. The solvent was evaporated under vacuum at 60 °C to obtain white crystals. The product was recrystallized from methanol–ether and dried at 70 °C under vacuum. Then the mixture of the obtained amino acid methyl ester hydrochloride (1 mmol), sodium tetrafluoroborate (2 mmol) and dry acetonitrile (5 mL) was flushed with argon and heated to 82 °C for 48 h. After cooling down to r.t., the white precipitate was filtered off and washed with 2 × 2 mL acetonitrile. The organic filtrate was concentrated under vacuum (rotary evaporator followed by vacuum pump) to obtain the product. Yield: 91–94%.
General synthetic procedure for amino acid-functionalized ILs
1-(2-(Glycyloxy)ethyl)-3-methylimidazolium tetrafluoroborate ([GlyO-EMIM][BF4]) was synthesized according to the earlier reported procedure.40 Dicyclohexylcarbodiimide (413 mg, 2 mmol) and dimethylaminopyridine (24 mg, 0.2 mmol) were added to 1-(2-hydroxyethyl)-3-methylimidazolium tetrafluoroborate (428 mg, 2 mmol) in dry acetonitrile (5 mL). The mixture was stirred on cooling for 10 min and then a Boc-protected amino acid (2.5 mmol), prepared according to the earlier reported procedure,41 dissolved in dry acetonitrile (5 mL) was added. The mixture was stirred vigorously for another 15 min and then for 24 h at r.t. After filtration the white precipitate was washed with acetonitrile (2 × 2 mL) and discarded. The combined organic phase was concentrated under vacuum at 60 °C. The ionic liquid functionalized with the Boc-amino acid in acetonitrile was treated with methanol (0.202 mL) and acetyl chloride (0.356 mL) for 24 h at r.t. Then the solvent was removed under reduced pressure. The solid residue was washed with 3 × 5 mL ethyl acetate and dried under vacuum (rotary evaporator followed by vacuum pump). Yield: 91%.
NMR measurements
All NMR measurements were performed using Bruker DRX 500 and Avance 600 spectrometers operating at 500.1 and 600.1 MHz for 1H and 125.8 and 150.9 MHz for 13C nuclei. The 1H NMR chemical shifts are reported relative to TMS as an internal standard. The 13C NMR chemical shifts are reported relative to the corresponding deuterated solvent signals. The spectra were processed using the Bruker Topspin 2.1 software package (Bruker).
ESI-MS measurements
ESI mass spectra were measured on a high-resolution Bruker maXis instrument equipped with an electrospray ionization (ESI) ion source. The measurements were performed in a positive ion mode (interface capillary voltage −4500 V) with a mass range m/z 50–3000 Da or in a negative ion mode (interface capillary voltage 2000 V) with a mass range m/z 50–3000 Da; external calibration of the mass spectrometer was performed using the Electrospray Tuning mix (Agilent). Syringe injection was used for solutions in MeCN–H2O (1
:
1) (flow rate 3.0 μL min−1). Nitrogen was applied as a nebulizer gas (0.4 bar) and a dry gas (4.0 L min−1), the interface temperature was set at 180 °C. Recorded spectra were processed using the Bruker DataAnalysis 4.0 software package (Bruker).
Cell cultures
CaCo-2 (colorectal adenocarcinoma, ATCC; purchased from the Institute of Cytology, Russian Academy of Sciences, St Petersburg, Russia) and NIH/3T3 (mouse fibroblasts, ATCC; purchased from the Institute of Cytology, Russian Academy of Sciences, St Petersburg, Russia) cells were cultured in plastic dishes (Corning Inc., USA) in a HeraCell 150 incubator (Thermo Electron Corp., USA) at 37 °C, 95% humidity, and 5% CO2. Cells were grown in the Dulbecco's modified Eagle's medium (DMEM; HyClone, USA) supplemented with 15% or 10% fetal bovine serum (FBS; HyClone, USA) (for CaCo-2 or NIH/3T3, respectively), 2 mM L-glutamine (HyClone, USA), 100 un. mL−1 penicillin (OAO Sintez, Russia), and 100 μg mL−1 streptomycin (OAO Biokhimik, Russia).
Cytotoxicity assay
The MTT assay was used for evaluation of IL cytotoxic action. This assay is based on measuring the activity of cellular enzymes that reduce the tetrazolium dyes MTT, XTT, MTS, or WST.42 Before the test, cells were trypsinized and passaged into 96-well flat-bottomed plates, 10
000 (5000 for CaCo-2) cells per well. The utmost wells were filled with 200 μL phosphate-buffered saline (PBS) (HyClone, USA). Cells were cultivated until reaching 70% monolayer (40 h for CaCo-2, 20 h for NIH/3T3) and then were incubated with ILs (in the concentration range from 200 mM to 390 μM, ten points in total, with the exception of [BMIM][PF6] for which the range from 55 mM to 110 μM was used due to its low water solubility). All test points were measured in 3–6 replicas. The same IL concentrations were applied to an empty plate to allow an adjustment for the IL influence on light absorption. 1% Triton (HyClone, USA) in DMEM was used as a positive control, and pure DMEM as a negative one. Cells were incubated with ILs for 24 h; then 20 μL of the MTS (3-(4,5-dimethylthiazol-2-yl)-5-(3-carboxymethoxyphenyl)-2-(4-sulfophenyl)-2H-tetrazolium) reagent (CellTiter 96® AQueous One Solution Cell Proliferation Assay, Promega, USA) were added into each cell, and the plates were incubated for 4 h. Optical density was measured at 492 and 650 nm using Original Multiskan EX (Lab Systems, USA); optical density values obtained at 650 nm were subtracted from the ones obtained at 492 nm to exclude background absorption. Statistical processing of the obtained data was carried out using Microsoft Excel 2010 (Microsoft) and Prism 5 (GraphPad); IC50 (half maximal inhibitory concentration) was calculated for each IL (data are expressed as the mean and standard error of the mean (SEM)).
Annexin apoptosis detection
Apoptosis was studied in NIH/3T3 cell culture. Cells were grown in DMEM, as described above. Before the test, the cells were seeded into six-well plates (Cornig, USA), 105 cells per milliliter, 3 mL per well. After 24 h the medium was removed and the fresh medium containing 25 mM [BMIM][Cl] or [BMIM][Gly] was added. The medium without IL was used as control. After 24 h the medium was removed and the cells were rinsed with PBS; the medium and PBS were stored in the corresponding vessels. The cells were treated with 0.05% trypsin (HyClone, USA), resuspended in the fresh medium, and were then placed into the vessels with the stored medium. The cells were centrifuged for 5 min at 1000 rcf, at r.t. The supernatant was removed; the pellet was resuspended in 1 mL PBS and centrifuged for 2 min at 1000 rcf, at r.t. Then the washing with PBS was repeated, the pellet was resuspended in 50 μL 1× buffer from the Annexin V-FITC Apoptosis Detection Kit (Sigma-Aldrich) and was placed into test tubes for flow cytometry (Beckman Coulter, Inc., USA). According to the protocol, 5 μL Annexin and 10 μL propidium iodide were added into each test tube. Fluorescence was measured using Cytomics FC 500 MPL (Beckman Coulter, Inc., USA); the results were interpreted in accordance with the manufacturer's recommendations and literature data.43
Hoechst staining
NIH/3T3 cells were grown in DMEM, as described above. Before the test, the cells were seeded into six-well plates (Cornig, USA), 105 cells per milliliter, 3 mL per well. Preliminarily, sterile cover glasses were placed on the bottom of the wells. After 24 h the medium was removed and the fresh medium containing 25 mM [BMIM][Cl] was added. The medium without IL was used as control. After 24 h the Hoechst reagent (bisBenzimide H 33342 trihydrochloride, Sigma-Aldrich) was added into the wells to the final concentration of 0.5 μg mL−1. The cells were incubated for 30 min in the CO2 incubator; then the medium was removed, the cells were washed with PBS thrice and were mounted into Mowiol (Calbiochem, Germany). The mounts were incubated in the thermostat for 1 h at 37 °C and then were captured using the Leica DMR microscope (lens 100×) with the Leica DC 350F camera.
Acknowledgements
The work was supported by the Russian Science Foundation (RSF Project 14-13-00448) and Russian Foundation for Basic Research (Projects 13-03-12231 and 14-03-31478). The authors thank Elena Lyssuk for valuable help in Annexin apoptosis assay and discussion of these results.
Notes and references
-
(a) N. V. Plechkova and K. R. Seddon, Chem. Soc. Rev., 2008, 37, 123 RSC;
(b) J. P. Hallett and T. Welton, Chem. Rev., 2011, 111, 3508 CrossRef CAS PubMed;
(c) J. D. Scholten, B. C. Leal and J. Dupont, ACS Catal., 2012, 2, 184 CrossRef CAS;
(d) Z. Lei, C. Dai and B. Chen, Chem. Rev., 2014, 114, 1289 CrossRef CAS PubMed;
(e) S. Zhang, J. Sun, X. Zhang, J. Xin, Q. Miao and J. Wang, Chem. Soc. Rev., 2014 10.1039/c3cs60409h.
-
(a)
Ionic liquids in chemical analysis, ed. M. Koel, CRC Press, Boca Raton, 2008 Search PubMed;
(b) R. Giernoth, Angew. Chem., Int. Ed., 2010, 49, 2834 CrossRef CAS PubMed;
(c) R. Giernoth, Angew. Chem., Int. Ed., 2010, 49, 5608 CrossRef CAS PubMed;
(d) V. P. Ananikov, Chem. Rev., 2011, 111, 418 CrossRef CAS PubMed;
(e) T. L. Greaves and C. J. Drummond, Chem. Soc. Rev., 2013, 42, 1096 RSC.
-
(a) H. Liu, Y. Liu and J. Li, Phys. Chem. Chem. Phys., 2010, 12, 1685 RSC;
(b) Y. Z. Su, Y. C. Fu, Y. M. Wei, J. W. Yan and B. W. Mao, ChemPhysChem, 2010, 11, 2764 CrossRef CAS PubMed.
- A. D. Sawant, D. G. Raut, N. B. Darvatkar and M. M. Salunkhe, Green Chem. Lett. Rev., 2011, 4, 41 CrossRef CAS.
-
(a) H. Olivier-Bourbigou, L. Magna and D. Morvan, Appl. Catal., A, 2010, 373, 1 CrossRef CAS PubMed;
(b) Q. H. Zhang, S. G. Zhang and Y. Q. Deng, Green Chem., 2011, 13, 2619 RSC.
-
(a) X. Sun, H. Luo and S. Dai, Chem. Rev., 2012, 112, 2100 CrossRef CAS PubMed;
(b) L. Vidal, M. L. Riekkola and A. Canals, Anal. Chim. Acta, 2012, 715, 19 CrossRef CAS PubMed.
-
(a) M. E. Zakrzewska, E. Bogel-Lukasik and R. Bogel-Lukasik, Chem. Rev., 2011, 111, 397 CrossRef CAS PubMed;
(b) E. A. Khokhlova, V. V. Kachala and V. P. Ananikov, ChemSusChem, 2012, 5, 783 CrossRef CAS PubMed;
(c) H. Wang, G. Gurau and R. D. Rogers, Chem. Soc. Rev., 2012, 41, 1519 RSC;
(d) A. Brandt, J. Gräsvik, J. P. Hallett and T. Welton, Green Chem., 2013, 15, 550 RSC;
(e) R. J. van Putten, J. C. van der Waal, E. de Jong, C. B. Rasrendra, H. J. Heeres and J. G. de Vries, Chem. Rev., 2013, 113, 1499 CrossRef CAS PubMed.
- K. S. Egorova and V. P. Ananikov, ChemSusChem, 2014, 7, 336 CrossRef CAS PubMed.
-
(a) S. V. Malhotra and V. Kumar, Bioorg. Med. Chem. Lett., 2010, 20, 581 CrossRef CAS PubMed;
(b) M. Moniruzzaman, Y. Tahara, M. Tamura, N. Kamiya and M. Goto, Chem. Commun., 2010, 46, 1452 RSC;
(c) L. Viau, C. Tourne-Peteilh, J. M. Devoisselle and A. Vioux, Chem. Commun., 2010, 46, 228 RSC;
(d) S. S. Kumar, M. Surianarayanan, R. Vijayaraghavan, A. B. Mandal and D. R. MacFarlane, Eur. J. Pharm. Sci., 2014, 51, 34 CrossRef CAS PubMed.
-
(a) P. Moriel, E. J. García-Suárez, M. Martínez, A. B. García, M. A. Montes-Morán, V. Calvino-Casilda and M. A. Bañares, Tetrahedron Lett., 2010, 51, 4877 CrossRef CAS PubMed;
(b) N. A. Larionova, A. S. Kucherenko, D. E. Siyutkin and S. G. Zlotin, Tetrahedron, 2011, 67, 1948 CrossRef CAS PubMed;
(c) P. Karthikeyan, S. S. Kumar, A. S. Arunrao, M. P. Narayan and P. R. Bhagat, Res. Chem. Intermed., 2012, 39, 1335 CrossRef;
(d) A. S. Kucherenko, D. E. Siyutkin, O. V. Maltsev, S. V. Kochetkov and S. G. Zlotin, Russ. Chem. Bull., 2012, 61, 1313 CrossRef CAS PubMed;
(e) X. Zhu, J. Liu, D. Zhang and C. Liu, Comput. Theor. Chem., 2012, 996, 21 CrossRef CAS PubMed;
(f) N. Ferlin, M. Courty, A. N. Van Nhien, S. Gatard, M. Pour, B. Quilty, M. Ghavre, A. Haiß, K. Kümmerer, N. Gathergood and S. Bouquillon, RSC Adv., 2013, 3, 26241 RSC;
(g) K. R. Roshan, T. Jose, D. Kim, K. A. Cherian and D. W. Park, Catal. Sci. Technol., 2014, 4, 963 RSC.
- D. Zhao, Y. Liao and Z. Zhang, Clean: Soil, Air, Water, 2007, 35, 42 CrossRef CAS.
-
(a) R. F. Frade and C. A. Afonso, Hum. Exp. Toxicol., 2010, 29, 1038 CrossRef CAS PubMed;
(b) M. Moniruzzaman, N. Kamiya and M. Goto, Org. Biomol. Chem., 2010, 8, 2887 RSC;
(c) T. P. Pham, C. W. Cho and Y. S. Yun, Water Res., 2010, 44, 352 CrossRef CAS PubMed.
- C. Pretti, C. Chiappe, I. Baldetti, S. Brunini, G. Monni and L. Intorre, Ecotoxicol. Environ. Saf., 2009, 72, 1170 CrossRef CAS PubMed.
- M. Matzke, S. Stolte, K. Thiele, T. Juffernholz, J. Arning, J. Ranke, U. Welz-Biermann and B. Jastorff, Green Chem., 2007, 9, 1198 RSC.
- J. Łuczak, C. Jungnickel, I. Łącka, S. Stolte and J. Hupka, Green Chem., 2010, 12, 593 RSC.
-
(a) R. F. M. Frade, A. Matias, L. C. Branco, C. A. M. Afonso and C. M. M. Duarte, Green Chem., 2007, 9, 873 RSC;
(b) A. García-Lorenzo, E. Tojo, J. Tojo, M. Teijeira, F. J. Rodríguez-Berrocal, M. P. González and V. S. Martínez-Zorzano, Green Chem., 2008, 10, 508 RSC;
(c) R. F. M. Frade, A. A. Rosatella, C. S. Marques, L. C. Branco, P. S. Kulkarni, N. M. M. Mateus, C. A. M. Afonso and C. M. M. Duarte, Green Chem., 2009, 11, 1660 RSC;
(d) G. V. S. M. Carrera, R. F. M. Frade, J. Aires-de-Sousa, C. A. M. Afonso and L. C. Branco, Tetrahedron, 2010, 66, 8785 CrossRef CAS PubMed;
(e) R. F. Frade, S. Simeonov, A. A. Rosatella, F. Siopa and C. A. Afonso, Chemosphere, 2013, 92, 100 CrossRef CAS PubMed;
(f) A. B. Pereiro, J. M. M. Araújo, S. Martinho, F. Alves, S. Nunes, A. Matias, C. M. M. Duarte, L. P. N. Rebelo and I. M. Marrucho, ACS Sustainable Chem. Eng., 2013, 1, 427 CrossRef CAS.
- M. McLaughlin, M. J. Earle, M. A. Gîlea, B. F. Gilmore, S. P. Gorman and K. R. Seddon, Green Chem., 2011, 13, 2794 RSC.
- N. Gal, D. Malferrari, S. Kolusheva, P. Galletti, E. Tagliavini and R. Jelinek, Biochim. Biophys. Acta, 2012, 1818, 2967 CrossRef CAS PubMed.
-
(a) X. Wang, C. A. Ohlin, Q. Lu, Z. Fei, J. Hu and P. J. Dyson, Green Chem., 2007, 9, 1191 RSC;
(b) X. Y. Li, C. Q. Jing, W. L. Lei, J. Li and J. J. Wang, Ecotoxicol. Environ. Saf., 2012, 83, 102 CrossRef CAS PubMed;
(c) X. Y. Li, C. Q. Jing, X. Y. Zang, S. Yang and J. J. Wang, Toxicol. in Vitro, 2012, 26, 1087 CrossRef CAS PubMed.
-
(a)
W. M. Nelson, in Ionic Liquids: Industrial Applications for Green Chemistry, ed. R. D. Rogers and K. R. Seddon, American Chemical Society, Washington, USA, 2002, vol. 818, pp. 30–41 Search PubMed;
(b) P. G. Jessop, Green Chem., 2011, 13, 1391 RSC;
(c) G. Stephens and P. Licence, Chim. Oggi, 2011, 29, 72 CAS;
(d) T. Welton, Green Chem., 2011, 13, 225 RSC;
(e) R. N. Das and K. Roy, Mol. Diversity, 2013, 17, 151 CrossRef CAS PubMed.
- K. Fukumoto and H. Ohno, Chem. Commun., 2006, 3081 RSC.
-
(a) N. Sharma, U. Kumar Sharma, R. Kumar, Richa and A. Kumar Sinha, RSC Adv., 2012, 2, 10648 RSC;
(b) R. Kumar, N. H. Andhare, A. Shard, Richa and A. K. Sinha, RSC Adv., 2014, 4, 19111 RSC;
(c) Z. Song, Y. Liang, M. Fan, F. Zhou and W. Liu, RSC Adv., 2014, 4, 19396 RSC.
-
(a) W. W. Souba and A. J. Pacitti, J. Parenter. Enteral Nutr., 1992, 16, 569 CAS;
(b) S. Broer, Physiol. Rev., 2008, 88, 249 CrossRef CAS PubMed;
(c)
Z. Qiu and M. Hu, in Oral Bioavailability: Basic Principles, Advanced Concepts, and Applications, ed. M. Hu and X. Li, John Wiley & Sons, Inc., Hoboken, NJ, USA, 2011, pp. 267–289 Search PubMed.
- H.-K. Han, R. L. A. de Vrueh, J. K. Rhie, K.-M. Y. Covitz, P. L. Smith, C.-P. Lee, D.-M. Oh, W. Sadee and G. L. Amidon, Pharm. Res., 1998, 15, 1154 CrossRef CAS.
-
(a) T.-Z. Su, E. Lunney, G. Campbell and D. L. Oxender, J. Neurochem., 2002, 64, 2125 CrossRef;
(b) T. Hatanaka, M. Haramura, Y. J. Fei, S. Miyauchi, C. C. Bridges, P. S. Ganapathy, S. B. Smith, V. Ganapathy and M. E. Ganapathy, J. Pharmacol. Exp. Ther., 2004, 308, 1138 CrossRef CAS PubMed.
- M. M. Seitkalieva, A. A. Grachev, K. S. Egorova and V. P. Ananikov, Tetrahedron, 2014, 70, 6975 CrossRef PubMed.
- C.-W. Cho, T. Phuong Thuy Pham, Y.-C. Jeon and Y.-S. Yun, Green Chem., 2008, 10, 67 RSC.
- M. G. Freire, C. M. Neves, I. M. Marrucho, J. A. Coutinho and A. M. Fernandes, J. Phys. Chem. A, 2010, 114, 3744 CrossRef CAS PubMed.
- Toxicology Data Network, http://toxnet.nlm.nih.gov/, (accessed June 2014).
- M. Selamnia, V. Robert, C. Mayeur, P.-H. Duée and F. Blachie, Biochim. Biophys. Acta, -Gen. Subj., 1998, 1379, 151 CrossRef CAS.
- S. Fulda, Semin. Cancer Biol., 2014 DOI:10.1016/j.semcancer.2014.05.002.
-
(a)
J. C. Williams and D. M. Waag, in Q Fever: The Biology of Coxiella burnetii (v. 2), ed. J. C. Williams and H. A. Thompson, CRC Press, Boca Raton, 1991, pp. 175–223 Search PubMed;
(b) S. A. Ermolaeva, I. F. Belyĭ and I. S. Tartakovskiĭ, Mol. Genet., Mikrobiol. Virusol., 2000, 1, 17 Search PubMed;
(c) J. Ko and G. A. Splitter, Clin. Microbiol. Rev., 2003, 16, 65 CrossRef CAS;
(d) J. Steel, BioDrugs, 2011, 25, 285 CrossRef CAS.
-
(a) N. V. Zhukov and S. A. Tjulandin, Biochemistry (Moscow), 2008, 73, 605 CrossRef CAS;
(b) C. Ghatak, V. G. Rao, S. Mandal, S. Ghosh and N. Sarkar, J. Phys. Chem. B, 2012, 116, 3369 CrossRef CAS PubMed;
(c) M. Kanapathipillai, A. Brock and D. E. Ingber, Adv. Drug Delivery Rev., 2014 DOI:10.1016/j.addr.2014.05.005.
-
(a) S. S. Larin, E. V. Korobko, O. S. Kustikova, O. R. Borodulina, N. T. Raikhlin, I. P. Brisgalov, G. P. Georgiev and S. L. Kiselev, J. Gene Med., 2004, 6, 798 CrossRef CAS PubMed;
(b) J. H. Finke, P. A. Rayman, J. S. Ko, J. M. Bradley, S. J. Gendler and P. A. Cohen, Cancer J., 2013, 19, 353 CrossRef CAS PubMed;
(c) M. H. Kershaw, J. A. Westwood and P. K. Darcy, Nat. Rev. Cancer, 2013, 13, 525 CrossRef CAS PubMed.
-
(a) S. Bontha, A. V. Kabanov and T. K. Bronich, J. Controlled Release, 2006, 114, 163 CrossRef CAS PubMed;
(b) J. O. Kim, G. Sahay, A. V. Kabanov and T. K. Bronich, Biomacromolecules, 2010, 11, 919 CrossRef CAS PubMed;
(c) M. Kamimura, J. O. Kim, A. V. Kabanov, T. K. Bronich and Y. Nagasaki, J. Controlled Release, 2012, 160, 486 CrossRef CAS PubMed;
(d) S. S. Desale, S. M. Cohen, Y. Zhao, A. V. Kabanov and T. K. Bronich, J. Controlled Release, 2013, 171, 339 CrossRef CAS PubMed;
(e) J. O. Kim, H. S. Oberoi, S. Desale, A. V. Kabanov and T. K. Bronich, J. Drug Targeting, 2013, 21, 981 CrossRef CAS PubMed;
(f) J. O. Kim, T. Ramasamy, C. S. Yong, N. V. Nukolov, T. K. Bronich and A. V. Kabanov, Mendeleev Commun., 2013, 23, 179 CrossRef CAS PubMed.
- K. Fukumoto, M. Yoshizawa and H. Ohno, J. Am. Chem. Soc., 2005, 127, 2398 CrossRef CAS PubMed.
- J. L. Ferguson, J. D. Holbrey, S. Ng, N. V. Plechkova, K. R. Seddon, A. A. Tomaszowska and D. F. Wassell, Pure Appl. Chem., 2012, 84, 723 CAS.
- L. C. Branco, J. N. Rosa, J. J. Moura Ramos and C. A. Afonso, Chem. – Eur. J., 2002, 8, 3671 CrossRef CAS.
- G. H. Tao, L. He, N. Sun and Y. Kou, Chem. Commun., 2005, 3562 RSC.
- M. Debdab, F. Mongin and J. Bazureau, Synthesis, 2006, 4046 CAS.
- G. L. Khatik, V. Kumar and V. A. Nair, Org. Lett., 2012, 14, 2442 CrossRef CAS PubMed.
- T. Mosmann, J. Immunol. Methods, 1983, 65, 55 CrossRef CAS.
-
(a) I. Vermes, C. Haanen, H. Steffensnakken and C. Reutelingsperger, J. Immunol. Methods, 1995, 184, 39 CrossRef CAS;
(b) M. van Engeland, F. C. Ramaekers, B. Schutte and C. P. Reutelingsperger, Cytometry, 1996, 24, 131 CrossRef CAS.
Footnote |
† Electronic supplementary information (ESI) available: NMR and ESI-MS spectral data are provided. See DOI: 10.1039/C4TX00079J |
|
This journal is © The Royal Society of Chemistry 2015 |
Click here to see how this site uses Cookies. View our privacy policy here.