DOI:
10.1039/C5SC02933C
(Edge Article)
Chem. Sci., 2015,
6, 7332-7335
Gold-catalyzed tandem reactions of amide–aldehyde–alkyne coupling and cyclization-synthesis of 2,4,5-trisubstituted oxazoles†
Received
9th August 2015
, Accepted 5th October 2015
First published on 6th October 2015
Abstract
We report the first cationic gold(I)-catalyzed one-pot reaction of amide, alkyne and aldehyde followed by cyclization, to successfully access highly substituted oxazoles derivatives in good yields. A single catalyst allows the occurring of this multi-step reaction atom- and step-economically, with water as the only theoretical side product.
Introduction
Oxazoles are important heterocyclic motifs present in a wide range of bioactive molecules,1 natural products,2 advanced materials,3 and ligand frameworks4 (Fig. 1). They exhibit highly variable properties and their structures are extremely diverse. As such, efficient synthetic methods accessing highly functionalized oxazoles are of great interest, yet remain challenging. Functionalization of pre-existing oxazole skeletons is one important strategy to access highly functionalized derivatives, but regioselectivity issues can limit such methods.5 More general synthetic pathways such as the Robinson–Gabriel6 and the van Leusen synthesis7 exploit a divergent strategy, consisting in the synthesis of acyclic oxazole precursors followed by a cyclisation.8 From an atom-economy perspective, such intramolecular cyclizations from acyclic precursors represent an attractive strategy for the preparation of substituted oxazoles. In the past decades, various transition metals have been reported to catalyze the cyclization of acetylenic precursors. Among these different methods, some use strong Brönsted acids or Lewis acid reagents which restrict the functional group tolerance.9 Thus, it is desirable to develop a simple approach to synthesize a broad variety of useful derivatives bearing diverse functionalities. Herein, we report a novel strategy for the multicomponent, one-pot synthesis of highly substituted oxazoles from simple amides, aldehydes and alkynes.
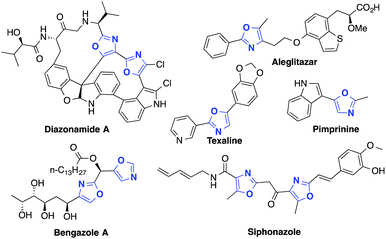 |
| Fig. 1 Examples of bioactive molecules and natural products containing oxazole moiety. | |
By furnishing complex products from simple building blocks in a minimum number of steps, multicomponent reactions represent efficient and rapid alternatives to traditional stepwise syntheses.10 One such reaction that has proven highly versatile and useful is the aldehyde–alkyne–amine coupling (A3-coupling) for the formation of propargylamines.11 Since its discovery,12 the multicomponent A3-coupling has been extensively developed by numerous authors, and shown great promise as a tool for the synthesis of complex molecules. In particular, its amenability to tandem transformations, especially cyclization, makes it an attractive technique for the synthesis of drug-like molecules. We envisioned that oxazoles might be accessed through such a tandem A3-coupling–cyclization, making use of amides instead of amines (Scheme 1). However, to the best of our knowledge, the formation of propargylamides via the coupling of amides, aldehydes and alkynes has never been reported before.13 Coinage transition-metal catalysts, such as gold, have shown excellent activity for the A3-coupling,14 and have been highly effective for the cyclization of acetylenic compounds.15 Thus, we envisioned that a judicious choice of gold catalyst might effectively catalyze both the A3-coupling and the tandem cyclization steps, providing access to highly functionalized oxazoles in a single pot.16
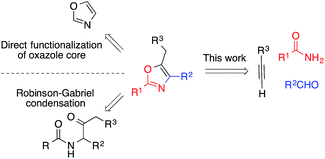 |
| Scheme 1 Designed strategy of one-pot gold-catalyzed A3/cyclization reaction. | |
Results and discussion
Inspired by our previous work on gold-catalyzed A3 reactions, we began our investigation using aryl amide 1a, alkyl aldehyde 2a and phenylacetylene 3a as substrates (Table 1).17
Table 1 Optimization of reaction conditionsa

|
Entry |
Catalyst (10 mol%) |
Additive (20 mol%) |
T (°C) |
Yield (%) |
5a
|
4a
|
Reaction conditions: benzamide (0.1 mmol), cyclohexanecarboxaldehyde (0.15 mmol), phenylacetylene (0.15 mmol), toluene (0.5 mL), under argon atmosphere.
4 Å molecular sieves were added.
50 mol% of additive was used. All reported yields were determined by 1H NMR spectroscopy of the crude reaction mixture using dibromomethane as internal standard. Yields in brackets are isolated.
|
1 |
Ph3PAuCl |
— |
100 |
5 |
0 |
2 |
Ph3PAuCl |
AgOTf |
100 |
45 |
30 |
3 |
Ph3PAuCl |
AgBF4 |
100 |
10 |
6 |
4 |
Ph3PAuCl |
AgSbF6 |
100 |
10 |
7 |
5 |
Ph3PAuCl |
AgNTf2 |
100 |
7 |
5 |
6b |
Ph3PAuCl |
AgOTf |
100 |
0 |
0 |
7c |
Ph3PAuCl |
AgOTf |
100 |
30 |
8 |
8 |
Ph3PAuCl |
AgOTf |
130 |
5 |
45 |
9 |
Ph3PAuCl |
AgOTf |
150 |
0 |
99 (95) |
10 |
— |
— |
150 |
0 |
0 |
11 |
— |
AgOTf |
150 |
10 |
0 |
12 |
— |
AgCl |
150 |
0 |
0 |
While triphenylphosphinegold(I) chloride on its own did not generate any desired product, the addition of silver(I) triflate furnished product 4a in 30% yield (entry 2). The counter-anion of silver salt dramatically influenced the yields of the reaction, with triflate giving the best result (entries 2–5). When Ph3PAuCl/AgOTf was used in toluene at 100 °C, a significant amount of 3-acylamidoketone 5a was detected, as well as its regioisomer 5b in a trace amount (<10%, see Scheme 2). To investigate the influence of water on the formation of this side-product, 4 Å molecular sieves were added (entry 6), which resulted in the complete inhibition of the desired reaction possibly due to gold poisoning from the molecular sieves.18 While it has been reported that a suitable acid activator (i.e. AgOTf) prevents the degradation of the gold catalyst,18 the addition of 50 mol% AgOTf was not beneficial to the reaction (entry 7). Although only a slight improvement of the reaction yield was observed at 130 °C, increasing the reaction temperature to 150 °C drastically accelerated the reaction, leading to complete conversion and excellent yield of the desired product (entries 8 and 9). In the absence of metal catalyst or additive, no desired product was observed (entry 10–12). The silver chloride formed during the catalyst preformation likewise showed no activity in the reaction (entry 12).
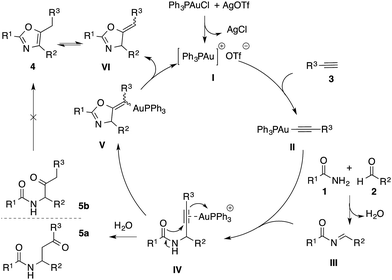 |
| Scheme 2 Proposed mechanism for the A3 coupling/cyclo-addition reaction. | |
With the optimized conditions in hand, we investigated the reaction scope (Table 2). We were pleased to find that both aliphatic and aromatic aldehydes delivered the corresponding oxazoles in moderate to excellent yields.
Table 2 Amide, aldehyde, alkyne coupling – formation of 2,4,5 tri-substituted oxazolesa
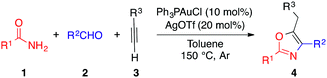
|
Conditions: amides (0.2 mmol), aldehydes (0.3 mmol), alkynes (0.3 mmol), Ph3PAuCl (10 mol%), AgOTf (20 mol%), 0.5 mL of toluene, 6 h, under argon. Isolated yields reported.
|
|
Cyclohexanecarboxaldehyde 2a reacted with the coupling partners to afford the substituted oxazole 4a in a significantly better yield (95% isolated yield) than acyclic aliphatic aldehyde 2b (4b). Aromatic aldehydes with various functional groups were well tolerated and the corresponding products were isolated in good to excellent yields (4c and 4d). While aromatic aldehydes with both electron-withdrawing groups (EWG) and -donating groups (EDG) were well tolerated under the reaction conditions, aldehydes bearing EWGs such as chloride and ester substituents generally provide the desired product (4g and 4i, respectively) in higher yields compared to the ones bearing EDGs such as –OMe (4e and 4f). Aromatic amides possessing different EWGs and EDGs were also evaluated, and resulted in good reaction yields (4j–n). It is noteworthy that even a boronic ester was tolerated under the reaction conditions, providing a handle for further functionalization via Suzuki coupling (4o). Impressively, our method can be further extended to the substrate bearing heterocyclic compound. The reaction of 4-chloronicotinamide afforded the oxazole product 4p in 62% yield. Fortunately, subjecting the substrate triisopropylsilyl acetylene 3q to the standard reaction conditions could successfully afford the corresponding oxazole heterocyclic compound 4q, albeit in a slightly lower yield. Besides, alkyl amide, such as acetamide, exposed to our reaction system produced 4r in a moderate yield.
Our proposed mechanism to rationalize this reaction is presented in Scheme 2. The abstraction of chloride from triphenylphosphinegold chloride complex by silver salt generates the active cationic gold species I, which reacts with phenylacetylene to form the gold acetylide II.19 Simultaneously, the condensation reaction between amide 1 and aldehyde 2 results in the formation of imide III. The subsequent addition of gold acetylide II to imide III affords propargylamide IV. Then the coordination of cationic gold species to alkyne can further assist either the intramolecular 5-exo-dig cyclization (towards the formation of cyclic organogold complex V), or the formation of hydrated side products 5a and 5b.17 It is noteworthy that in our experiments, these hydrated side-products were produced exclusively at lower temperature. To determine the fate of these side-products, control experiments with and without gold catalyst were conducted under our optimized reaction conditions. We observed that compounds 5a and 5b did not lead to the formation of the corresponding oxazoles. Finally, succeeding the formation of V, the oxazoline intermediate VI is obtained via protodeauration, which further tautomerizes into the desired tri-substituted oxazole product 4.
Conclusions
In summary, we have successfully developed a highly efficient one-pot coupling method for the direct synthesis of tri-substituted oxazoles via an unprecedented amide, aldehyde and alkyne coupling (A′A2). Using the tool of a single cationic gold(I) catalyst in one-pot to accomplish both the A′A2 and the cycloaddition reactions, provides a novel atom-economical and practical alternative to construct important heterocyclic compounds, with water as the only side product. We further envisioned that this tandem reaction could be extended towards many other synthetically useful motifs and the expansion of the scope of simple starting material is currently undergoing in our laboratory.
Acknowledgements
We are grateful to the Canada Research Chair (Tier 1) foundation (to C.-J.L.), NSERC, CFI, and FQRNT (CCVC) for their support of our research.
Notes and references
-
(a) N. Desroy, F. Moreau, S. Briet, G. L. Fralliec, S. Floquet, L. Durant, V. Vongsouthi, V. Gerusz, A. Denis and S. Escaich, Bioorg. Med. Chem., 2009, 17, 1276 CrossRef CAS PubMed;
(b) W.-L. Wang, D.-Y. Yao, M. Gu, M.-Z. Fan, J.-Y. Li, Y.-C. Xing and F.-J. Nan, Bioorg. Med. Chem. Lett., 2005, 15, 5284 CrossRef CAS PubMed.
-
(a) V. S. C. Yeh, Tetrahedron, 2004, 60, 11995 CrossRef CAS PubMed;
(b) P. Wipf, Chem. Rev., 1995, 95, 2115 CrossRef CAS;
(c) Z. Jin, Nat. Prod. Rep., 2006, 23, 464 RSC;
(d) Z. Jin, Nat. Prod. Rep., 2009, 26, 382 RSC;
(e) Z. Jin, Nat. Prod. Rep., 2011, 28, 1143 RSC.
- B. Iddon, Heterocycles, 1994, 37, 1321 CrossRef CAS.
-
(a) R. C. Jones, M. W. Chojnacka, J. W. Quail, M. G. Gardiner, A. Decken, B. F. Yates and R. A. Gossage, Dalton Trans., 2011, 40, 1594 RSC;
(b) A. Decken and R. A. Gossage, J. Inorg. Biochem., 2005, 99, 664 CrossRef CAS PubMed.
-
(a) S. Piguel, F. Besselièvre, S. Lebrequier and F. Mahuteau-Betzer, Synthesis, 2009, 20, 3511 CrossRef;
(b) P. Lassalas, F. Marsais and C. Hoarau, Synlett, 2013, 24, 2233 CrossRef CAS;
(c) N. A. Strotman, H. R. Chobanian, Y. Guo, J. He and J. E. Wilson, Org. Lett., 2010, 12, 3578 CrossRef CAS PubMed;
(d) X.-B. Shen, Y. Zhang, W.-X. Chen, Z.-K. Xiao, T.-T. Hu and L.-X. Shao, Org. Lett., 2014, 16, 1984 CrossRef CAS PubMed;
(e) L. Ackermann, C. Kornhaass and Y. Zhu, Org. Lett., 2012, 14, 1824 CrossRef CAS PubMed;
(f) J. Huang, J. Chan, Y. Chen, C. J. Borths, K. D. Baucom, R. D. Larsen and M. M. Faul, J. Am. Chem. Soc., 2010, 132, 3674 CrossRef CAS PubMed;
(g) C. Verrier, T. Martin, C. Hoarau and F. Marsais, J. Org. Chem., 2008, 73, 7383 CrossRef CAS PubMed.
- R. Robinson, J. Chem. Soc., Trans., 1909, 95, 2167 RSC.
- A. M. van Leusen, B. E. Hoogenboom and H. Siderius, Tetrahedron Lett., 1972, 13, 2369 CrossRef.
-
(a) C.-g. Shin, Y. Sato, H. Sugiyama, K. Nanjo and J. Yoshimura, Bull. Chem. Soc. Jpn., 1977, 50, 1788 CrossRef CAS;
(b) J. Das, J. A. Reid, D. R. Kronenthal, J. Singh, P. D. Pansegrau and R. H. Mueller, Tetrahedron Lett., 1992, 33, 7835 CrossRef CAS;
(c) S. K. Chattopadhyay, J. Kempson, A. McNeil, G. Pattenden, M. Reader, D. E. Rippon and D. Waite, J. Chem. Soc., Perkin Trans. 1, 2000, 1, 2415 RSC;
(d) F. Glorius and K. Schuh, Synthesis, 2007, 15, 2297 CrossRef;
(e) R. Martín, A. Cuenca and S. L. Buchwald, Org. Lett., 2007, 9, 5521 CrossRef PubMed;
(f) T. Lechel, M. Gerhard, D. Trawny, B. Brusilowskij, L. Schefzig, R. Zimmer, J. P. Rabe, D. Lentz, C. A. Schalley and H.-U. Reissig, Chem.–Eur. J., 2011, 17, 7480 CrossRef CAS PubMed;
(g) P. M. T. Ferreira, L. S. Monteiro and G. Pereira, Eur. J. Org. Chem., 2008, 27, 4676 CrossRef PubMed;
(h) P. M. T. Ferreira, E. M. S. Castanheira, L. S. Monteiro, G. Pereira and H. Vilaca, Tetrahedron, 2010, 66, 8672 CrossRef CAS PubMed;
(i) A. E. Wendlandt and S. S. Stahl, Org. Biomol. Chem., 2012, 10, 3866 RSC;
(j) Y. Zheng, X. Li, C. Ren, D. Zhang-Negrerie, Y. Du and K. Zhao, J. Org. Chem., 2012, 77, 10353 CrossRef CAS PubMed;
(k) N. C. Misra and H. Ila, J. Org. Chem., 2010, 75, 5195 CrossRef CAS PubMed;
(l) S. R. Bathula, M. P. Reddy, K. K. D. R. Viswanadham, P. Sathyanarayana and M. S. Reddy, Eur. J. Org. Chem., 2013, 21, 4552 CrossRef PubMed;
(m) N. Panda and R. Mothkuri, New J. Chem., 2014, 38, 5727 RSC.
- C. W. Cheung and S. L. Buchwald, J. Org. Chem., 2012, 77, 7526 CrossRef CAS PubMed.
- H. Xu, S. Ma, Y. Xu, L. Bian, T. Ding, X. Fang, W. Zhang and Y. Ren, J. Org. Chem., 2015, 80, 1789 CrossRef CAS PubMed.
-
(a) V. A. Peshkov, O. P. Pereshivko and E. V. van der Eycken, Chem. Soc. Rev., 2012, 41, 3790 RSC;
(b) C.-J. Li, C. Wei and Z. Li, Synlett, 2004, 9, 1472 CrossRef;
(c) C.-J. Li, Acc. Chem. Res., 2010, 43, 581 CrossRef CAS PubMed;
(d) L. Zani and C. Bolm, Chem. Commun., 2006, 4263 RSC;
(e) W.-J. Yoo, L. Zhao and C.-J. Li, Aldrichimica Acta, 2011, 44, 43 CAS.
-
(a) C.-J. Li and C. Wei, Chem. Commun., 2002, 3, 268 RSC;
(b) A. B. Dyatkin and R. A. Rivero, Tetrahedron Lett., 1998, 39, 3647 CrossRef CAS.
-
(a) H. Feng, D. S. Ermolat'ev, G. Song and E. V. van der Eycken, Adv. Synth. Catal., 2012, 354, 505 CrossRef CAS PubMed;
(b) X.-Y. Dou, Q. Shuai, L.-N. He and C.-J. Li, Adv. Synth. Catal., 2010, 352, 2437 CrossRef CAS PubMed;
(c) D. A. Black and B. A. Arndtsen, Org. Lett., 2004, 6, 1107 CrossRef CAS PubMed;
(d) C. Wei and C.-J. Li, Lett. Org. Chem., 2005, 2, 410 CrossRef CAS.
-
(a) C. Wei and C.-J. Li, J. Am. Chem. Soc., 2003, 125, 9584 CrossRef CAS PubMed;
(b) M. Cheng, Q. Zhang, X.-Y. Hu, B.-G. Li, J.-X. Ji and A. S. C. Chan, Adv. Synth. Catal., 2011, 353, 1274 CrossRef CAS PubMed;
(c) K.-F. Wong, J.-R. Deng, X.-Q. Wei, S.-P. Shao, D.-P. Xiang and M.-K. Wong, Org. Biomol. Chem., 2015, 13, 7408 RSC.
-
(a) Y.-M. Wang, A. D. Lackner and F. D. Toste, Acc. Chem. Res., 2013, 47, 889 CrossRef CAS PubMed;
(b) P. W. Davies, A. Cremonesi and L. Dumitrescu, Angew. Chem., Int. Ed., 2011, 50, 8931 CrossRef CAS PubMed;
(c) C. Ferrer and A. M. Echavarren, Angew. Chem., Int. Ed., 2006, 45, 1105 CrossRef CAS PubMed;
(d) A. S. K. Hashmi and G. J. Hutchings, Angew. Chem., Int. Ed., 2006, 45, 7896 CrossRef PubMed;
(e) A. S. K. Hashmi, A. M. Schuster and F. Rominger, Angew. Chem., Int. Ed., 2009, 48, 8247 CrossRef CAS PubMed;
(f) Y. Ma, S. Zhang, S. Yang, F. Song and J. You, Angew. Chem., Int. Ed., 2014, 53, 7870 CrossRef CAS PubMed;
(g) M. Hoffmann, S. Miaskiewicz, J.-M. Weibel, P. Pale and A. Blanc, Beilstein J. Org. Chem., 2013, 9, 1774 CrossRef PubMed;
(h) M. J. Campbell and F. D. Toste, Chem. Sci., 2011, 2, 1369 RSC;
(i) N. Patil, S. M. Inamdar and A. Konala, Chem. Commun., 2014, 50, 15124 RSC;
(j) R. Dorel and A. M. Echavarren, Chem. Rev., 2015, 115, 9028 CrossRef CAS PubMed;
(k) H. V. Adcock, T. Langer and P. W. Davies, Chem.–Eur. J., 2014, 20, 7262 CrossRef CAS PubMed;
(l) H. v. Wachenfeldt, P. Röse, F. Paulsen, N. Loganathan and D. Strand, Chem.–Eur. J., 2013, 19, 7982 CrossRef PubMed;
(m) T. Wang, L. Huang, S. Shi, M. Rudolph and A. S. K. Hashmi, Chem.–Eur. J., 2014, 20, 14868 CrossRef CAS PubMed;
(n) J. P. Weyrauch, A. S. K. Hashmi, A. Schuster, T. Hengst, S. Schetter, A. Littmann, M. Rudolph, M. Hamzic, J. Visus, F. Rominger, W. Frey and J. W. Bats, Chem.–Eur. J., 2010, 16, 956 CrossRef CAS PubMed;
(o) Z. Xin, S. Kramer, J. Overgaard and T. Skrydstrup, Chem.–Eur. J., 2014, 20, 7926 CrossRef CAS PubMed;
(p) A. S. K. Hashmi, M. Rudolph, S. Schymura, J. Visus and W. Frey, Eur. J. Org. Chem., 2006, 21, 4905 CrossRef PubMed;
(q) N. Ghosh, S. Nayak, B. Prabagar and A. K. Sahoo, J. Org. Chem., 2014, 79, 2453 CrossRef CAS PubMed;
(r) Y. Jeong, B.-I. Kim, J. K. Lee and J.-S. Ryu, J. Org. Chem., 2014, 79, 6444 CrossRef CAS PubMed;
(s) J.-M. Tang, T.-A. Liu and R.-S. Liu, J. Org. Chem., 2008, 73, 8479 CrossRef CAS PubMed;
(t) S. Zhu, H. Huang, Z. Zhang, T. Ma and H. Jiang, J. Org. Chem., 2014, 79, 6113 CrossRef CAS PubMed;
(u) W. He, C. Li and L. Zhang, J. Am. Chem. Soc., 2011, 133, 8482 CrossRef CAS PubMed;
(v) A. S. K. Hashmi, J. P. Weyrauch, W. Frey and J. W. Bats, Org. Lett., 2004, 6, 4391 CrossRef CAS PubMed;
(w) X. Yao and C.-J. Li, Org. Lett., 2006, 8, 1953 CrossRef CAS PubMed;
(x) Y. Zhang, J. P. Donahue and C.-J. Li, Org. Lett., 2007, 9, 627 CrossRef CAS PubMed;
(y) A. S. K. Hashmi, R. Salathe and W. G. Frey, Synlett, 2007, 11, 1763 CrossRef.
-
(a) N. Asao, S. Yudha, T. Nogami and Y. Yamamoto, Angew. Chem., Int. Ed., 2005, 44, 5526 CrossRef CAS PubMed;
(b) N. Chernyak and V. Gevorgyan, Angew. Chem., Int. Ed., 2010, 49, 2743 CrossRef CAS PubMed;
(c) H. Ohno, Y. Ohta, S. Oishi and N. Fujii, Angew. Chem., Int. Ed., 2007, 46, 2295 CrossRef CAS PubMed;
(d) K. Cao, F.-M. Zhang, Y.-Q. Tu, X.-T. Zhuo and C.-A. Fan, Chem.–Eur. J., 2009, 15, 6332 CrossRef CAS PubMed;
(e) Y. Zhang, P. Li and L. Wang, J. Heterocycl. Chem., 2011, 48, 153 CrossRef CAS PubMed;
(f) M. Saifuddin, P. K. Agarwal and B. Kundu, J. Org. Chem., 2011, 76, 10122 CrossRef CAS PubMed;
(g) Q. Zhang, M. Cheng, X. Hu, B.-G. Li and J.-X. Ji, J. Am. Chem. Soc., 2010, 132, 7256 CrossRef CAS PubMed;
(h) F. P. Xiao, Y. L. Chen, Y. Liu and J. B. Wang, Tetrahedron, 2008, 64, 2755 CrossRef CAS PubMed;
(i) H. Z. Syeda Huma, R. Halder, S. Singh Kalra, J. Das and J. Iqbal, Tetrahedron Lett., 2002, 43, 6485 CrossRef CAS.
- For additional screening results, see ESI Tables S1–S4. For control experiments, see Schemes S1–S2.†.
- M. Kumar, G. B. Hammond and B. Xu, Org. Lett., 2014, 16, 3452 CrossRef CAS PubMed . For influence of desiccants see Table S3 in ESI†.
-
(a) J. Bucher, T. Wurm, K. S. Nalivela, M. Rudolph, F. Rominger and A. S. K. Hashmi, Angew. Chem., Int. Ed., 2014, 53, 3854 CrossRef CAS PubMed;
(b) D. J. Gorin and F. D. Toste, Nature, 2007, 446, 395 CrossRef CAS PubMed.
Footnote |
† Electronic supplementary information (ESI) available: Experimental procedures and data for new compounds. See DOI: 10.1039/c5sc02933c |
|
This journal is © The Royal Society of Chemistry 2015 |
Click here to see how this site uses Cookies. View our privacy policy here.