DOI:
10.1039/C5SC02143J
(Edge Article)
Chem. Sci., 2015,
6, 5978-5983
Pyridine-enabled copper-promoted cross dehydrogenative coupling of C(sp2)–H and unactivated C(sp3)–H bonds†
Received
13th June 2015
, Accepted 20th July 2015
First published on 20th July 2015
Abstract
The pyridine-enabled cross dehydrogenative coupling of sp2 C–H bonds of polyfluoroarenes and unactivated sp3 C–H bonds of amides was achieved via a copper-promoted process with good functional group compatibility. This reaction showed great site-selectivity by favoring the sp2 C–H bonds ortho to two fluoro atoms of arenes and the sp3 C–H bonds of α-methyl groups over those of the α-methylene, β- or γ-methyl groups of the aliphatic amides. Mechanistic studies revealed that sp3 C–H bond cleavage is an irreversible but not the rate-determining step, and the sp2 C–H functionalization of arenes appears precedent to the sp3 C–H functionalization of amides in this process.
Introduction
Transition metal-promoted direct functionalization of unactivated C–H bonds is a highly valuable approach for the selective construction of C–C bonds, and considerable efforts have been devoted into this research area over the past couple of decades.1 Within this reaction category, ligand-assisted cross dehydrogenative coupling (CDC) is of current interest, and significant progress has been achieved in recent years.2 Compared with the conventional cross coupling reactions, this method enables the direct manipulation of aromatic and aliphatic C–H bonds by obviating the pre-installation of the functional groups. Moreover, the ligand acts as a directing group to ensure the high site-selectivity. In the process, a noble metal species such as palladium, rhodium, or ruthenium is often employed as a catalyst. From an economical point of view, avoidance of the use of the precious catalyst in the process would be highly desirable. Towards this effort, Miura and co-workers reported the first copper-promoted cross dehydrogenative coupling of 2-phenylpyridines and benzoxazoles in 2011 (Scheme 1a).3 It was then found that azine-N-oxides, benzamides, indoles, naphthylamines, and 2-pyridones were also effective substrates.4 Despite being a highly efficient method for the construction of C–C bonds, this process does not allow for the site-selective direct functionalization of unactivated sp3 bonds coupling with an arene bearing a directing group, thus restricting the product diversity.5,6 Inspired by the bidentate directing group-assisted unactivated sp3 C–H bond activation process developed by Daugulis' group,7 we envisaged that attachment of a bidentate directing group to an aliphatic acid may potentially overcome this drawback.8 With this design, we have examined and report here the copper-promoted cross dehydrogenative coupling of aliphatic amides9 and polyfluoroarenes10 (Scheme 1b), which provides an efficient access to alkyl-substituted perfluoroarenes, an important structural motif in pharmaceuticals and agrochemicals.11 It is worth mentioning that this is the first example of ligand-directed copper-promoted cross dehydrogenative coupling reaction by employing polyfluoroarenes as the coupling partners.
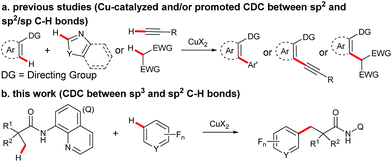 |
| Scheme 1 Copper-promoted cross dehydrogenative coupling (CDC) reactions. | |
Results and discussion
Our investigation commenced with cross dehydrogenative coupling of 2-ethyl-2-methyl-N-(quinolin-8-yl)pentanamide (1a) and pentafluorobenzene (2a) in 1,4-dioxane with stoichiometric amounts of Cu(OAc)2 under atmospheric oxygen (Table 1). After an extensive screening of the bases, pyridine has proven to be optimal, affording the desired product 3a in 22% yield, while all inorganic bases failed in the reaction (entries 1–7). Considering that pyridine could also act as a ligand in the process, and thus promote the reaction, a screening of nitrogen-containing potential bidentate ligands was further carried out. As shown in Table 1, several ligands such as TMEDA, 2,2′-bipyridine, and 1,10-phenanthroline could promote the process, but none of these molecules is as effective as pyridine (entries 8–10). Next, different copper sources were examined, and it was found that CuOAc was the only other species, but with less efficiency (entry 13). Then, the effects of an oxidant towards the reaction were examined, and it turned out that di-tert-butyl peroxide was optimal, providing 3a in 44% yield (entry 17). It was also noted that a higher yield could be obtained under atmospheric nitrogen (entry 18). Following the above investigation, we carried out an extensive screening of the solvents, and the reaction was significantly improved with the solvent mixture of DME and 1,4-dioxane (entry 22). Furthermore, an excellent yield was observed with increased amounts of pyridine (entry 24). It was also noted that the reaction yield was dramatically decreased with reduced amounts of the copper species (entry 25), presumably due to the competitive coordination of pyridine or tert-butanolate released from di-tert-butyl peroxide to copper. Moreover, it is clear that pyridine is required for this process since no apparent product formation occurred in the absence of pyridine (entry 26). As expected, a high site-selectivity was observed with a preference for the C–H bond of the α-methyl over that of the α-methylene, β- or γ-methyl group,9 which is believed to arise from the steric effect and preference for the formation of a five-membered ring intermediate over the six- or seven-membered ring intermediate in the cyclometalation step.
Table 1 Optimization of reaction conditionsa
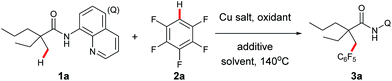
|
Entry |
Cu salt |
Oxidant |
Additive (eq.) |
Solvent |
Yield (%)b |
Reaction conditions: 1a (0.3 mmol), 2a (0.6 mmol), Cu salt (0.3 mmol), oxidant (0.75 mmol), additive, 1.0 mL of solvent, 140 °C, 16 h.
Yields and conversions are based on 1a, determined by 1H-NMR using dibromomethane as the internal standard. Isolated yield is in parenthesis.
Under N2 atmosphere.
Cu(OAc)2 (0.15 mmol). Q = 8-quinolinyl.
|
1 |
Cu(OAc)2 |
|
K2CO3 (2) |
1,4-Dioxane |
0 |
2 |
Cu(OAc)2 |
|
K2HPO4 (2) |
1,4-Dioxane |
0 |
3 |
Cu(OAc)2 |
|
PhCO2Na (2) |
1,4-Dioxane |
0 |
4 |
Cu(OAc)2 |
|
Et3N (2) |
1,4-Dioxane |
<5 |
5 |
Cu(OAc)2 |
|
Py (2) |
1,4-Dioxane |
22 |
6 |
Cu(OAc)2 |
|
2,6-Lutidine (2) |
1,4-Dioxane |
14 |
7 |
Cu(OAc)2 |
|
DMAP (2) |
1,4-Dioxane |
17 |
8 |
Cu(OAc)2 |
|
TMEDA (1) |
1,4-Dioxane |
6 |
9 |
Cu(OAc)2 |
|
2,2′-Dipyridyl (1) |
1,4-Dioxane |
16 |
10 |
Cu(OAc)2 |
|
1,10-Phen (1) |
1,4-Dioxane |
<5 |
11 |
CuCl2 |
|
Py (2) |
1,4-Dioxane |
0 |
12 |
CuBr2 |
|
Py (2) |
1,4-Dioxane |
0 |
13 |
CuOAc |
|
Py (2) |
1,4-Dioxane |
<5 |
14 |
CuBr |
|
Py (2) |
1,4-Dioxane |
0 |
15 |
Cu(OAc)2 |
Ag2O |
Py (2) |
1,4-Dioxane |
25 |
16 |
Cu(OAc)2 |
TBHP |
Py (2) |
1,4-Dioxane |
10 |
17 |
Cu(OAc)2 |
(tBuO)2 |
Py (2) |
1,4-Dioxane |
44 |
18c |
Cu(OAc)2 |
(tBuO)2 |
Py (2) |
1,4-Dioxane |
59 |
19c |
Cu(OAc)2 |
(tBuO)2 |
Py (2) |
DME |
46 |
20c |
Cu(OAc)2 |
(tBuO)2 |
Py (2) |
THF |
40 |
21c |
Cu(OAc)2 |
(tBuO)2 |
Py (2) |
Toluene |
15 |
22c |
Cu(OAc)2 |
(tBuO)2 |
Py (2) |
DME–1,4-dioxane (7 : 3) |
84 |
23c |
Cu(OAc)2 |
(tBuO)2 |
Py (1) |
DME–1,4-dioxane (7 : 3) |
50 |
24c |
Cu(OAc)2 |
(tBuO)2 |
Py (3) |
DME–1,4-dioxane (7 : 3) |
96 (92) |
25cd |
Cu(OAc)2 |
(tBuO)2 |
Py (3) |
DME–1,4-dioxane (7 : 3) |
63 |
26c |
Cu(OAc)2 |
(tBuO)2 |
— |
DME–1,4-dioxane (7 : 3) |
0 |
Under the optimized conditions, the scope with respect to fluoroarenes was examined. As shown in Table 2, tetrafluorobenzenes bearing a methoxyl, bromo, cyano, or trifluoromethyl group were compatible with the process (3b–e). Additionally, higher yields were observed with substrates substituted by an electron-withdrawing group, presumably due to the increased reactivity of the aromatic C–H bonds from the increased acidity of these bonds and/or increased electronegativity of a copper intermediate which facilitates the sp3 C–H bond activation.4 Furthermore, 1,2,3,5-tetrafluorobenzene, 1,2,4,5-tetrafluorobenzene, and trifluorobenzenes with an additional electron-withdrawing group were effective substrates (3f–i). Not surprisingly, low reactivity was observed with 1,3,5-trifluorobenzene, 1,2,4-trifluorobenzene, and 3,5-difluorobenzonitrile. Delightfully, successful couplings of these substrates were achieved at an elevated reaction temperature (3j–l). Interestingly, a high regioselectivity was also observed in these cases by favoring the C–H bonds between the two C–F bonds, the most acidic C–H bonds.10 Furthermore, fluoropyridines were also effective coupling partners for this process (3m and 3n). Unfortunately, other heteroaromatic substrates such as benzoxazoles, thiophenes, indoles etc. failed to provide any desired products under the current conditions.
Table 2 Scope of fluoroarenesab
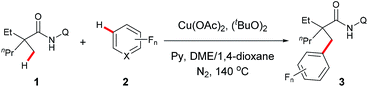
|
Reaction conditions: 1a (0.3 mmol), 2 (0.6 mmol), Cu(OAc)2 (0.3 mmol), (tBuO)2 (0.75 mmol), Py (0.9 mmol), DME/1,4-dioxane (v/v = 7 : 3, 1.0 mL), 140 °C, 16 h.
Isolated yield.
2 (1.2 mmol), run at 160 °C.
|
|
Next, the substrate scope study of aliphatic amides was investigated (Table 3). As expected, 2,2-disubstituted propanamides bearing either the linear or cyclic chains provided the corresponding desired products in good yields with good functional group compatibility. In addition, a predominant preference for functionalizing the C–H bonds of the α-methyl over those of the α-methylene, β- or γ-methyl groups, was observed in all cases, presumably due to the steric effect.9 Notably, both mono- and di-pentafluoro-substituted coupling products were obtained with 2,2-dimethyl butanamide and trifluropropanamide (3w and 3x). Interestingly, only the mono-coupling products were observed with α-phthalimide and α-sulfone-substituted amides, which is believed to be due to the steric effects (3y and 3z). Not surprisingly, both mono- and bis-coupling products were isolated with N-(quinolin-8-yl)pivalamide (3aa). Moreover, it was found that a tertiary α-carbon is necessary for this reaction since amides 4 and 5 failed to provide the corresponding desired products. Unfortunately, functionalization of secondary β-sp3 carbons was not successful (6 and 7).
Table 3 Scope of amidesab
To gain some mechanistic insights into this reaction, a series of deuterium-labeling experiments were carried out (Scheme 2). Considering that tBuOH is generated from (tBuO)2 as a byproduct in the process, stoichiometric amounts of tBuOD was added to the reaction system for this study. It was noted that an apparent H/D exchange occurred with pentafluorobenzene (2a) with or without 2-ethyl-2-methyl-N-(quinolin-8-yl)butanamide (1b), indicating that C–H bond cleavage of fluorobenzene is a reversible step. Furthermore, either a small or trace amount of [D]-2a was observed in the absence of Cu(OAc)2 or pyridine, while the obvious H–D scrambling occurred without (tBuO)2. It should be mentioned that H–D scrambling could also be promoted by CuOAc instead of Cu(OAc)2 in the absence of (tBuO)2. These results suggest that the copper species promotes the sp2 C–H bond cleavage with the pyridine as a base and ligand to facilitate the process, and a pyridine-coordinated aryl copperII or aryl copperI intermediate may be involved in the reaction.4e–i In the study, it was found that H/D exchange did not happen with 1b in the absence of 2a, indicating that an aryl copper intermediate may be involved in the sp3 C–H bond cleavage step of the amide.
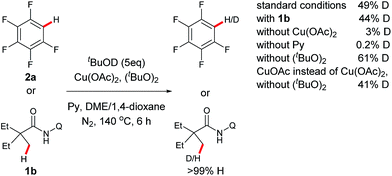 |
| Scheme 2 Deuterium labeling experiments. | |
We further carried out deuterium-labeling experiments with [D3]-1b. As shown in Scheme 3, a H/D exchange was not observed with either this substrate or the product, suggesting that sp3 C–H bond cleavage is an irreversible step. In addition, a second order kinetic isotope effect was observed with 1b in the process, indicating that cyclometalation of the amide is not the rate-limiting step.
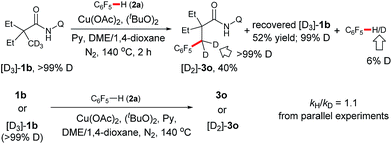 |
| Scheme 3 Deuterium labeling experiments of amides. | |
On the basis of the above observed results and the previous reports,4,9,12 a plausible mechanism for this reaction is proposed (Scheme 4). It is believed that this process begins with the reversible C–H cupration of a fluoro(hetero)arene with Cu(OAc)2 in the presence of pyridine. Coordination of amide 1 to this CuII species followed by a ligand exchange step gives rise to the CuII intermediate B. Subsequent oxidation of the CuII species B generates the CuIII intermediate C, which undergoes an intramolecular cyclometalation step to provide the CuIII complex D. Reductive elimination of this intermediate followed by a ligand dissociation process affords the product 3 and a CuI species. Oxidation of the CuI species by (tBuO)2 regenerates the CuII species. Alternatively, on the basis of the observations in Scheme 2, the cupration of fluoro(hetero)arene 2 could take place with the CuI species, providing the CuI intermediate F which could then be oxidized to the CuII intermediate A by (tBuO)2.
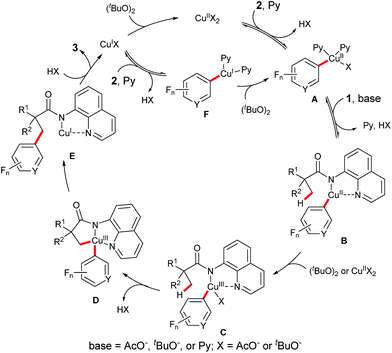 |
| Scheme 4 Plausible reaction mechanism. | |
Conclusions
In summary, copper-promoted pyridine-enabled cross dehydrogenative coupling of aromatic sp2 C–H bonds and unactivated aliphatic sp3 C–H bonds was developed with high efficiency and good functional group tolerance. In this process, high regioselectivity was observed with sp2 C–H bond functionalization, favoring an sp2 C–H bond between two C–F bonds of (hetero)arenes. In addition, a predominant preference for functionalizing the sp3 C–H bonds of α-methyl groups over those of the α-methylene, β- or γ-methyl groups was observed with aliphatic amides. Mechanistic studies suggested that sp2 C–H bond cleavage is a reversible step while sp3 C–H bond cleavage is an irreversible but not the rate-limiting step. Interestingly, it was also found that sp3 C–H bond cleavage is dependent on sp2 C–H bond cleavage. The detailed mechanistic studies and potential synthetic applications of this process are currently under investigation in our laboratory.
Acknowledgements
The authors gratefully acknowledge NSF CHE-1350541 and Indiana University-Purdue University Indianapolis for financial support.
Notes and references
- For selected recent reviews, see:
(a) O. Daugulis, H.-Q. Do and D. Shabashov, Acc. Chem. Res., 2009, 42, 1074 CrossRef CAS PubMed;
(b) X. Chen, K. M. Engle, D.-H. Wang and J.-Q. Yu, Angew. Chem., Int. Ed., 2009, 48, 5094 CrossRef CAS PubMed;
(c) M. Wasa, K. M. Engle and J.-Q. Yu, Isr. J. Chem., 2010, 50, 605 CrossRef CAS PubMed;
(d) D. A. Colby, R. G. Bergman and J. A. Ellman, Chem. Rev., 2010, 110, 624 CrossRef CAS PubMed;
(e) T. W. Lyons and M. S. Sanford, Chem. Rev., 2010, 110, 1147 CrossRef CAS PubMed;
(f) R. Jazzar, J. Hitce, A. Renaudat, J. Sofack-Kreutzer and O. Baudoin, Chem. –Eur. J., 2010, 16, 2654 CrossRef CAS PubMed;
(g) L. Ackermann, Chem. Commun., 2010, 46, 4866 RSC;
(h) H. Li, B.-J. Li and Z.-J. Shi, Catal. Sci. Technol., 2011, 1, 191 RSC;
(i) N. Yoshikai, Synlett, 2011, 1047 CrossRef CAS;
(j) L. Ackermann, Chem. Rev., 2011, 111, 1315 CrossRef CAS PubMed;
(k) H. M. L. Davis, J. Du Bois and J.-Q. Yu, Chem. Soc. Rev., 2011, 40, 1855 RSC;
(l) W. R. Gutekunst and P. S. Baran, Chem. Soc. Rev., 2011, 40, 1976 RSC;
(m) J. F. Hartwig, Chem. Soc. Rev., 2011, 40, 1992 RSC;
(n) T. Newhouse and P. S. Baran, Angew. Chem., Int. Ed., 2011, 50, 3362 CrossRef CAS PubMed;
(o) O. Baudoin, Chem. Soc. Rev., 2011, 40, 4902 RSC;
(p) M. C. White, Science, 2012, 335, 807 CrossRef CAS PubMed;
(q) D. A. Colby, A. S. Tsai, R. G. Bergman and J. A. Ellman, Acc. Chem. Res., 2012, 45, 814 CrossRef CAS PubMed;
(r) P. B. Arockiam, C. Bruneau and P. H. Dixneuf, Chem. Rev., 2012, 112, 5879 CrossRef CAS PubMed;
(s) J. A. D. Yamaguchi and K. Itami, Angew. Chem., Int. Ed., 2012, 51, 8960 CrossRef CAS PubMed;
(t) K. Collins and F. Glorius, Nat. Chem., 2013, 5, 597 CrossRef CAS PubMed;
(u) K. M. Engle and J.-Q. Yu, J. Org. Chem., 2013, 78, 8927 CrossRef CAS PubMed;
(v) R. Rossi, F. Bellina, M. Lessi and C. Manzini, Adv. Synth. Catal., 2014, 356, 17 CrossRef CAS PubMed;
(w) X.-X. Guo, D.-W. Gu, Z. Wu and W. Zhang, Chem. Rev., 2015, 115, 1622 CrossRef CAS PubMed.
- For selected reviews, see:
(a) C. S. Yeung and V. M. Dong, Chem. Rev., 2011, 111, 1215 CrossRef CAS PubMed;
(b) C. Liu, H. Zhang and A. Lei, Chem. Rev., 2011, 111, 1780 CrossRef CAS PubMed;
(c) S. H. Cho, J. Y. Kim, J. Kwak and S. Chang, Chem. Soc. Rev., 2011, 40, 5068 RSC;
(d)
Y. Yamamoto, in Copper-Mediated Cross-Coupling Reactions, ed. G. Evano and N. Blanchard, John Wiley & Sons, Inc., Hoboken, NJ, USA, 2013, p. 335 Search PubMed;
(e)
W. Su, M. Zhang and G. Wu, Double C–H activation in Pd-catalyzed cross-coupling reactions of non-preactivated arenes, in C–H and C–X Bond Functionalization: Transition Metal Mediation, 2013, Royal Society of Chemistry, UK, vol. 11, p. 328 Search PubMed;
(f) R. Kumar and E. V. van der Eychen, Chem. Soc. Rev., 2013, 42, 1121 RSC;
(g) Y. Wu, J. Wang, F. Mao and F. Y. Kwong, Chem.–Asian J., 2014, 9, 26 CrossRef CAS PubMed;
(h) S. A. Girard, T. Knauber and C.-J. Li, Angew. Chem., Int. Ed., 2014, 53, 74 CrossRef CAS PubMed;
(i) S. De Sarkar, W. Liu, S. I. Kozhushkov and L. Ackermann, Adv. Synth. Catal., 2014, 356, 1461 CrossRef CAS PubMed;
(j) M. Zhang, Y. Zhang, X. Jie, H. Zhao, G. Li and W. Su, Org. Chem. Front., 2014, 1, 843 RSC.
- M. Kitahara, N. Umeda, K. Hirano, T. Satoh and M. Miura, J. Am. Chem. Soc., 2011, 133, 2160 CrossRef CAS PubMed.
- For selected recent reviews, see:
(a) M. Miura, T. Satoh and K. Hirano, Bull. Chem. Soc. Jpn., 2014, 87, 751 CrossRef CAS;
(b) K. Hirano and M. Miura, Chem. Commun., 2012, 48, 10704 RSC;
(c) K. Hirano and M. Miura, Top. Catal., 2014, 57, 878 CrossRef CAS;
(d) J. Dong, Q. Wu and J. You, Tetrahedron Lett., 2015, 56, 1591 CrossRef CAS PubMed . For examples: ;
(e) M. Nishino, K. Hirano, T. Satoh and M. Miura, Angew. Chem., Int. Ed., 2012, 51, 6993 CrossRef CAS PubMed;
(f) M. Nishino, K. Hirano, T. Satoh and M. Miura, Angew. Chem., Int. Ed., 2013, 52, 4457 CrossRef CAS PubMed;
(g) R. Odani, K. Hirano, T. Satoh and M. Miura, J. Org. Chem., 2013, 78, 11045 CrossRef CAS PubMed;
(h) R. Odani, K. Hirano, T. Satoh and M. Miura, Angew. Chem., Int. Ed., 2014, 53, 10784 CrossRef CAS PubMed;
(i) R. Odani, K. Hirano, T. Satoh and M. Miura, J. Org. Chem., 2015, 80, 2384 CrossRef CAS PubMed.
- For Cu-catalyzed and/or promoted CDC between sp2 and sp2/sp C-H bonds, see:
(a) J. Dong, F. Wang and J. You, Org. Lett., 2014, 16, 2884 CrossRef CAS PubMed;
(b) W. Zhu, D. Zhang, N. Yang and H. Liu, Chem. Commun., 2014, 50, 10634 RSC;
(c) M. Shang, H.-L. Wang, S.-Z. Sun, H.-X. Dai and J.-Q. Yu, J. Am. Chem. Soc., 2014, 136, 11590 CrossRef CAS PubMed;
(d) Y.-J. Liu, Y.-H. Liu, X.-S. Yin, W.-J. Gu and B.-F. Shi, Chem. –Eur. J., 2015, 21, 205 CrossRef CAS PubMed;
(e) H.-L. Wang, M. Shang, S.-Z. Sun, Z.-L. Zhou, B. N. Laforteza, H.-X. Dai and J.-Q. Yu, Org. Lett., 2015, 17, 1228 CrossRef CAS PubMed.
- For selected examples of transition metal-catalyzed CDC between sp2 and sp3 C–H bonds, see:
(a) G. Deng, L. Zhao and C.-J. Li, Angew. Chem., Int. Ed., 2008, 47, 6278 CrossRef CAS PubMed;
(b) S. Guin, S. K. Rout, A. Banerjee, S. Nandi and B. K. Patel, Org. Lett., 2012, 14, 5294 CrossRef CAS PubMed;
(c) C. Pierre and O. Baudoin, Tetrahedron, 2013, 69, 4473 CrossRef CAS PubMed;
(d) Y. Aihara, M. Tobisu, Y. Fukumoto and N. Chatani, J. Am. Chem. Soc., 2008, 130, 1128 CrossRef PubMed.
- For a pioneering example of using 8-aminoquinoline as the bidentate directing group in C(sp3)–H activation, see: V. Zaitsev, D. Shabashov and O. Daugulis, J. Am. Chem. Soc., 2005, 127, 13154 CrossRef CAS PubMed.
- For selected recent reviews, see:
(a) M. Corbet and F. de Campo, Angew. Chem., Int. Ed., 2013, 52, 9896 CrossRef CAS PubMed;
(b) G. Rouquet and N. Chatani, Angew. Chem., Int. Ed., 2013, 52, 11726 CrossRef CAS PubMed;
(c) O. Daugulis, J. Roane and L. D. Tran, Acc. Chem. Res., 2015, 48, 1053 CrossRef CAS PubMed. For selected recent examples, see:
(d) W.-W. Sun, P. Cao, R.-Q. Mei, Y. Li, Y.-L. Ma and B. Wu, Org. Lett., 2014, 16, 480 CrossRef CAS PubMed;
(e) Y. Aihara and N. Chatani, J. Am. Chem. Soc., 2014, 136, 898 CrossRef CAS PubMed;
(f) X. Wu, Y. Zhao and H. Ge, J. Am. Chem. Soc., 2014, 136, 1789 CrossRef CAS PubMed;
(g) K. S. Kanyiva, Y. Kuninobu and M. Kanai, Org. Lett., 2014, 16, 1968 CrossRef CAS PubMed;
(h) W. R. Gutekunst and P. S. Baran, J. Org. Chem., 2014, 79, 2430 CrossRef CAS PubMed;
(i) M. Li, J. Dong, X. Huang, K. Li, Q. Wu, F. Song and J. You, Chem. Commun., 2014, 50, 3944 RSC;
(j) Q. Gu, H. H. Al Mamari, K. Graczyk, E. Diers and L. Ackermann, Angew. Chem., Int. Ed., 2014, 53, 3868 CrossRef CAS PubMed;
(k) X. Wu, Y. Zhao and H. Ge, Chem. –Eur. J., 2014, 20, 9530 CrossRef CAS PubMed;
(l) C. Wang, C. Chen, J. Zhang, J. Han, Q. Wang, K. Guo, P. Liu, M. Guan, Y. Yao and Y. Zhao, Angew. Chem., Int. Ed., 2014, 53, 9884 CrossRef CAS PubMed;
(m) X. Li, Y.-H. Liu, W.-J. Gu, B. Li, F.-J. Chen and B.-F. Shi, Org. Lett., 2014, 16, 3904 CrossRef CAS PubMed;
(n) D. P. Affron, O. A. Davis and J. A. Bull, Org. Lett., 2014, 16, 4956 CrossRef CAS PubMed;
(o) R. K. Rit, M. R. Yadav, K. Ghosh, M. Shankar and A. K. Sahoo, Org. Lett., 2014, 16, 5258 CrossRef CAS PubMed;
(p) Y. Zong and Y. Rao, Org. Lett., 2014, 16, 5278 CrossRef CAS PubMed;
(q) W. Gong, G. Zhang, T. Liu, R. Giri and J.-Q. Yu, J. Am. Chem. Soc., 2014, 136, 16940 CrossRef CAS PubMed;
(r) B. Wang, C. Lu, S.-Y. Zhang, G. He, W. A. Nack and G. Chen, Org. Lett., 2014, 16, 6260 CrossRef CAS PubMed;
(s) S. Y. Zhang, Q. Li, G. He, W. A. Nack and G. Chen, J. Am. Chem. Soc., 2015, 137, 531 CrossRef CAS PubMed;
(t) K. Chen, S.-Q. Zhang, H.-Z. Jiang, J.-W. Xu and B.-F. Shi, Chem. –Eur. J., 2015, 21, 3264 CrossRef CAS PubMed;
(u) X. Wu, K. Yang, Y. Zhao, H. Sun, G. Li and H. Ge, Nat. Commun., 2015, 6, 6442 CrossRef PubMed;
(v) X. Wu, Y. Zhao and H. Ge, J. Am. Chem. Soc., 2015, 137, 4924 CrossRef CAS PubMed;
(w) C. Lin, W. Yu, J. Yao, B. Wang, Z. Liu and Y. Zhang, Org. Lett., 2015, 17, 1340 CrossRef CAS PubMed;
(x) S.-Y. Yan, Y.-J. Liu, B. Liu, Y.-H. Liu, Z.-Z. Zhang and B.-F. Shi, Chem. Commun., 2015, 51, 7341 RSC;
(y) R. Parella and S. A. Badu, J. Org. Chem., 2015, 80, 2339 CrossRef CAS PubMed;
(z) K. Chen, Z.-W. Li, P.-X. Shen, H.-W. Zhao and Z.-J. Shi, Chem. –Eur. J., 2015, 21, 7389 CrossRef CAS PubMed;
(a
a) X. Ye, J. L. Petersen and X. Shi, Chem. Commun., 2015, 51, 7863 RSC;
(a
b) X. Wang, R. Qiu, C. Yan, V. P. Reddy, L. Zhu, X. Xu and S.-F. Yin, Org. Lett., 2015, 17, 1970 CrossRef CAS PubMed;
(a
c) Y.-J. Liu, Z.-Z. Zhang, S.-Y. Yan, Y.-H. Liu and B.-F. Shi, Chem. Commun., 2015, 51, 7899 RSC.
-
(a) Z. Wang, J. Ni, Y. Kuninobu and M. Kanai, Angew. Chem., Int. Ed., 2014, 53, 3496 CrossRef CAS PubMed;
(b) X. Wu, Y. Zhao, G. Zhang and H. Ge, Angew. Chem., Int. Ed., 2014, 53, 3706 CrossRef CAS PubMed;
(c) X. Wu, Y. Zhao and H. Ge, Chem.–Asian J., 2014, 9, 2736 CrossRef CAS PubMed;
(d) Z. Wang, Y. Kuninobu and M. Kanai, Org. Lett., 2014, 16, 4790 CrossRef CAS PubMed;
(e) J. Zhang, H. Chen, B. Wang, Z. Liu and Y. Zhang, Org. Lett., 2015, 17, 2768 CrossRef CAS PubMed.
- For selected recent examples, see:
(a) H. Q. Do and O. Daugulis, J. Am. Chem. Soc., 2008, 130, 1128 CrossRef CAS PubMed;
(b) H.-Q. Do, R. M. K. Khan and O. Daugulis, J. Am. Chem. Soc., 2008, 130, 15185 CrossRef CAS PubMed;
(c) N. Matsuyama, M. Kitahara, K. Hirano, T. Satoh and M. Miura, Org. Lett., 2010, 12, 2358 CrossRef CAS PubMed;
(d) W. Ye, H. Zhao, J. Kan, W. Su and M. Hong, J. Am. Chem. Soc., 2010, 132, 2522 CrossRef PubMed;
(e) M. Miyasaka, K. Hirano, T. Satoh, R. Kowalczyk, C. Bolm and M. Miura, Org. Lett., 2011, 13, 359 CrossRef CAS PubMed;
(f) N. Matsuda, K. Hirano, T. Satoh and M. Miura, Org. Lett., 2011, 13, 2860 CrossRef CAS PubMed;
(g) T. Yao, K. Hirano, T. Satoh and M. Miura, Angew. Chem., Int. Ed., 2011, 50, 2990 CrossRef CAS PubMed;
(h) H.-Q. Do and O. Daugulis, J. Am. Chem. Soc., 2011, 133, 13577 CrossRef CAS PubMed;
(i) S. Ye, G. Liu, S. Pu and J. Wu, Org. Lett., 2012, 14, 70 CrossRef CAS PubMed;
(j) A. Nakatani, K. Hirano, T. Satoh and M. Miura, Org. Lett., 2012, 14, 2586 CrossRef CAS PubMed;
(k) S. Fan, Z. Chen and X. Zhang, Org. Lett., 2012, 14, 4950 CrossRef CAS PubMed;
(l) L.-H. Zou, J. Mottweiler, D. L. Priebbenow, J. Wang, J. A. Stubenrauch and C. Bolm, Chem. –Eur. J., 2013, 19, 3302 CrossRef CAS PubMed;
(m) S. Xu, G. Wu, F. Ye, X. Wang, H. Li, X. Zhao, Y. Zhang and J. Wang, Angew. Chem., Int. Ed., 2015, 54, 4669 CrossRef CAS PubMed.
- For selected recent reviews, see:
(a) E. A. Meyer, R. K. Castellano and F. Diederich, Angew. Chem., Int. Ed., 2003, 42, 1210 CrossRef CAS PubMed;
(b) J. C. Biffinger, H. W. Kim and S. G. DiMagno, ChemBioChem, 2004, 5, 622 CrossRef CAS PubMed;
(c) H. Amii and K. Uneyama, Chem. Rev., 2009, 109, 2119 CrossRef CAS PubMed;
(d) C. J. Pace and J. Gao, Acc. Chem. Res., 2013, 46, 907 CrossRef CAS PubMed;
(e) B. H. Hoff and E. Sundby, Bioorg. Chem., 2013, 51, 31 CrossRef CAS PubMed;
(f) T. Liang, C. N. Neumann and T. Ritter, Angew. Chem., Int. Ed., 2013, 52, 8214 CrossRef CAS PubMed.
-
(a) H. Furuta, H. Maeda and A. Osuka, J. Am. Chem. Soc., 2000, 122, 803 CrossRef CAS;
(b) X. Ribas, D. A. Jackson, B. Donnadieu, J. Mahía, T. Parella, R. Xifra, B. Hedman, K. O. Hodgson, A. Llobet and T. D. P. Stack, Angew. Chem., Int. Ed., 2002, 41, 2991 CrossRef CAS;
(c) H. Maeda, A. Osuka and H. Furuta, J. Am. Chem. Soc., 2003, 125, 15690 CrossRef CAS PubMed;
(d) R. Xifra, X. Ribas, A. Llobet, A. Poater, M. Duran, M. Solà, T. D. P. Stack, J. Benet-Buchholz, B. Donnadieu, J. Mahía and T. Parella, Chem. –Eur. J., 2005, 11, 5146 CrossRef CAS PubMed;
(e) M. Pawlicki, I. Kańska and L. Latos-Graźyński, Inorg. Chem., 2007, 46, 6575 CrossRef CAS PubMed;
(f) L. M. Huffman and S. S. Stahl, J. Am. Chem. Soc., 2008, 130, 9196 CrossRef CAS PubMed;
(g) B. Yao, D.-X. Wang, Z.-T. Huang and M.-X. Wang, Chem. Commun., 2009, 2899 RSC;
(h) A. E. King, L. M. Huffman, A. Casitas, M. Costas, X. Ribas and S. S. Stahl, J. Am. Chem. Soc., 2010, 132, 12068 CrossRef CAS PubMed;
(i) X. Ribas, C. Calle, A. Poater, A. Casitas, L. Gómez, R. Xifra, T. Parella, J. Benet-Buchholz, A. Schweiger, G. Mitrikas, M. Solà, A. Llobet and T. D. P. Stack, J. Am. Chem. Soc., 2010, 132, 12299 CrossRef CAS PubMed;
(j) A. M. Suess, M. Z. Ertem, C. J. Cramer and S. S. Stahl, J. Am. Chem. Soc., 2013, 135, 9797 CrossRef CAS PubMed.
Footnote |
† Electronic supplementary information (ESI) available: Experimental details including characterization data, copies of 1H, 13C NMR and NOESY spectra. See DOI: 10.1039/c5sc02143j |
|
This journal is © The Royal Society of Chemistry 2015 |