DOI:
10.1039/C5SC01827G
(Edge Article)
Chem. Sci., 2015,
6, 5564-5570
Enantioselective gold-catalyzed intermolecular [2+2] versus [4+2]-cycloadditions of 3-styrylindoles with N-allenamides: observation of interesting substituent effects†
Received
20th May 2015
, Accepted 22nd June 2015
First published on 23rd June 2015
Abstract
A highly enantioselective [2+2] versus a [4+2]-cycloaddition of 3-styrylindoles to N-allenamides catalyzed by identical gold(I)/chiral phosphoramidite complexes is presented, which provides facile access to synthetically valuable, optically active substituted cyclobutanes and tetrahydrocarbazoles. The cycloaddition mode unexpectedly depends on the electronic nature of the N-substituent 3-styrylindoles, the origin of which could be well rationalized using DFT calculations and experimental results. To the best of our knowledge, the present work represents the first example of such an impressive substituent effect in tuning the reaction mode with high chemo-, regio- and enantioselectivity in asymmetric gold catalysis.
Introduction
Over the past decade, gold-catalyzed intermolecular cycloadditions of two unsaturated components have shown their power for rapid access to various cyclic and polycyclic ring systems in an extremely efficient and stereoselective manner.1 In this context, due to their unique reactivity and ease of accessibility, N-allenamides2 have emerged as unique partners for gold-catalyzed [2+2],3 [3+2],4 [4+2],5 and cascade cycloadditions,6 leading to synthetically useful four to seven membered carbocyclic or heterocyclic scaffolds. However, gold-catalyzed cycloadditions still pose challenges with respect to the cycloaddition mode as well as the enantioselectivity,7 only a few elegant examples of enantioselective cycloadditions involving N-allenamides have been reported to date. For example, González and co-workers3e achieved the first asymmetric [2+2]-cycloaddition with alkenes, leading to optically active cyclobutanes8 in good yields. Recently, Mascareñas and López5c explored the elegant asymmetric [4+2]-cycloaddition with 1,3-dienes, leading to cyclohexenes and a cascade cycloaddition with the alkene tethered ketone.6 An efficient asymmetric [3+2]-cycloaddition with nitrones was successfully realized by Chen.4b Very recently, Rossi, Vicente and their co-workers developed a seminal gold(I)-catalyzed intermolecular [4+2]-cycloaddition of 2-vinylindoles with N-allenamides, furnishing good yields of racemic tetrahydrocarbazoles (Scheme 1a).5b However, to the best of our knowledge, a cycloaddition mode which depends on the electronic nature of the substituent is unprecedented in asymmetric gold catalysis. As a part of our continual program in developing asymmetric gold-catalyzed cycloadditions,9 we report the gold catalyzed enantioselective [2+2] and [4+2] cycloadditions of 3-styrylindoles with N-allenamides (Scheme 1b), furnishing synthetically valuable tetrahydrocarbazole10,11 and 3-cyclobutylindole12,13 scaffolds, respectively, which frequently appear in natural products and bioactive compounds (Fig. 1).
 |
| Scheme 1 Previous work and this work. | |
 |
| Fig. 1 Natural products and bioactive compounds containing the tetrahydrocarbazole and 3-cyclobutylindole scaffolds. | |
Results and discussion
3-Styrylindole 1a and N-allenamide 2a were initially chosen as the model substrates to examine a series of chiral ligands (Scheme 2). It was surprising to find that the unexpected [2+2]-cycloadduct 3a, rather than the [4+2]-cycloadduct, was always furnished in excellent yields (>95%) under all of the screened reaction conditions. Using BINOL-derived phosphoramidite ligand (S,S,S)-L1 and its diastereomer (S,R,R)-L1, the reactions could give (+)-3a or its enantiomer (−)-3a with the same enantioselectivity (68% ee). Gratifyingly, the enantioselectivity could be significantly improved by the use of the H8-BINOL-derived phosphoramidite (S,R,R)-L2, furnishing (−)-3a in 90% ee. Notably, (+)-3a was obtained in 76% ee with the application of (R,R,R)-L2 as the chiral ligand. Accordingly, the introduction of a methyl group to the 3- and 3′-positions of the BINOL moiety of L2, denoted as L3, could not increase the enantioselectivity. The changes of the N-substituents in L4 or L5 could not induce a high enantioselectivity either. The BINOL-derived ligand (R,R,R)-L6 with 9-anthracenyl at the 3,3′-positions still could not improve the enantioselectivity. The further variation of other reaction parameters including the solvents, silver salts and concentration did not improve the result, but running the reaction at −60 °C led to the ee increasing to 96%.14 It should be noted that the reaction is easy to handle, and it works well in wet solvents under air without any detriment to the enantioselectivity or yield.
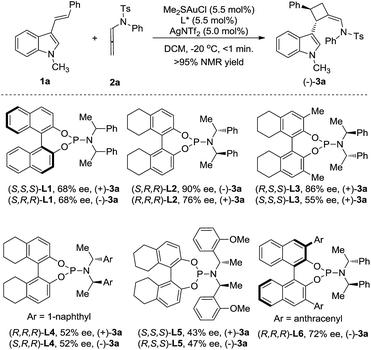 |
| Scheme 2 Investigation of the chiral ligands. | |
With the optimal reaction conditions in hand, a variety of 3-styrylindoles, 1, with different N-protecting groups were prepared and investigated (Table 1). To our delight, those 3-styrylindoles with Bn (1b), allyl (1c) and free H (1d) groups could smoothly undergo the [2+2]-cycloaddition, furnishing the corresponding cycloadducts 3b–3d in 72–92% ee as the single (Z)-stereoisomer (Table 1, entries 2–4). To our surprise, the [4+2]-cycloadducts 4a–4c rather than the [2+2]-cycloadducts were obtained in 67–95% yields with up to 95% ee and 7.3
:
1 Z/E-selectivity with the use of an identical chiral gold catalyst under condition B (DCE, −30 °C, <1 min) when employing 3-styrylindoles 1e–1g with electron-withdrawing N-protecting groups such as CO2Et, Ts and acyl (Table 1, entries 5–7). It should be noted that the [4+2]-cycloaddition takes place with a slightly lower Z/E selectivity but with almost the same enantioselectivity under condition A.
Table 1 The N-substituent effect of 3-styrylindolesa
The scope of the 3-styrylindole for the asymmetric [2+2]-cycloaddition reaction was found to be general (Table 2). For example, the styryl moieties with both electron-rich and electron-deficient aryl groups are compatible, furnishing the desired [2+2]-cycloadducts in 96–99% yield with excellent ee (95–96% ee) (Table 2, entries 1–5). Moreover, different substituents, such as MeO, Me and Br, could be introduced to different positions of the indole moiety and the corresponding [2+2]-cycloadducts could be obtained in 76–99% yield with excellent enantioselectivities (Table 2, entries 6–9); however, the reaction required either a higher catalyst loading (5 mol%) or a much longer reaction time (12 h) for those 3-styrylindoles with electron-donating groups such as MeO and Me (Table 2, entries 6 and 7). In particular, besides styryl, the R1 could be a heteroaryl, such as thienyl (1q), and the reaction also worked very well to give the desired product 3n in 90% yield with 93% ee (Table 2, entry 10). Gratifyingly, the reaction of 1r with an aliphatic R1 group could produce the desired [2+2] cycloadduct 3o in 58% yield with an acceptable 82% ee with the use of (R,R,R)-L6 as the chiral ligand, but the reaction of 1s with a terminal olefin was very messy (Table 2, entries 11–12). Finally, the reaction scope of this asymmetric [2+2]-cycloaddition was evaluated by variation of R2 on the allenamides, 2. The desired products 3q–3r could be produced in close to quantitative yield with 96% ee (Table 2, entries 13–14).
Table 2 Asymmetric [2+2]-cycloaddition

|
Entry |
1, R1, R |
2, R2 |
3
|
Yielda |
ee |
Isolated yield.
Reacting for 30 min.
5 mol% of catalyst.
−50 °C for 10 min, then −20 °C for another 5 min.
(R,R,R)-L6 was used.
The reaction was messy.
|
1 |
1h, 4-BrC6H4/H |
2a, Ph |
3e
|
98% |
96% |
2 |
1i, 4-ClC6H4/H |
2a
|
3f
|
98% |
96% |
3 |
1j, 4-CH3C6H4/H |
2a
|
3g
|
97% |
95% |
4 |
1k, 4-CF3C6H4/H |
2a
|
3h
|
99% |
96% |
5b |
1l, 4-CH3OC6H4/H |
2a
|
3i
|
96% |
95% |
6c |
1m, Ph/5-CH3O |
2a
|
3j
|
76% |
96% |
7c |
1n, Ph/5-CH3 |
2a
|
3k
|
91% |
95% |
8 |
1o, Ph/5-Br |
2a
|
3l
|
99% |
95% |
9 |
1p, Ph/4-Br |
2a
|
3m
|
93% |
95% |
10c,d |
1q, 2-thienyl/H |
2a
|
3n
|
90% |
93% |
11e |
1r, n-Pr/H |
2a
|
3o
|
58% |
82% |
12f |
1s, H/H |
2a
|
3p
|
— |
— |
13 |
1a
|
2b, 4-CH3OC6H4 |
3q
|
98% |
96% |
14 |
1a
|
2c, 4-BrC6H4 |
3r
|
99% |
96% |
In addition to the asymmetric [2+2]-cycloadditions, various substituted N-CO2Et 3-styrylindoles are applicable to [4+2]-cycloadditions, leading to the optically active tetrahydrocarbazoles in good to excellent yields with a Z
:
E selectivity of up to 14
:
1. Gratifyingly, both the Z- and E-isomers could be obtained in high levels of enantioselectivity (88–97% ee). However, substrates 1r′ with aliphatic R1 and 1s′ with a terminal olefin could not give the corresponding [4+2]-cycloadducts, which is attributed to the lower reactivity of the aliphatic alkenylindole (Table 3, entries 11–12).
Table 3 Asymmetric [4+2]-cycloaddition

|
Entry |
1′
, R1, R |
2, R2 |
4
|
Z : E |
Total yield |
ee (%) of Z, E |
1h′ means the substrate is bearing the same substituents at R and R1 as 1h in Table 2 but has a different N-substituent.
The ee could not be determined by chiral HPLC.
Only the dimer of 2a was isolated.
Yield of the isolated (Z)-isomer.
|
1 |
1h′, 4-BrC6H4/H |
2a
|
4d
|
3.5 : 1 |
98% |
97, 90 |
2 |
1i′, 4-ClC6H4/H |
2a
|
4e
|
3.4 : 1 |
95% |
95, 90 |
3 |
1j′, 4-CH3C6H4/H |
2a
|
4f
|
5.6 : 1 |
95% |
96, 90 |
4 |
1k′, 4-CF3C6H4/H |
2a
|
4g
|
3.8 : 1 |
82% |
97, —b |
5 |
1l′, 4-CH3OC6H4/H |
2a
|
4h
|
3.0 : 1 |
88% |
95, —b |
6 |
1m′, Ph/5-CH3O |
2a
|
4i
|
4.7 : 1 |
88% |
97, 92 |
7 |
1n′, Ph/5-CH3 |
2a
|
4j
|
5.0 : 1 |
92% |
96, 88 |
8 |
1o′, Ph/5-Br |
2a
|
4k
|
5.2 : 1 |
97% |
97, 92 |
9 |
1p′, Ph/4-Br |
2a
|
4l
|
6.1 : 1 |
99% |
97, 92 |
10 |
1q′, 2-thienyl/H |
2a
|
4m
|
1 : 1 |
90% |
93, 91 |
11 |
1r′, n-Pr/H |
2a
|
4n
|
— |
0%c |
—, — |
12 |
1s′, H/H |
2a
|
4o
|
— |
0%c |
—, — |
13 |
1e
|
2b
|
4p
|
10 : 1 |
89%d |
90, — |
14 |
1e
|
2c
|
4q
|
14 : 1 |
92%d |
89, — |
To test the practicality of the new methodology, a gram-scale reaction was carried out. To our delight, the catalyst loading could be reduced to only 0.25 mol% on a 4 mmol scale to furnish 1.83 g of 4a in 88% yield with a 5.5
:
1 Z/E ratio and excellent enantioselectivity (Z-4a, 96% ee, and E-4a, 92% ee), indicating that this transformation is easy to scale-up to gram scale without a loss in the efficiency or enantioselectivity (Scheme 3). To showcase the synthetic applications, three transformations of product 4a were carried out. The first transformation was the process involving the selective hydrolysis of the exo enamide moiety and the endocyclic double bond migration of 4a under the catalysis of TsOH at 50 °C, leading to the formation of aldehyde 5a in 66% yield with 96% ee. The second transformation was the selective hydrogenation of the endocyclic double bond of Z-4a at room temperature under the catalysis of 10 mol% Pd/C; the optically active hexahydrocarbazole 6a with three chiral stereocenters could be isolated in 80% yield with 98% ee. The last transformation was the selective endocyclic double bond migration under acidic conditions, producing a new type of tetrahydrocarbazole 7a in 79% yield without the loss of the chiral information. Meanwhile, the exo enamide of 3a could also be hydrogenated at high pressure, and the highly strained cyclobutane 8a with 96% ee could be produced in 95% yield.
 |
| Scheme 3 Gram-scale synthesis and synthetic applications. | |
To rationalize the origin of this dramatic substituent effect on the cycloaddition mode under the catalysis of gold,15 we performed Density Functional Theory (DFT) calculations using the M06 method to calculate the reaction pathway. We presumed that both cycloadditions were stepwise reactions.3–6 The calculated free energy profiles of the cycloaddition reactions of the 3-styrylindole 1a with Int-A at the temperature 298 K are shown in Fig. 2(a). This figure shows the cycloaddition reaction of N-methyl 3-styrylindole 1a and Int A. Fig. 3(a) clearly indicates that the electrostatic potential in the vicinity of the N-CH3 is positive due to the effect of the electron-donating methyl. In contrast, the moiety of the double bond in styryl exhibits a negative electrostatic potential, which tends to interact with positively charged species (ETS-1Me = 1.7 kcal mol−1vs. ETS*-1Me = 6.1 kcal mol−1), leading to a quite stable iminium Int-B with a releasing energy of 10.7 kcal mol−1. For the second reaction step of the ring closure, the barrier of TS-2Me is still lower than the corresponding TS*-2Me by 1.2 kcal mol−1 (ETS-2Me = 9.7 kcal mol−1vs. ETS*-2Me = 10.9 kcal mol−1). In addition, the formation of the [2+2] cycloaddition may be favoured by the stereoelectronic effect in energetics (ΔETS-2Me−TS**-2Me = 4.8 kcal mol−1). Furthermore, Int-C that leads to the [2+2] cycloadduct is more stable than Int*-C by 6.1 kcal mol−1. Moreover, the complete (Z)-selectivity of the [2+2] cycloaddition product can also be easily explained using the computational calculations (please see the details in the ESI†). Thus, the DFT calculations indicate that the (Z)-isomer of the [2+2]-cycloadduct is highly controlled with the N-methyl 3-styrylindole 1a as the substrate and the [2+2] cycloaddition is more favorable than the [4+2] cycloaddition with the N-methyl 3-styrylindole 1a as the substrate.
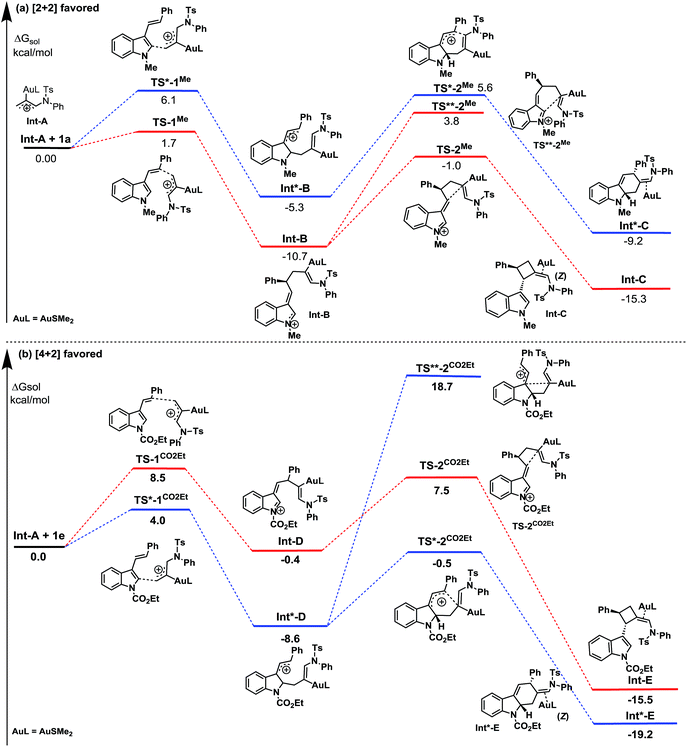 |
| Fig. 2 The free energy profiles ΔGsol for the reaction of 3-styrylindoles 1a and 1e with the Au-allyl cation species Int-A. | |
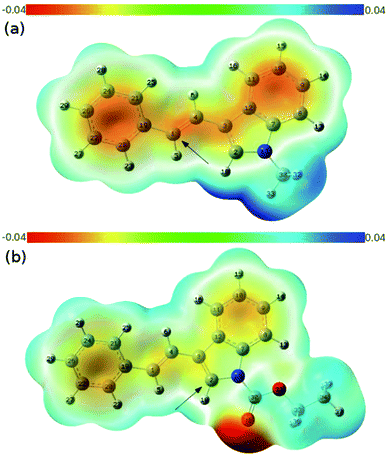 |
| Fig. 3 The calculated contour maps of the electrostatic potentials corresponding to (a) 1a and (b) 1e in Fig. 2, respectively. | |
This is in contrast to when the N-CO2Et 3-styrylindole 1e was used as a substrate (Fig. 2(b)). Fig. 3(b) shows that the calculated electrostatic potential of the N-CO2Et in 1e is completely contrary to that of 1a. Due to the electron-withdrawing effect of N-CO2Et, the vicinity of the oxygen atom of the carbonyl group exhibits a large negative electrostatic potential, which strongly induces an electrostatic interaction with the Au-allyl cation species Int-A. Thus, the first addition of Int-A occurs at the carbonic position of the indole ring in 1e, rather than at the double bond of the styryl, which leads to the intermediate Int*-D (ΔETS*-1CO2Et−TS-1CO2Et = −4.5 kcal mol−1). The barriers of TS*-2CO2Et leading to the [4+2]-cycloadduct and TS-2CO2Et leading to the [2+2]-cycloadduct are very close, with energies of 8.1 and 7.9 kcal mol−1, respectively. By avoiding the drastic steric effects in TS**-2CO2Et, the Int*-D proceeds through the transition state TS*-2CO2Et (ΔETS*-2CO2Et−TS**-2CO2Et = −19.2 kcal mol−1). In addition, the Int*-E is thermodynamically more favorable than Int-E. Moreover, the results in which (Z) and (E)-[4+2] cycloaddition isomers are both obtained, are due to the small steric factor (the exo-double bond of the six-membered ring) and influenced by the substrates,16 catalysts and ligands. In short, the current DFT calculations could well elucidate the chemoselectivity of the 3-styrylindole with different electron-donating and electron-withdrawing groups.
Conclusions
In summary, we have developed a gold(I)-catalyzed highly enantioselective intermolecular [2+2] or [4+2]-cycloaddition of various substituted 3-styrylindoles with N-allenamides. The discrimination between the anticipated [4+2]-product and the unexpected [2+2]-cycloaddition adduct is dependent on the electronic nature of the N-substituent of the 3-styrylindoles, which is well rationalized by DFT calculations. With electron-withdrawing groups, such as CO2Et, Ts and Ac, the [4+2] cycloaddition occurs; in contrast, the [2+2]-cycloadditions take place with electron-donating groups such as methyl, benzyl, allyl and even H groups. The salient features of the present method, including using readily available starting materials and catalysts, high enantioselectivity, good yields, the ease of scale-up to gram scale, and atom-economy, make it give extremely facile access to optically active substituted cyclobutanes and tetrahydrocarbazoles, which are highly valuable building blocks in organic synthesis. Further studies, including the design of a new chiral ligand to address the unsatisfactory enantioselectivity issue of the oxazolidinone-based allenamide,16 are under way in this laboratory and will be reported in due course.
Acknowledgements
We are grateful to the 973 programs (2015CB856600), the National Natural Science Foundation of China (2142520, 21372084), and the Shanghai Eastern Scholar Program for financial supports.
Notes and references
- For selected accounts and general reviews, see:
(a) A. S. K. Hashmi, Chem. Rev., 2007, 107, 3180 CrossRef CAS PubMed;
(b) E. Jiménez-Núñez and A. M. Echavarren, Chem. Rev., 2008, 108, 3326 CrossRef PubMed;
(c) D. J. Gorin, B. D. Sherry and F. D. Toste, Chem. Rev., 2008, 108, 3351 CrossRef CAS PubMed;
(d) S. M. A. Sohel and R. S. Liu, Chem. Soc. Rev., 2009, 38, 2269 RSC;
(e) A. Fürstner, Chem. Soc. Rev., 2009, 38, 3208 RSC;
(f) A. Corma, A. Leyva-Perez and M. J. Sabater, Chem. Rev., 2011, 111, 1657 CrossRef CAS PubMed;
(g) C. Aubert, L. Fensterbank, P. Garcia, M. Malacria and A. Simonneau, Chem. Rev., 2011, 111, 1954 CrossRef CAS PubMed;
(h) N. Krause and C. Winter, Chem. Rev., 2011, 111, 1994 CrossRef CAS PubMed;
(i) M. Bandini, Chem. Soc. Rev., 2011, 40, 1358 RSC;
(j) B.-L. Lu, L. Dai and M. Shi, Chem. Soc. Rev., 2012, 41, 3318 RSC;
(k) D. Garayalde and C. Nevado, ACS Catal., 2012, 2, 1462 CrossRef CAS;
(l) Y.-W. Sun, Q. Xu and M. Shi, Beilstein J. Org. Chem., 2013, 9, 2224 CrossRef PubMed;
(m) F. López and J. L. Mascareñas, Beilstein J. Org. Chem., 2013, 9, 2250 CrossRef PubMed;
(n) G. Cera and M. Bandini, Isr. J. Chem., 2013, 53, 848 CrossRef CAS PubMed;
(o) W. Yang and A. S. K. Hashmi, Chem. Soc. Rev., 2014, 43, 2941 RSC;
(p) F. López and J. L. Mascareñas, J. Chem. Soc. Rev., 2014, 43, 2904 RSC;
(q) C. Obradors and A. M. Echavarren, Acc. Chem. Res., 2014, 47, 902 CrossRef CAS PubMed;
(r) A. S. K. Hashmi, Acc. Chem. Res., 2014, 47, 864 CrossRef CAS PubMed;
(s) L. Zhang, Acc. Chem. Res., 2014, 47, 877 CrossRef CAS PubMed;
(t) F. López and J. L. Mascareñas, Beilstein J. Org. Chem., 2011, 7, 1075 CrossRef PubMed;
(u) D. Qian and J. Zhang, Chem. Rec., 2014, 14, 280 CrossRef CAS PubMed;
(v) D. Qian and J. Zhang, Chem. Soc. Rev., 2015, 44, 677 RSC.
- For general reviews on allenamides, see:
(a) L.-L. Wei, H. Xiong and R. P. Hsuang, Acc. Chem. Res., 2003, 36, 773 CrossRef CAS PubMed;
(b) T. Lu, Z. Lu, Z.-X Ma, Y. Zhang and R. P. Hsung, Chem. Rev., 2013, 113, 4862 CrossRef CAS PubMed.
- For examples of [2+2]-cycloadditions, see:
(a) S. Suárez-Pantiga, C. Hernández-Díaz, M. Piedrafita, E. Rubio and J. M. González, Adv. Synth. Catal., 2012, 354, 1651 CrossRef PubMed;
(b) X.-X. Li, L.-L. Zhu, W. Zhou and Z. Chen, Org. Lett., 2012, 14, 436 CrossRef CAS PubMed;
(c) H. Faustino, P. Bernal, L. Castedo, F. López and J. L. Mascareñas, Adv. Synth. Catal., 2012, 354, 1658 CrossRef CAS PubMed;
(d) P. Bernal-Albert, H. Faustino, A. Gimeno, G. Asensio, J. L. Mascareñas and F. López, Org. Lett., 2014, 16, 6196 CrossRef CAS PubMed. For elegant asymmetric examples, see:
(e) S. Suárez-Pantiga, C. Hernández-Díaz, E. Rubio and J. M. González, Angew. Chem., Int. Ed., 2012, 51, 11552 CrossRef PubMed;
(f) M. Jia, M. Monari, Q. Q. Yang and M. Bandini, Chem. Commun., 2015, 51, 2320 RSC.
- For examples of [3+2]-cycloadditions, see:
(a) W. Zhou, X. X. Li, G. H. Li, Y. Wu and Z. Chen, Chem. Commun., 2013, 49, 3552 RSC. For an example of a highly enantioselective version, see:
(b) G.-H. Li, W. Zhou, X.-X. Li, Q.-W. Bi, Z. Wang, Z.-G. Zhao, W.-X. Hu and Z. Chen, Chem. Commun., 2013, 49, 4770 RSC.
- For examples of [4+2]-cycloadditions, see:
(a) H. Faustino, F. López, L. Castedo and J. L. Mascareñas, Chem. Sci., 2011, 2, 633 RSC;
(b) V. Pirovano, L. Decataldo, E. Rossi and R. Vicente, Chem. Commun., 2013, 49, 3594 RSC. For an impressive asymmetric example, see:
(c) J. Francos, F. Grande-Carmona, H. Faustino, J. Iglesias-Sigüenza, E. Díez, I. Alonso, R. Fernández, J. M. Lassaletta, F. López and J. L. Mascareñas, J. Am. Chem. Soc., 2012, 134, 14322 CrossRef CAS PubMed.
-
(a) H. Faustino, I. Alonso, J. Mascareñas and F. López, Angew. Chem., Int. Ed., 2013, 52, 6526 CrossRef CAS PubMed;
(b) H. Faustino, I. Varela, J. Mascareñas and F. López, Chem. Sci. 10.1039/c5sc00295h.
- For recent reviews or accounts on enantioselective gold-catalysis, see:
(a) R. A. Widenhoefer, Chem.–Eur. J., 2008, 14, 5382 CrossRef CAS PubMed;
(b) N. Bongers and N. Krause, Angew. Chem., Int. Ed., 2008, 47, 2178 CrossRef CAS PubMed;
(c) A. S. K. Hashmi and C. Hubbert, Angew. Chem., Int. Ed., 2010, 49, 1010 CrossRef CAS PubMed;
(d) S. Sengupta and X. Shi, ChemCatChem, 2010, 2, 609 CrossRef CAS PubMed;
(e) M. Rudolph and A. S. K. Hashmi, Chem. Soc. Rev., 2012, 41, 2448 RSC;
(f) Y.-M. Wang, A. D. Lackner and F. D. Toste, Acc. Chem. Res., 2014, 47, 889 CrossRef CAS PubMed. For selected recent examples of enantioselective gold-catalysis:
(g) L. Huang, H.-B. Yang, D.-H. Zhang, Z. Zhang, X.-Y. Tang, Q. Xu and M. Shi, Angew. Chem., Int. Ed., 2013, 52, 6767 CrossRef CAS PubMed;
(h) B. Liu, K.-N. Li, S.-W. Luo, J.-Z. Huang, H. Peng and L.-Z. Gong, J. Am. Chem. Soc., 2013, 135, 3323 CrossRef CAS PubMed;
(i) R. Guo, K.-N. Li, B. Liu, H. -J. Zhu, Y.-M. Fan and L.-Z. Gong, Chem. Commun., 2014, 50, 5451 RSC;
(j) S. D. Lee, J. C. Timmerman and R. A. Widenhoefer, Adv. Synth. Catal., 2014, 356, 3187 CrossRef CAS PubMed;
(k) K. Ji, Z. Zheng, Z. Wang and L. Zhang, Angew. Chem., Int. Ed., 2015, 54, 1245 CrossRef CAS PubMed.
- For selected recent asymmetric syntheses of cyclobutanes, see:
(a) M. R. Luzung, P. Mauleón and F. D. Toste, J. Am. Chem. Soc., 2007, 129, 12402 CrossRef CAS PubMed;
(b) H. Teller, S. Flügge, R. Goddard and A. Fürstner, Angew. Chem., Int. Ed., 2010, 49, 1949 CrossRef CAS PubMed;
(c) A. Z. González, D. Benitez, E. Tkatchouk, W. A. Goddard, III and F. D. Toste, J. Am. Chem. Soc., 2011, 133, 5500 CrossRef PubMed. For recent reviews on racemic [2+2] cycloadditions, see:
(d) B. Alcaide, P. Almendros and C. Aragoncillo, Chem. Soc. Rev., 2010, 39, 783 RSC;
(e) A. Brandi, S. Cicchi, F. Cordero and A. Goti, Chem. Rev., 2014, 114, 7317 CrossRef CAS PubMed. For a nice example of the synthesis of racemic cyclobutanes, see
(f) L. Zhang, J. Am. Chem. Soc., 2005, 127, 16804 CrossRef CAS PubMed.
-
(a) F. Liu, D. Qian, L. Li, X. Zhao and J. Zhang, Angew. Chem., Int. Ed., 2010, 49, 6669 CrossRef CAS PubMed;
(b) G. Zhou, F. Liu and J. Zhang, Chem.–Eur. J., 2011, 17, 3101 CrossRef CAS PubMed;
(c) Z.-M. Zhang, P. Chen, W. Li, Y. Niu, X. Zhao and J. Zhang, Angew. Chem., Int. Ed., 2014, 53, 4350 CrossRef CAS PubMed;
(d) D. Qian and J. Zhang, Chem.–Eur. J., 2013, 19, 6984 CrossRef CAS PubMed;
(e) D. Qian, H. Hu, F. Liu, B. Tang, W. Ye, Y. Wang and J. Zhang, Angew. Chem., Int. Ed., 2014, 53, 13751 CrossRef CAS PubMed;
(f) F. Liu, Y. Wang, W. Ye and J. Zhang, Org. Chem. Front., 2015, 2, 221 RSC.
- For examples of natural compounds with tetrahydrocarbazole scaffolds, see:
(a) M. G. Levkovich, P. K. Yuldashev, M. M. Mirzaeva and B. Tashkhodzhaev, Chem. Nat. Compd., 2012, 48, 440 CrossRef CAS;
(b) J. Bonjoch and D. Solé, Chem. Rev., 2000, 100, 3455 CrossRef CAS PubMed;
(c) A. B. Dounay, L. E. Overman and A. D. Wrobleskiand, J. Am. Chem. Soc., 2005, 127, 10186 CrossRef CAS PubMed;
(d) H. Ishikawa, G. I. Elliott, J. Velcicky, Y. Choi and D. L. Boger, J. Am. Chem. Soc., 2006, 128, 10596 CrossRef CAS PubMed;
(e) A. Nikitenko, D. Evrard, A. L. Sabb, R. L. Vogel, G. Stack, M. Young, M. Lin, B. L. Harrison and J. R. Potoski, Org. Process Res. Dev., 2008, 12, 76 CrossRef CAS;
(f) J. Dai, J. I. Jimenez, M. Kelly and P. G. Williams, J. Org. Chem., 2010, 75, 2399 CrossRef CAS PubMed;
(g) D. Kato, Y. Sasaki and D. L. Boger, J. Am. Chem. Soc., 2010, 132, 3685 CrossRef CAS PubMed;
(h) P. Liu, J. Wang, J. Zhang and F. G. Qiu, Org. Lett., 2011, 13, 6426 CrossRef CAS PubMed;
(i) S. B. Jones, B. Simmons, A. Mastracchio and D. W. C. MacMillan, Nature, 2011, 475, 183 CrossRef CAS PubMed. and references cited therein.
- For selected recent asymmetric syntheses of tetrahydrocarbazoles, see:
(a) X. Han and R. A. Widenhoefer, Org. Lett., 2006, 8, 3801 CrossRef CAS PubMed;
(b) C. Liu and R. A. Widenhoefer, Org. Lett., 2007, 9, 1935 CrossRef CAS PubMed;
(c) C. Gioia, A. Hauville, L. Bernardi, F. Fini and A. Ricci, Angew. Chem., Int. Ed., 2008, 47, 9236 CrossRef CAS PubMed;
(d) H. Huang and R. Peters, Angew. Chem., Int. Ed., 2009, 48, 604 CrossRef CAS PubMed;
(e) M. Bandini and A. Eichholzer, Angew. Chem., Int. Ed., 2009, 48, 9533 CrossRef CAS PubMed;
(f) X. F. Wang, J. R. Chen, Y. J. Cao, H. G. Cheng and W.-J. Xiao, Org. Lett., 2010, 12, 1140 CrossRef CAS PubMed;
(g) B. Tan, G. Hernández-Torres and C. F. Barbas, III, J. Am. Chem. Soc., 2011, 133, 12354 CrossRef CAS PubMed;
(h) S. Müller, M. J. Webber and B. List, J. Am. Chem. Soc., 2011, 133, 18534 CrossRef PubMed;
(i) C. C. J. Loh, G. Raabe and D. Enders, Chem.–Eur. J., 2012, 18, 13250 CrossRef CAS PubMed;
(j) Q. Cai and S. L. You, Org. Lett., 2012, 14, 3040 CrossRef CAS PubMed;
(k) Q.-F. Wu, C. Zheng and S.-L. You, Angew. Chem., Int. Ed., 2012, 51, 1680 CrossRef CAS PubMed;
(l) M. Kawano, T. Kiuchi, S. Negishi, H. Tanaka, T. Hoshikawa, J.-i. Matsuo and H. Ishibashi, Angew. Chem., Int. Ed., 2013, 52, 906 CrossRef CAS PubMed.
- W. R. Gutekunst and P. S. Baran, J. Org. Chem., 2014, 79, 2430 CrossRef CAS PubMed.
- K. S. Jandu, V. Barrett, M. Brockwell, D. Cambridge, D. R. Farrant, C. Foster, H. Giles, R. C. Glen, A. P. Hill, H. Hobbs, A. Honey, G. R. Martin, J. Salmon, D. Smith, P. Woollard and D. L. Selwood, J. Med. Chem., 2001, 44, 681 CrossRef CAS PubMed.
- Please find more screening conditions in the ESI†.
- For selected recent references, which rationalize the mechanism of gold-catalyzed reactions using DFT calculations, see:
(a) W. Yang and A. S. K. Hashmi, Chem. Soc. Rev., 2014, 43, 2941 RSC;
(b) M. H. Vilhelmsen and A. S. K. Hashmi, Chem.–Eur. J., 2014, 20, 1901 CrossRef CAS PubMed;
(c) S. Montserrat, H. Faustino, A. Lledós, J. L. Mascareñas, F. López and G. Ujaque, Chem.–Eur. J., 2013, 19, 15248 CrossRef CAS PubMed;
(d) T. D. Haro, E. Gómez-Bengoa, R. Cribiú, X. Huang and C. Nevado, Chem.–Eur. J., 2012, 18, 6811 CrossRef PubMed;
(e) A. S. K. Hashmi, A. M. Schuster, S. Litters, F. Rominger and M. Pernpointner, Chem.–Eur. J., 2011, 17, 5661 CrossRef CAS PubMed;
(f) I. Alonso, B. Trillo, F. López, S. Montserrat, G. Ujaque, L. Castedo, A. Lledós and J. L. Mascareñas, J. Am. Chem. Soc., 2009, 131, 13020 CrossRef CAS PubMed.
- When using the oxazolidinone-based allenamides as the reacting partner, both cycloadditions could proceed smoothly to afford the corresponding products in excellent yields as a single (Z)-stereoisomer, with low to moderate ee:
.
Footnote |
† Electronic supplementary information (ESI) available: Data for the new compounds, experimental procedures and theoretical studies on the mechanisms. CCDC 1036866 and 1036867. For ESI and crystallographic data in CIF or other electronic format see DOI: 10.1039/c5sc01827g |
|
This journal is © The Royal Society of Chemistry 2015 |