DOI:
10.1039/C5SC01950H
(Edge Article)
Chem. Sci., 2015,
6, 5964-5968
Gold-catalyzed formal [4π + 2π]-cycloadditions of propiolate derivatives with unactivated nitriles†
Received
1st June 2015
, Accepted 17th July 2015
First published on 20th July 2015
Abstract
Gold-catalyzed hetero-[4π + 2π]-cycloadditions of tert-butyl propiolates with unactivated nitriles are described; the resulting 6H-1,3-oxazin-6-ones are not easily accessible via conventional methods. This new finding enables a one-pot gold-catalyzed synthesis of highly substituted pyridines through sequential gold-catalyzed reactions of tert-butyl propiolates with nitriles, and then with electron-deficient alkynes in the same solvent. The utility of these [4 + 2]-cycloadditions is further expanded with various aldehydes, ketones and 2-phenyloxetane, yielding satisfactory yields of cycloadducts.
Introduction
Metal-catalyzed [4π + 2π]-cycloadditions are powerful tools for the construction of carbo- or heterocyclic frameworks.1,2 Although common nitriles and alkynes represent common triple bond motifs, nitriles are generally less reactive than alkynes in catalytic [4π + 2π]-cycloadditions; the chemical stability of nitriles is reflected by their bond energy (854 kJ mol−1), being larger than that of alkynes (835 kJ mol−1).3 For instance, thermal [4π + 2π]-cycloadditions of dienes with unactivated nitriles required 600 °C (2 min) to give pyridine derivatives in 0.1–0.5% yields.4a In the context of catalytic [4π + 2π]-cycloadditions, not surprisingly, only one literature report documents both nitrile/1,3-diene and nitrile/1,3-enyne systems (eqn (1) and (2)).4b,c Ogoshi reported the first formal [4 + 2]-cycloadditions of common nitriles with dienes using Ni(0) catalysts (eqn (1)).4b Although Barluenga and Aguilar reported formal [4π + 2π]-cycloadditions of some 3-en-1-ynes with unactivated nitriles,4c such highly functionalized 3-en-1-ynes (X = cis-unsaturated ester, Z = alkoxy) are too specialized to reflect the reaction generality (eqn (2)). The [4π + 2π]-nitrile cycloadditions still remain an unsolved task for O- and N-substituted analogues of 1,3-dienes and 1,3-enynes (X = O, NR′, eqn (1) and (2)).5 In a significant advance, we here report the gold-catalyzed formal hetero-[4π + 2π]-cycloadditions6,7 of various propiolates with nitriles to afford 6H-1,3-oxazin-6-ones efficiently (eqn (3)).8 These findings enable the development of new cascade cycloadditions using three π-motifs including propiolates, nitriles and alkynes, yielding highly substituted pyridine derivatives. Notably, 6H-1,3-oxazin-6-ones are useful intermediates in various organic reactions whereas highly substituted pyridines are important structural cores commonly found in many bioactive molecules (see ESI Fig. S1†);9,10 their availability from convenient t-butyl propiolates increases the synthetic utility of this gold catalysis. |  | (1) |
| 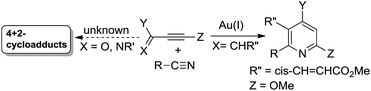 | (2) |
| 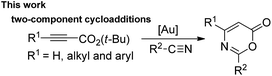 | (3) |
| 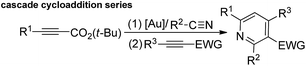 | (4) |
Results and discussion
We envisage that direct [4π + 2π]-cycloadditions of propiolate derivatives with nitriles provide the most convenient synthesis of 6H-1,3-oxazin-6-ones such as 3; the current procedures rely mainly on thermal rearrangement of N-acyl β-lactams.8a–d To test the feasibility, as shown in Table 1, tert-butyl hept-2-ynoate (1a, 1 equiv.) was treated with benzonitrile 2a (3 equiv.) and AuCl3 (5 mol%) in hot DCE (70 °C, 16 h), affording the desired product 3a in only a small yield (5%) together with the initial 1a in 45% recovery (entry 1). The use of PPh3AuCl/AgSbF6 significantly increased the yield of the desired 3a to 51% (entry 2). We also examined other cationic gold catalysts (5 mol%) including IPrAuCl/AgSbF6 and P(t-Bu)2(o-biphenyl)AuCl/AgSbF6, yielding compound 3a in 64% and 85% yields, respectively (see entries 3 and 4). With the alteration of the silver salts as in P(t-Bu)2(o-biphenyl)AuCl/AgX (X = NTf2 and OTf), the product yields slightly decreased to 77% and 72%, respectively (entries 5 and 6). AgSbF6 (70 °C, 24 h) and Zn(OTf)2 (19 h) were found to be inactive in DCE, leading to a recovery of the starting compound 1a in 72–75% yield (entries 7 and 8). The use of In(OTf)3, Sc(OTf)3 and TfOH in DCE gave hept-2-ynoic acid 1a′ in 65–72% yield and amide species 2a-H (25–35% yield) along with unreacted starting compound 1a (5–15% yield, entries 9–11). The yields of compound 3a varied with the solvents (70 °C), with 65% in toluene (22 h), 82% in C6H5Cl (18 h) and 56% in 1,4-dioxane (19 h, entries 12–14).
Table 1 Tests of propiolate derivatives with gold catalysts
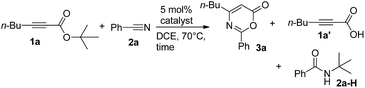
|
Entries |
Catalyst |
Solvent |
Time (h) |
Yieldsa,b (%) |
1a
|
3a
|
1a′
|
2a-H
|
[1a] = 0.18 M.
Product yields are reported after purification using a silica column. IPr = 1,3-bis(diisopropyl phenyl)-imidazol-2-ylidene, L = P(t-Bu)2(o-biphenyl), Tf = trifluoromethansesulfonyl.
Reactions carried out at room temperature.
|
1 |
AuCl3 |
DCE |
16 |
45 |
5 |
— |
— |
2 |
Ph3PAuCl/AgSbF6 |
DCE |
12 |
— |
51 |
— |
— |
3 |
IPrAuCl/AgSbF6 |
DCE |
19 |
— |
64 |
— |
— |
4 |
LAuCl/AgSbF6 |
DCE |
18 |
— |
85 |
— |
— |
5 |
LAuCl/AgNTf2 |
DCE |
20 |
— |
77 |
— |
— |
6 |
LAuCl/AgOTf |
DCE |
22 |
— |
72 |
— |
— |
7 |
AgSbF6 |
DCE |
24 |
75 |
— |
|
|
8 |
Zn(OTf)2c |
DCE |
19 |
72 |
— |
— |
— |
9 |
In(OTf)3c |
DCE |
18 |
15 |
— |
72 |
35 |
10 |
Sc(OTf)3c |
DCE |
22 |
10 |
— |
65 |
32 |
11 |
HOTfc |
DCE |
15 |
5 |
— |
67 |
25 |
12 |
LAuCl/AgSbF6 |
Toluene |
22 |
— |
65 |
— |
— |
13 |
LAuCl/AgSbF6 |
C6H5Cl |
18 |
— |
82 |
— |
— |
14 |
LAuCl/AgSbF6 |
1,4-Dioxane |
19 |
— |
56 |
— |
— |
Table 2 assesses the reaction generality using various propiolate derivatives with varied nitriles. We first examined the reactions with unsubstituted propiolate species 1b; its cycloaddition with benzonitrile 2a proceeded smoothly to form the formal cycloadduct 3b in 65% yield (entry 1). The reaction scope is extensible to aliphatically substituted propiolate species 1c–1e (R = isopropyl, cyclopropyl and cyclohexyl), yielding the desired products 3c–3e in satisfactory yields (77–85%, entries 2–4). This formal cycloaddition is also applicable to alkenyl-substituted propiolate 1f to afford the corresponding product 3f in 68% yield (entry 5). We tested the reactions on various phenyl-substituted propiolate species 1g–1j bearing various para-substituents (X = H, OMe, F and Cl); their resulting cycloadducts 3g–3j were obtained in satisfactory yields (65–72%, entries 6–9). We performed an X-ray diffraction study of product 3g to confirm its molecular structure.11 We also prepared 2- and 3-thienyl-substituted propiolate derivatives 1k and 1l; their reactions with benzonitrile afforded cycloadducts 3k and 3l in reasonable yields (entries 10 and 11, 55–58%). Entries 12–15 show the tests of tert-butyl hept-2-ynoate 1a with benzonitriles 2b–2e bearing various para-substituents (X = OMe, Me, CO2Me, Cl) that afforded the desired cycloadducts 3m–3p in satisfactory yields (62–76%). These catalytic cycloadditions were compatible with disparate nitriles including cyclohexyl nitrile (2f), cinnamonitrile (2g) and 3-thienyl nitrile (2h), affording the expected products 3q–3s in satisfactory yields (66–78%, entries 16–18).
Table 2 Formal cycloadditions of various propiolates with nitriles

|
2 (3 equiv.), [1] = 0.18 M.
Product yields are reported after purification using a silica column. L = P(t-Bu)2(o-biphenyl).
|
|
As inferred from the chemistry of 2H-pyran-2-ones,12,13 one representative compound 3a (1 equiv.) was treated with diethyl but-2-ynedioate (4 equiv.) in hot p-xylene (150 °C, 10 h) to afford tetrasubstituted pyridine 5a in 96% yield; this reaction sequence presumably proceeds with intermediate I that is prone to a loss of CO2 (eqn (5)). As chlorobenzene is also an effective solvent for such a nitrile/propiolate cycloaddition (Table 1, entry 9), we developed a one-pot reaction involving the prior heating of a chlorobenzene solution of propiolate derivative 1a, benzonitrile (3 equiv.) and P(t-Bu)2(o-biphenyl) AuCl/AgSbF6 (5 mol%) at 70 °C (18 h) in a sealed tube to ensure a complete consumption of starting compound 1a; to this solution was added diethyl but-2-ynedioate (4 equiv.) with further heating at 150 °C for 20 h. This one-pot process delivered the desired pyridine 5a in 80% yield (eqn (6)). If the three reactants in the same proportions were heated together with a gold catalyst in hot chlorobenzene (150 °C, 20 h), the yield of 5a was decreased to 38% yield.
| 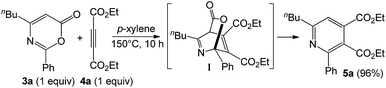 | (5) |
| 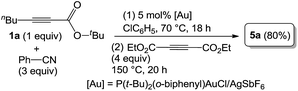 | (6) |
The easy operation of this one-pot reaction inspires us to examine the scope of the reaction using various propiolates, nitriles and alkynes; the results are summarized in Table 3. The procedures follow exactly that described in eqn (6). In the second stage of heating, the temperature is 150 °C for entries 1–7 and 180 °C for entries 8–12. Entry 1 shows the compatibility of these cycloadditions with unsubstituted propiolate derivative 1b (R = H) that reacted sequentially with benzonitrile (2a) and diethyl but-2-ynedioate (4a) to yield the desired pyridine 5b in 45% yield. We also tested the reactions on various alkyl-substituted propiolates 1c–1e (R = isopropyl, cyclopropyl and cyclohexyl) that reacted with the same alkyne and benzonitrile to afford the desired pyridine species 5c–5e in 73–76% yields (entries 2–4). The reaction is further applicable to aryl-substituted propiolates 1g and 1l (R = Ph, 3-thienyl) to deliver the desired pyridines 5f and 5g in 61% and 51% yield, respectively (entries 5 and 6). We tested the reactions of model propiolate (1a) and diethyl but-2-ynedioate (4a) with various nitriles (R1 = cyclohexyl, 3-thienyl and trans-styryl), affording the expected pyridine products 5h–5j in satisfactory yields (68–75%, entries 7–9). The reactions were extensible to various unsymmetric alkynes 4b–4f that reacted with propiolate (1a) and benzonitrile (2a) with excellent or high regioselectivity (entries 11–15). The reactions worked well for terminal alkynes 4b (EWG = COOMe) and 4c (EWG = COPh) to afford the desired pyridines 5k and 5l as single regioisomers, with respective yields of 83% and 76% (entries 10 and 11). For n-butyl propiolate 4d, this one-pot sequence gave two inseparable isomeric products 5m/5m′ = 4/1, in a combined 82% yield (entry 12). For the other n-butyl and phenyl-substituted ynones 4e and 4f (EWG = COPh), their reactions afforded 5n and 5o with excellent regioselectivity and satisfactory yields (81–84%) (entries 13–14). The structures of representative compounds 5m and 5n were confirmed by proton NOE effects whereas the structure of cycloadduct 5o was elucidated with an HMBC experiment (see ESI†).
Table 3 One-pot operations with nitriles, propiolates and alkynes
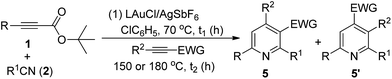
|
5 mol% gold catalyst, L = P(t-Bu)2(o-biphenyl), R1CN (3 equiv.), R2CC–EWG (4 equiv.), 150 °C for entries 1–9 and 180 °C for entries 10–14.
These data correspond to the reaction time t1/t2.
|
|
As nitriles are weakly nucleophilic, we envisage that aldehydes and ketones might be applicable substrates. To our pleasure, gold-catalyzed reactions of 3-phenylpropiolate 1g with benzaldehyde, phenyl methyl ketone and acetone in hot dichloroethane (DCE) proceeded smoothly to afford formal cycloadducts 6a–6c in high yields (86–89%, eqn (7)). The structure of compound 6a was determined by X-ray diffraction.11 These carbonyl cycloadditions were also applicable to alkyl-substituted propiolates (1a) and (1e), yielding the desired compounds 6d and 6e in 87% and 77% yield, respectively (eqn (8)). Such a reaction was, notably, accessible to an eight-membered oxacyclic compound 6f with 2.5 mol% 1,3-bis(diisopropyl phenyl)-imidazol-2-ylidene AuSbF6; it was isolated as a single regioisomer with 67% yield with 2-phenyloxetane (3 equiv.) and its molecular structure has been confirmed by X-ray diffraction.11 The compatibility of this gold catalysis with aldehydes, ketones and oxetanes truly reflects a broad applicability of these cycloadditions.
| 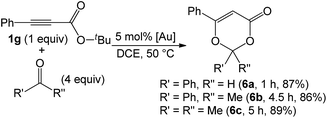 | (7) |
| 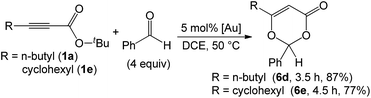 | (8) |
|  | (9) |
Prior to this work, Shin reported gold-catalyzed [4 + 2]-cycloadditions of alkenes with propiolic acid, which was, however, the only applicable substrate.6a Here, we employ diverse propiolate substrates to comply with not only nitriles but also aldehydes, ketones and oxetanes. To understand this discrepancy, we performed the reaction of 3-phenylpropiolic acid (1g′) with benzonitrile with the same gold catalyst in DCE, but the yield of the desired compound 1g was only 8%, much smaller than that (68%) of its tert-butoxy derivative 1g (Table 2, entry 5). Clearly, prior transformations of t-butoxy propiolates to the propiolate acids do not occur in the course of the reactions. For ethyl propiolate 1g′′, its corresponding reaction with benzonitrile gave the amide-addition product 3g′ in 68% yield (eqn (10)); under this condition, benzonitrile was not effectively transformed into benzamide with this gold catalyst.14
| 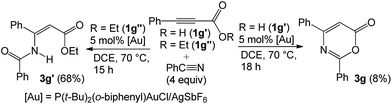 | (10) |
The control experiments in eqn (10) indicate a mechanism involving a prior formation of nitrilium species Bvia a π-alkyne activation, proceeding with an attack of nitrile at the gold-π-alkyne species A. As shown in Scheme 1, we postulate that the tert-butyoxy group of species B increases the nucleophilicity of a carbonyl group to attack this nitrilium moiety efficiently. This process releases a tert-butyl cation to induce a demetalation to form the observed cycloadduct 3g. Beside nitriles, various aldehydes, ketones and oxetanes are more reactive than alkenes upon comparison of their applicable propiolates. We postulate that these nucleophiles generate intermediates B, E and F bearing a large positive charge on the reacting Cα-carbons because of their adjacent oxonium and ammonium centers. We envisage that the propiolate cycloadditions match well with those nucleophiles that can develop highly polarized carbocations through π-alkyne activations.
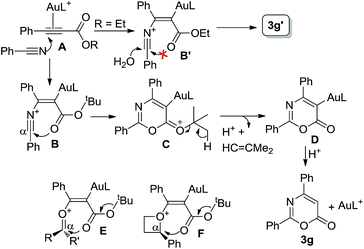 |
| Scheme 1 A postulated reaction mechanism. | |
Conclusions
Unactivated nitriles are known to be stable triple-bond species, and their [4 + 2]-cycloadditions with 4π-bond motifs and other small molecules have few successful examples.15 This work reports the hetero-[4π + 2π]-cycloadditions of tert-butyl propiolates and nitriles catalyzed by gold catalysts. Such formal cycloadditions are applicable to diverse tert-butyl propiolates and nitriles, yielding useful 6H-1,3-oxazin-6-ones, which are not readily prepared with current methods.8 This new finding enables a one-pot gold-catalyzed synthesis of highly substituted pyridines through sequential reactions of tert-butyl propiolates with nitriles, and then with electron-deficient alkynes in the same solvent. The utility of these [4 + 2]-cycloadditions is further expanded with various aldehydes, ketones and 2-phenyloxetane, yielding satisfactory yields of cycloadducts. This work provides a new version of tert-butyl propiolates that feature useful four-atom building blocks with polar π-bond motifs such as nitriles, aldehydes and ketones, although their reactions with alkenes were reported to be restrictive.8
Acknowledgements
We thank the National Science Council, Taiwan, for financial support of this work.
Notes and references
- For metal-catalyzed [4 + 2]-cycloaddition reactions, see selected reviews:
(a) M. Lautens, W. Klute and W. Tam, Chem. Rev., 1996, 96, 49–92 CrossRef CAS PubMed;
(b) P. A. Inglesby and P. A. Evans, Chem. Soc. Rev., 2010, 39, 2791–2805 RSC;
(c) X. Jiang and R. Wang, Chem. Rev., 2013, 113, 5515–5546 CrossRef CAS PubMed;
(d) H. B. Kagan and O. Riant, Chem. Rev., 1992, 92, 1007–1019 CrossRef CAS;
(e) I. Bauer and H.-J. Knölker, Chem. Rev., 2015, 115, 3170–3387 CrossRef CAS PubMed.
- For gold-catalyzed cycloaddition reactions see:
(a) M. E. Muratore, A. Homs, C. Obradors and A. M. Echavarren, Chem.–Asian J., 2014, 9, 3066–3082 CrossRef CAS PubMed;
(b) F. López and J. L. Mascareñas, Chem. Soc. Rev., 2014, 43, 2904–2915 RSC;
(c) F. López and J. L. Mascareñas, Beilstein J. Org. Chem., 2013, 9, 2250–2264 CrossRef PubMed;
(d) N. T. Patil and Y. Yamamoto, Chem. Rev., 2008, 108, 3395–3442 CrossRef CAS PubMed;
(e) A. S. K. Hashmi, Chem. Rev., 2007, 107, 3180–3211 CrossRef CAS PubMed;
(f) D. Qian and J. Zhang, Chem. Rec., 2014, 14, 280–302 CrossRef CAS PubMed;
(g) J. Francos, F. Grande Carmona, H. Faustino, J. Iglesias Sigüenza, E. Díez, I. Alonso, R. Fernández, J. M. Lassaletta, F. López and J. L. Mascareñas, J. Am. Chem. Soc., 2012, 134, 14322–14325 CrossRef CAS PubMed;
(h) H. Faustino, F. López, L. Castedo and J. L. Mascareñas, Chem. Sci., 2011, 2, 633–637 RSC;
(i) N. Huguet, D. Leboeuf and A. M. Echavarren, Chem.–Eur. J., 2013, 19, 6581–6585 CrossRef CAS PubMed;
(j) A. Homs, C. Obradors, D. Leboeuf and A. M. Echavarren, Adv. Synth. Catal., 2014, 356, 221–228 CrossRef CAS PubMed.
-
(a) S. J. Blanksby and G. B. Ellison, Acc. Chem. Res., 2003, 36, 255–263 CrossRef CAS PubMed;
(b)
M. B. Smith and J. March, March’s Advanced Organic Chemistry, 2007, 6th edn, p. 29 Search PubMed.
-
(a) C. S. Marvel and J. C. H. Hwa, J. Org. Chem., 1950, 15, 525–529 CrossRef CAS;
(b) M. Ohashi, I. Takeda, M. Ikawa and S. Ogoshi, J. Am. Chem. Soc., 2014, 133, 18018–18021 CrossRef PubMed;
(c) J. Barluenga, M. A. Fernández-Rodríguez, P. García-García and E. Aguilar, J. Am. Chem. Soc., 2008, 130, 2764–2765 CrossRef CAS PubMed.
- For the cycloadditions of 1,3-dienes with activated nitriles, see the following examples; most instances refer to stoichiometric reactions:
(a) W.-T. Li, F.-C. Lai, G.-H. Lee, S.-M. Peng and R.-S. Liu, J. Am. Chem. Soc., 1998, 120, 4520–4521 CrossRef CAS;
(b) J. Chen, Q. Song, C. Wang and Z. Xi, J. Am. Chem. Soc., 2002, 124, 6238–6239 CrossRef CAS PubMed;
(c) H. Junge and G. Oehme, Tetrahedron, 1998, 54, 11027–11032 CrossRef CAS;
(d) I. Hussain, M. A. Yawer, M. Lalk, U. Lindequist, A. Villinger, C. Fischer and P. Langer, Bioorg. Med. Chem. Lett., 2008, 16, 9898–9903 CrossRef CAS PubMed.
- Although gold-catalyzed reactions of propiolic acids with alkenes or alkynes were reported, the mechanisms were distinct from those of this work:
(a) H.-S. Yeom, J. Koo, H.-S. Park, Y. Wang, Y. Liang, Z.-X. Yu and S. Shin, J. Am. Chem. Soc., 2012, 134, 208–211 CrossRef CAS PubMed;
(b) T. Luo, M. Dai, S.-L. Zheng and S. L. Schreiber, Org. Lett., 2011, 13, 2834–2836 CrossRef CAS PubMed.
- For gold-catalyzed cycloadditions of common nitriles with other small molecules, see:
(a) S. N. Karad and R. S. Liu, Angew. Chem., Int. Ed., 2014, 53, 9072–9076 CrossRef CAS PubMed;
(b) W. He, C. Li and L. Zhang, J. Am. Chem. Soc., 2011, 133, 8482–8485 CrossRef CAS PubMed;
(c) Y. Xiao and L. Zhang, Org. Lett., 2012, 14, 4662–4665 CrossRef CAS PubMed;
(d) G. Lonzi and L. A. Lopez, Adv. Synth. Catal., 2013, 355, 1948–1954 CrossRef CAS.
- For reported synthetic procedures of 6H-1,3-oxazin-6-ones, see reported examples:
(a) M. Alajarin, A. Vidal, P. Sánchez-Andrada, F. Tovar and G. Ochoa, Org. Lett., 2000, 2, 965–968 CrossRef CAS PubMed;
(b) M. Alajarin, P. Sánchez-Andrada, F. P. Cossio, A. Arrieta and B. Lecea, J. Org. Chem., 2001, 66, 8470–8477 CrossRef CAS PubMed;
(c) G. Cainelli, D. Giacomini, M. Gazzano, P. Galleti and A. Quintavalla, Tetrahedron Lett., 2003, 44, 6269–6272 CrossRef CAS;
(d) Z.-Y. Ge, Q.-M. Xu, X.-D. Fei, T. Tang, Y.-M. Zhu and S.-J. Ji, J. Org. Chem., 2013, 78, 4524–4529 CrossRef CAS PubMed;
(e) M. Chen, Z.-H. Ren, Y.-Y. Wang and Z.-H. Guan, Angew. Chem., Int. Ed., 2013, 52, 14196–14199 CrossRef CAS PubMed;
(f) A. D. Clark, W. K. Janowski and R. H. Prager, Tetrahedron, 1999, 55, 3637–3648 CrossRef CAS.
-
(a) L. Hedstrom, A. R. Moorman, J. Dobbs and R. H. Abeles, Biochemistry, 1984, 23, 1753–1759 CrossRef CAS PubMed;
(b) R. L. Jarvest, M. J. Parratt, C. M. Debouck, J. G. Gorniak, J. L. Jennings, H. T. Serafinowska and J. E. Strickler, Bioorg. Med. Chem. Lett., 1996, 6, 2463–2466 CrossRef CAS;
(c) Q. Liu, P. Chen and G. Liu, ACS Catal., 2013, 3, 178–181 CrossRef CAS;
(d) M. Gütschow and U. Neumann, Bioorg. Med. Chem., 1997, 5, 1935–1942 CrossRef.
-
(a) A. M. F. Eissa and R. El-Sayed, J. Heterocycl. Chem., 2006, 43, 1161–1168 CrossRef CAS;
(b) U. Neumann, N. M. Schechter and M. Gütschow, Bioorg. Med. Chem., 2001, 9, 947–954 CrossRef CAS PubMed;
(c) P. Kopelman, A. Bryson, R. Hickling, A. Rissanen, S. Rossner, S. Toubro and P. Valensi, Int. J. Obes., 2006, 31, 494–499 CrossRef PubMed;
(d) M. Baumann and I. R. Baxendale, Beilstein J. Org. Chem., 2013, 9, 2265–2319 CrossRef PubMed;
(e) A. E. Goetz and N. K. Garg, Nat. Chem., 2013, 5, 54–60 CrossRef CAS PubMed.
- ESI†.
- For review, see: K. Afarinkia, V. Vinader, T. D. Nelson and G. H. Posner, Tetrahedron, 1992, 48, 9111–9171 CrossRef CAS.
-
(a) K. Kranjc, B. Štefane, S. Polanc and M. Kočevar, J. Org. Chem., 2004, 69, 3190–3193 CrossRef CAS PubMed;
(b) J. A. Reed, C. L. Schilling Jr, R. F. Tarvin, T. A. Rettig and J. K. Stille, J. Org. Chem., 1969, 34, 2188–2192 CrossRef CAS;
(c) Y. Kuninobu, H. Takata, A. Kawata and K. Takai, Org. Lett., 2008, 10, 3133–3135 CrossRef CAS PubMed;
(d) E. S. Kim, K. H. Kim, S. H. Kim and J. N. Kim, Tetrahedron Lett., 2009, 50, 5098–5101 CrossRef CAS;
(e) C. J. Moody, J. Chem. Soc., Chem. Commun., 1984, 925–926 RSC.
- In the absence of ethyl propiolate 1g′′, treatment of benzonitrile with P(t-Bu)2(o-biphenyl)AuCl/AgSbF6 (5 mol%) in hot DCE (70 °C, 15 h) gave benzamide in 34% yield with 1g′ recovered in 60% yield. A prior hydrolysis of benzonitrile could not be completed for the reaction in eqn (10).
- Only one paper was noted for the [4 + 2]-cycloadditions of common nitriles with non-4π-conjugated species, see, B. A. Bhanu Prasad, A. Bisai and V. K. Singh, Org. Lett., 2004, 6, 4829–4831 CrossRef PubMed.
|
This journal is © The Royal Society of Chemistry 2015 |
Click here to see how this site uses Cookies. View our privacy policy here.