DOI:
10.1039/C5SC02407B
(Edge Article)
Chem. Sci., 2015,
6, 7028-7033
Construction of highly functionalized carbazoles via condensation of an enolate to a nitro group†
Received
4th July 2015
, Accepted 2nd September 2015
First published on 16th September 2015
Abstract
This paper describes a novel synthesis of highly functionalized and diverse carbazoles via transition-metal-free and mild base-promoted condensations of readily available 2-nitrocinnamaldehyde or 2-nitrochalcones with various β-ketoesters or 1,3-diaryl-2-propanones. The method selectively forms four bonds by the intramolecular conjugate addition of an enolate to the enal or chalcone bearing an o-nitro group. This group then undergoes in situ N–O bond cleavage under non-reductive conditions in a one-pot procedure. This protocol allows for the introduction of various functional groups at all positions of the newly formed aromatic ring of the carbazole moiety. The utility of this methodology is further illustrated by the concise synthesis of naturally occurring hyellazole and chlorohyellazole.
Introduction
The carbazole framework is found in a wide range of bioactive natural products and pharmaceuticals (Fig. 1).1,2 These carbazole-containing molecules show antiviral,3 antimalarial,4 and antitumor activity.5 Some of them are currently being used as lead compounds for drug development.6 Carbazoles are also used as building blocks for the synthesis of functional materials, such as organic light-emitting diodes (OLED), because of their wide band gap, high luminescence efficiency, and allowing flexible modification of the parent skeleton.7,8
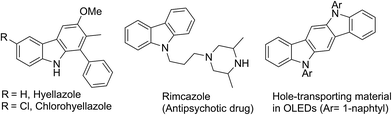 |
| Fig. 1 Selected naturally occurring, pharmaceutical, or electroactive carbazoles. | |
Owing to the importance and usefulness of these carbazole-based compounds, various approaches for their construction have been developed. The general and representative strategies can be classified into two main types depending on how the carbazole ring is constructed. The first strategy relies on the formation of a C–C or a C–N bond to construct the middle pyrrole ring starting from arene building blocks (methods A and B, Fig. 2).9–16 Also, the reaction of arynes with nitrosoarene and the nitrogenation of biphenyl halides have been reported.17 The second strategy involves the installation of a new aromatic ring onto functionalized indole derivatives via benzannulation (methods C and D, Fig. 2).18–23
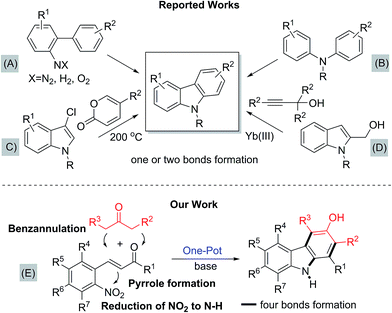 |
| Fig. 2 Diverse synthetic routes for carbazoles. | |
Despite their own merits, most, if not all, of these methods suffer from certain drawbacks, including low tolerance of functionality, limited substrate scope, not-easily accessible starting materials, the necessity of complex and expensive transition-metal catalysts, and harsh reaction conditions. In particular, many existing methods require either highly elaborated biaryls or biarylamines to construct the central pyrrole moiety or pre-functionalized indole derivatives for benzannulation. Therefore, more environmentally benign and modular multi-bond forming approaches accommodating structurally simple building blocks as the feedstock are highly sought-after to improve on these shortcomings. In relation to the synthesis of 3-hydroxy carbazoles, iron-mediated reactions have also been reported.24 A recently-reported rhodium-catalyzed tandem annulation uses a new approach, where the [5 + 1] cycloaddition of 3-hydroxy-1,4-enynes with CO generates three bonds and two rings.25 Yet, even for this transformation, various 3-hydroxy-1,4-enyne reagents must be prepared by a multi-step route.
In this regard, the new approach, depicted in E, accommodating a novel double annulation through the consecutive construction of a pyrrole and a benzene moiety reflects further innovation (Fig. 2). A unique feature of the current reaction compared with all other reported pyrrole formations or benzannulations is the formation of the carbazole nitrogen atom by electrophilic attack on a nitro group rather than the use of an amine nucleophile. Herein, we describe a unique tandem annulation followed by N–O bond cleavage without any external reductant for the synthesis of various functionalized 3-hydroxycarbazoles from readily available 2-nitrocinnamaldehyde or 2-nitrochalcone and β-ketoesters or 1,3-diaryl-2-propanone.
Results and discussion
First, the reaction of 2-nitrocinnamaldehyde (1a) and methyl 2-oxobutanoate (2a) was examined with several bases and solvents to optimize the reaction conditions (Table 1). The initial attempt with NaOMe (1 equiv.) in refluxing toluene for 12 h did not provide product 3a (Table 1, entry 1), but produced an intractable mixture. With triethylamine (1 equiv.), product 3a was also not formed (Table 1, entry 2), but with DBU (1 equiv.), 3a was produced in 10% yield (Table 1, entry 3). Encouraged by this result, other bases were screened. With K2CO3 (1 equiv.) for 6 h, the yield of 3a increased to 67% (Table 1, entry 4). The highest yield (81%) was achieved with 1.0 equivalent of Cs2CO3 in refluxing toluene for 4 h (Table 1, entry 5). Increasing the amount of Cs2CO3 to 1.5 equivalents (entry 6) or decreasing it to 0.1 equivalent (Table 1, entry 7) lowered the yield of 3a. Based on these results, this transformation was found to be sensitive towards the base strength used. For example, strong bases like NaOMe (1 equiv.) or DBU (1 equiv.) provided very little or no desired product, while weak bases provided better yields. Among the screened bases, Cs2CO3 was superior in terms of both reaction time and yield for this reaction, probably due to its mild and optimum base strength.26 In two other nonpolar solvents (benzene or dichloroethane), 3a was produced in 35 and 51% yield, respectively, whereas 3a was not obtained in a more polar solvent, such as methanol, DMSO, or water (Table 1, entries 8–12). The structure of 3a was established by spectroscopic analysis. The 1H NMR of 3a showed a characteristic singlet of the OH group at δ 11.12 ppm and another broad singlet for the NH proton at δ 8.17 ppm. The 13C NMR showed the expected characteristic ester carbonyl carbon at δ 171.6 ppm and an aromatic carbon containing OH at δ 157.7 ppm. The structural confirmation of 3a was further evidenced by X-ray crystallographic analysis of the related compound 7a (see ESI†).
Table 1 Optimization of the reaction conditions for the synthesis of carbazole 3aa
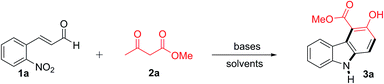
|
Entry |
Base |
Solvent |
Condition |
Yieldb (%) |
Reactions were conducted on a 1.0 mmol scale of 1a.
Isolated yield.
|
1 |
NaOMe (1 equiv.) |
Toluene |
Reflux, 12 h |
0 |
2 |
TEA (1 equiv.) |
Toluene |
Reflux, 12 h |
0 |
3 |
DBU (1 equiv.) |
Toluene |
Reflux, 12 h |
10 |
4 |
K2CO3 (1 equiv.) |
Toluene |
Reflux, 6 h |
67 |
5
|
Cs
2
CO
3
(1 equiv.)
|
Toluene
|
Reflux, 4 h
|
81
|
6 |
Cs2CO3 (1.5 equiv.) |
Toluene |
Reflux, 4 h |
78 |
7 |
Cs2CO3 (0.1 equiv.) |
Toluene |
Reflux, 12 h |
32 |
8 |
Cs2CO3 (1 equiv.) |
Benzene |
Reflux, 12 h |
35 |
9 |
Cs2CO3 (1 equiv.) |
DCE |
Reflux, 12 h |
51 |
10 |
Cs2CO3 (1 equiv.) |
MeOH |
Reflux, 12 h |
0 |
11 |
Cs2CO3 (1 equiv.) |
DMSO |
Reflux, 12 h |
0 |
12 |
Cs2CO3 (1 equiv.) |
Water |
Reflux, 12 h |
0 |
With the optimized conditions in hand, the generality of this reaction was explored by employing different β-ketoesters 2b–2i (Table 2). Reaction of 2-nitrocinnamaldehyde (1a) with several β-ketoesters such as ethyl 2-oxobutanoate (2b), allyl 3-oxobutanoate (2c) and benzyl 3-oxobutanoate (2d), afforded the desired products 3b–3d in 79, 82 and 77% yield, respectively. Moreover, the reactions of other β-ketoesters such as ethyl 3-oxopentanoate (2e), ethyl 3-oxohexanoate (2f), methyl 3-oxooctanoate (2g), methyl 3-oxododecanoate (2h), and methyl 3-oxo-4-phenylbutanoate (2i) provided the desired carbazoles 3e–3i in 73–78% yield.
Table 2 Formation of carbazole 3b–3i by the reaction of 2-nitrocinnamaldehyde 1a with various β-ketoesters 2b–2ia
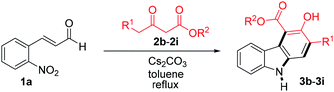
|
Reactions were performed on a 1.0 mmol scale according to the standard conditions described in Table 1.
|
|
The scope of the reaction was further extended by employing a series of 2-nitrochalcones and β-ketoesters (Table 3). When 2-nitrochalcone 4a was treated with allyl 3-oxobutanoate (2c), ethyl 3-oxopentanoate (2e) or 3-oxo-4-phenylbutanoate (2i) under optimized reaction conditions, the desired products 5a, 5b and 5c were formed in 75, 73 and 78% yield respectively. Furthermore, 2-nitrochalcones 4b–4c, bearing electron-donating or -withdrawing groups such as a methyl or bromo substituent on the 1-phenyl group, and β-ketoesters 2b, 2d and 2e also provided the desired products 5d–5f in 76, 75, and 70% yield, respectively. In addition, 2-nitrochalcones 4d–4f, having electron-donating or -withdrawing groups such as a methoxy, bromo and chloro substituent on the 3-phenyl group, produced the expected carbazoles 5g–5k in good yield (70–78%).
Table 3 Formation of carbazoles 5a–5k from various 2-nitrochalcones (4a–4f) and several β-ketoesters (2a–2e and 2i)a
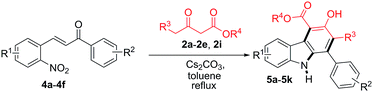
|
Reactions were performed on a 1.0 mmol scale according to the standard conditions described in Table 1.
|
|
The reactions between 2-nitrocinnamaldehyde (1a) or one of the 2-nitrochalcones (4a, 4c, 4d, 4e and 4f) and 1,3-diarylpropan-2-ones 6a and 6b were examined to further demonstrate the versatility of this carbazole formation (Table 4). The reaction of 1a with 6a or 6b in refluxing toluene for 4 h afforded the corresponding products 7a–7b in 81 and 80% yield, respectively. Similarly, the treatment of the nitrochalcones (4a, 4c, 4d, 4e, and 4f) with 6a or 6b provided the products 7c–7g in the range of 68–78% yield.
Table 4 Formation of carbazoles 7a–7g from 1a or the 2-nitrochalcones and 6a or 6ba
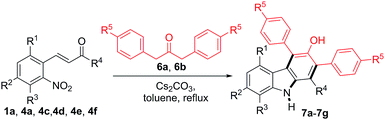
|
Reactions were performed on a 1.0 mmol scale according to the standard conditions described in Table 1.
|
|
Having confirmed the general applicability of the reaction by using 2-nitrocinnamaldehyde and the 2-nitrochalcones as starting materials, the possibility of using the 2-nitrochalcones bearing a heteroatom was examined, which would lead to the formation of carbazole derivatives with extended structural space. To our delight, the reactions of 8a or 8b with β-ketoesters 2b, 2d, and 2e provided the expected products 9a–9f in the range of 71–75% yield (Table 5).
Table 5 Formation of carbazoles 9a–9f from various 2-nitrochalcones (8a and 8b) and β-ketoesters (2b, 2d and 2e)a
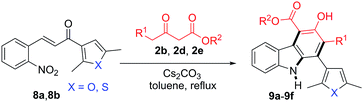
|
Reactions were performed on a 1.0 mmol scale according to the standard conditions described in Table 1.
|
|
We propose that the formation of the observed carbazole products may involve a mechanism shown in Scheme 1. In a basic medium, enolate 10 derived from 2a undergoes Michael addition onto 1a to give the new enolate intermediate 11, which subsequently reacts with the nitro group to form the bicyclic intermediate 12.27 The reorganization of the O–N–OH moiety in 12 to N–O–OH would generate 15via13 or 14. The base-induced elimination of the hydrogen peroxide from 15 would generate 16, which would then undergo sequential double tautomerization via17 or 18 to generate the observed product 3a.
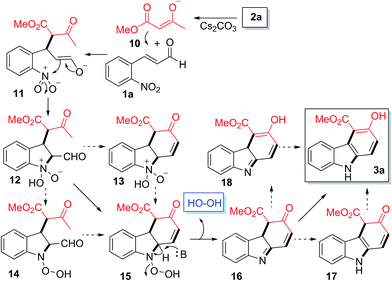 |
| Scheme 1 Proposed mechanism for the formation of 3a. | |
To obtain evidence for the formation of H2O2 during the reaction sequence, a control experiment was carried out with added aryl boronic acid (Scheme 2). To our delight, this reaction involving 1a, 2a and 2-naphthyl boronic acid 19 under the standard reaction conditions provided product 3a (61%) together with 2-naphthol 20 in 31% yield. The formation of 2-naphthol 20 implies the existence of in situ generated H2O2 in the reaction, although other mechanistic possibilities cannot be excluded.28
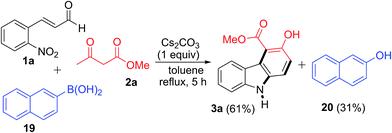 |
| Scheme 2 Control experiment to detect H2O2 in the reaction pathway. | |
Next, we broaden the carbazole structures to those that do not carry a carboethoxy group at the 4-position (Scheme 3). By carrying out the reaction at a higher temperature (145 °C) for a prolonged time using 2 equivalents of Cs2CO3 for decarboethoxylation, carbazoles 21a–21d were obtained in 68–75% yield.
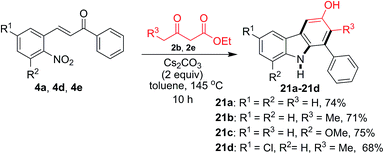 |
| Scheme 3 Formation of the decarboethoxylated carbazoles 21a–21d from various 2-nitrochalcones and β-ketoesters. | |
The utility of this new protocol was demonstrated by the conversion of 21b and 21d to biologically active natural products (Scheme 4). Upon treating 21b and 21d with iodomethane in refluxing acetone in the presence of K2CO3, hyellazole (22) and chlorohyellazole (23) were obtained in 94% and 92% yields, respectively. Our concise synthesis of hyellazole and chlorohyellazole was achieved in two steps from commercially available starting materials in 67% and 63% overall yields, respectively. This protocol has several advantages such as higher yields, lower cost, fewer steps, transition metal-free, and environmentally benignity.29,30 The identity of these two natural products was confirmed by the comparison of their spectroscopic data with those previously reported.29,30
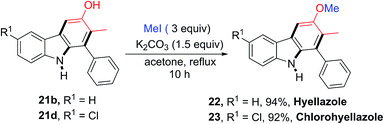 |
| Scheme 4 Synthesis of naturally occurring hyellazole (22) and chlorohyellazole (23). | |
Conclusions
A highly efficient, transition-metal-free, modular and operationally simple tandem annulation process was developed for the synthesis of diverse carbazole derivatives starting from readily available 2-nitrocinnamaldehydes or 2-nitrochalcones and β-ketoesters or 1,3-diaryl-2-propanones. This synthetic approach for the rapid construction of various functionalized carbazoles involves the intramolecular addition of an enolate to a nitro group and a unique in situ N–O bond cleavage under non-reductive conditions. As an application of this new synthetic methodology, a concise synthesis of naturally occurring bioactive hyellazole and chlorohyellazole has been realized in two steps.
Acknowledgements
This research was supported by the Nano Material Technology Development Program through the Korean National Research Foundation (NRF) funded by the Korean Ministry of Education, Science, and Technology (2012M3A7B4049675) and by the Korea government (MSIP) (NRF-2014R1A2A1A11052391).
Notes and references
-
(a) H.-J. Knölker and K. R. Reddy, Chem. Rev., 2002, 102, 4303 CrossRef PubMed;
(b) H.-J. Knölker, Curr. Org. Synth., 2004, 1, 309 CrossRef;
(c) H.-J. Knölker, Chem. Lett., 2009, 38, 8 CrossRef.
-
(a) C. Ito, M. Itoigawa, A. Sato, C. M. Hasan, M. A. Rashid, H. Tokuda, T. Mukainaka, H. Nishino and H. Furukawa, J. Nat. Prod., 2004, 67, 1488 CrossRef CAS PubMed;
(b) W. Maneerat, T. Ritthiwigrom, S. Cheenpracha, T. Promgool, K. Yossathera, S. Deachathai, W. Phakhodee and S. Laphookhieo, J. Nat. Prod., 2012, 75, 741 CrossRef CAS PubMed.
- A. W. Schmidt, K. R. Reddy and H.-J. Knölker, Chem. Rev., 2012, 112, 3193 CrossRef CAS PubMed.
- W.-S. Li, J. D. McChesney and F. S. El-Feraly, Phytochemistry, 1991, 30, 343 CrossRef CAS.
-
(a) T. Janosik, N. Wahlstrom and J. Bergman, Tetrahedron, 2008, 64, 9159 CrossRef CAS PubMed;
(b) W. R. Chao, D. Yean, K. Amin, C. Green and L. Jong, J. Med. Chem., 2007, 50, 3412 CrossRef CAS PubMed.
-
(a) A. A. Pieper, S. L. McKnight and J. M. Ready, Chem. Soc. Rev., 2014, 43, 6716 RSC;
(b) T. Takeuchi, S. Oishi, T. Watanabe, H. Ohno, J.-I. Sawada and K. Matsuno, J. Med. Chem., 2011, 54, 4839 CrossRef CAS PubMed.
-
(a) R. A. Reddy, U. Baumeister, C. Keith and C. Tschierske, J. Mater. Chem., 2007, 17, 62 RSC;
(b) J. Li and A. C. Grimsdale, Chem. Soc. Rev., 2010, 39, 2399 RSC.
-
(a) X. Liu, Y. Xu and D. Jiang, J. Am. Chem. Soc., 2012, 134, 8738 CrossRef CAS PubMed;
(b) H. Huang, Q. Fu, B. Pan, S. Zhaang, L. Wang, J. Chen, D. Ma and C. Yang, Org. Lett., 2012, 14, 4786 CrossRef CAS PubMed;
(c) D. Tsvelikhovsky and S. L. Buchwald, J. Am. Chem. Soc., 2011, 133, 14228 CrossRef CAS PubMed.
-
(a) L. Ackermann and A. Althammer, Angew. Chem., Int. Ed., 2007, 46, 1627 CrossRef CAS PubMed;
(b) R. B. Bedford and M. Betham, J. Org. Chem., 2006, 71, 9403 CrossRef CAS PubMed.
-
(a) A. L. Pumphrey, H. Dong and T. G. Driver, Angew. Chem., Int. Ed., 2012, 51, 5920 CrossRef CAS PubMed;
(b) B. J. Stokes, B. Jovanovic, H. Dong, K. J. Richert, R. D. Riell and T. G. Driver, J. Org. Chem., 2009, 74, 3225 CrossRef CAS PubMed.
-
(a) A. C. Hernandez-Perez and S. K. Collins, Angew. Chem., Int. Ed., 2013, 52, 12696 CrossRef CAS PubMed;
(b) L.-C. Campeau, M. Parisien, A. Jean and K. Fagnou, J. Am. Chem. Soc., 2006, 128, 581 CrossRef CAS PubMed;
(c) S. Trosien, P. Bottger and R. W. Siegfried, Org. Lett., 2014, 16, 402 CrossRef CAS PubMed;
(d) C. Wang, I. Piel and F. Glorius, J. Am. Chem. Soc., 2009, 131, 4194 CrossRef CAS PubMed;
(e) V. P. Kumar, K. K. Gruner, O. Kataeva and H.-J. Knölker, Angew.
Chem., Int. Ed., 2013, 52, 11073 CAS.
-
(a) K. Takamatsu, K. Hirano, T. Satoh and M. Miura, Org. Lett., 2014, 16, 2892 CrossRef CAS PubMed;
(b) A. P. Antonchick, R. Samanta, K. Kulikov and J. Lategahn, Angew. Chem., Int. Ed., 2011, 50, 8605 CrossRef CAS PubMed;
(c) J. A. Jordan-Hore, C. C. C. Johansson, M. Gulias, E. M. Beck and M. J. Gaunt, J. Am. Chem. Soc., 2008, 130, 16184 CrossRef CAS PubMed;
(d) S. H. Cho, S. H. Yoon and S. Chang, J. Am. Chem. Soc., 2011, 133, 5996 CrossRef CAS PubMed;
(e) W. C. P. Tsang, N. Zheng and S. L. Buchwald, J. Am. Chem. Soc., 2005, 127, 14560 CrossRef CAS PubMed.
-
(a) K. Nozaki, K. Takahashi, K. Nakano, T. Hiyama, H.-Z. Tang, M. Fujiki, S. Yamaguchi and K. Tamao, Angew. Chem., Int. Ed., 2003, 42, 2051 CrossRef CAS PubMed;
(b) A. Kuwahara, K. Nakano and K. Nozaki, J. Org. Chem., 2005, 70, 413 CrossRef CAS PubMed.
-
(a) J. I. G. Cadogan and M. Cameron-Wood, Proc. Chem. Soc. London, 1962, 361 CAS;
(b) F. Ragaini, S. Cenini, E. Gallo, A. Caselli and S. Fantauzzi, Curr. Org. Chem., 2006, 10, 1479 CrossRef CAS;
(c) J. T. Kuethe and K. G. Childers, Adv. Synth. Catal., 2008, 350, 1577 CrossRef CAS PubMed.
- H. Gao, Q.-L. Xu, M. Yousufuddin, D. H. Ess and L. Kurti, Angew. Chem., Int. Ed., 2014, 53, 2701 CrossRef CAS PubMed.
-
(a) H.-J. Knölker and N. O'Sullivan, Tetrahedron, 1994, 50, 10893 CrossRef;
(b) M. P. Krahl, A. Jäger, T. Krause and H.-J. Knölker, Org. Biomol. Chem., 2006, 4, 3215 RSC;
(c) R. Forke, A. Jäger and H.-J. Knölker, Org. Biomol. Chem., 2008, 6, 2481 RSC;
(d) R. Forke, M. P. Krahl, F. Däbritz, A. Jäger and H.-J. Knölker, Synlett, 2008, 1870 CAS;
(e) M. Schmidt and H.-J. Knölker, Synlett, 2009, 2421 CAS;
(f) R. Hesse, K. K. Gruner, O. Kataeva, A. W. Schmidt and H.-J. Knölker, Chem.–Eur. J., 2013, 19, 14098 CrossRef CAS PubMed.
- S. Chakrabarty, I. Chatterjee, L. Tebben and A. Studer, Angew. Chem., Int. Ed., 2013, 52, 2968 CrossRef CAS PubMed; Y. Oua and N. Jiao, Chem. Commun., 2013, 49, 3473 RSC.
-
(a) K. Ozaki, H. Zhang, H. Ito, A. Lei and K. Itami, Chem. Sci., 2013, 4, 3416 RSC;
(b) M. Abid, A. Spaeth and B. Torok, Adv. Synth. Catal., 2006, 348, 2191 CrossRef CAS PubMed.
- T. Guney, J. J. Lee and G. A. Kraus, Org. Lett., 2014, 16, 1124 CrossRef CAS PubMed.
- M. Yamashita, K. Hirano, T. Satoh and M. Miura, Org. Lett., 2009, 11, 2337 CrossRef CAS PubMed.
- S. Samala, A. K. Mandadapu, M. Saifuddin and B. Kundu, J. Org. Chem., 2013, 78, 6769 CrossRef CAS PubMed.
- S. Wang, Z. Chai, Y. Wei, X. Zhu, S. Zhou and S. Wang, Org. Lett., 2014, 16, 3592 CrossRef CAS PubMed.
- T. Tsuchimoto, H. Matsubayashi, M. Kaneko, Y. Nagase, T. Miyamura and E. Shirakawa, J. Am. Chem. Soc., 2008, 130, 15823 CrossRef CAS PubMed.
-
(a) H.-J. Knölker, M. Bauermeister and J.-B. Pannek, Chem. Ber., 1992, 125, 2783 CrossRef PubMed;
(b) H.-J. Knolker and M. Bauermeister, J. Chem. Soc., Chem. Commun., 1989, 1468 RSC;
(c) H.-J. Knölker and W. Fröhner, Tetrahedron Lett., 1997, 38, 1535 CrossRef;
(d) W. Fröhner, M. P. Krahl, K. R. Reddy and H.-J. Knölker, Heterocycles, 2004, 63, 2393 CrossRef;
(e) O. Kataeva, M. P. Krahl and H.-J. Knölker, Org. Biomol. Chem., 2005, 3, 3099 RSC;
(f) K. E. Knott, S. Auschill, A. Jäger and H.-J. Knölker, Chem. Commun., 2009, 1467 RSC;
(g) K. K. Gruner, T. Hopfmann, K. Matsumoto, A. Jäger, T. Katsukib and H.-J. Knölker, Org. Biomol. Chem., 2011, 9, 2057 RSC.
- X. Li, W. Song and W. Tang, J. Am. Chem. Soc., 2013, 135, 16797 CrossRef CAS PubMed.
-
(a) T. N. Poudel and Y. R. Lee, Org. Lett., 2015, 17, 2050 CrossRef CAS PubMed;
(b) T. N. Poudel and Y. R. Lee, Chem.–Eur. J., 2014, 12, 919 CAS;
(c) J. Qian, W. Yi, X. Huang, Y. Miao, J. Zhang, C. Cai and W. Zhang, Org. Lett., 2015, 17, 1090 CrossRef CAS PubMed.
-
(a) N. Moskalev and M. Makosza, Chem. Commun., 2001, 1248 RSC;
(b) M. Makosza, Chem.–Eur. J., 2014, 20, 5536 CrossRef CAS PubMed.
-
(a) G. K. S. Prakash, S. Chacko, C. Panja, T. E. Thomas, L. Gurung, G. Rasul, T. Mathew and G. A. Olah, Adv. Synth. Catal., 2009, 351, 1567 CrossRef CAS PubMed;
(b) J. Simon, S. Salzbrunn, G. K. S. Prakash, N. A. Petasis and G. A. Olah, J. Org. Chem., 2001, 66, 633 CrossRef CAS.
-
(a) S. B. Markad and N. P. Argade, Org. Lett., 2014, 16, 5470 CrossRef CAS PubMed;
(b) S. Kano, E. Sugino, S. Shibuya and S. Hibino, J. Org. Chem., 1981, 46, 3856 CrossRef CAS.
-
(a) S. Takano, Y. Suzuki and K. Ogasawara, Heterocycles, 1981, 16, 1479 CrossRef CAS;
(b) C. J. Moody and P. Shah, J. Chem. Soc., Perkin Trans. 1, 1989, 2463 RSC;
(c) R. L. Danheiser, R. G. Brisbois, J. J. Kowalczyk and R. F. Miller, J. Am. Chem. Soc., 1990, 112, 3039 CrossRef;
(d) T. Kawasaki, Y. Nonaka, M. Akahane, N. Maeda and M. Sakamoto, J. Chem. Soc., Perkin Trans. 1, 1993, 1777 RSC;
(e) E. M. Beccalli, A. Marchesin and T. Pilati, J. Chem. Soc., Perkin Trans. 1, 1994, 579 RSC;
(f) H.-J. Knölker, E. Baum and T. Hopfmann, Tetrahedron Lett., 1995, 36, 5339 Search PubMed;
(g) T. Choshi, T. Sada, H. Fujimoto, C. Nagayama, E. Sugino and S. Hibino, J. Org. Chem., 1997, 62, 2535 CrossRef CAS PubMed;
(h) H.-J. Knölker, E. Baum and T. Hopfmann, Tetrahedron, 1999, 55, 10391 CrossRef;
(i) E. Duval and G. D. Cuny, Tetrahedron Lett., 2004, 45, 5411 CrossRef CAS PubMed;
(j) H.-J. Knölker, W. Fröhner and R. Heinrich, Synlett, 2004, 2705 CrossRef.
Footnote |
† Electronic supplementary information (ESI) available: Experimental procedures, characterization data, 1H NMR and 13C NMR spectra for synthesized compounds. X-ray structure and data for 7a. CCDC 1046362. For ESI and crystallographic data in CIF or other electronic format see DOI: 10.1039/c5sc02407b |
|
This journal is © The Royal Society of Chemistry 2015 |
Click here to see how this site uses Cookies. View our privacy policy here.