DOI:
10.1039/C5RA17242J
(Paper)
RSC Adv., 2016,
6, 6504-6510
TEMPO-mediated oxidized nanocellulose incorporating with its derivatives of carbon dots for luminescent hybrid films†
Received
26th August 2015
, Accepted 23rd December 2015
First published on 28th December 2015
Abstract
The first use of nanocellulose extracted from bamboo fibers as a fibrous network skeleton and carbon dots derived from nanocellulose as guest fluorescent nanomaterials to construct a transparent, photoluminescent hybrid film is reported. The primary hydroxyls of nanocellulose are firstly converted to the carboxyl form by using tetramethyl-1-piperidinyloxy (TEMPO)-mediated oxidation to enhance the interfacial interaction with carbon dots and then to assemble heterogeneous network architectures through covalent bonding. The carbon dots derived from TEMPO-mediated oxidized nanocellulose display highly uniform spherical morphology with a narrow size distribution ranging from 6 nm to 11 nm. The resultant nanocellulose-based hybrid film has high transparency in bright field imaging and a strong blue luminescence under ultraviolet excitation. Furthermore, the biocompatible hybrid film extracted from the biomass exhibits excellent thermal stability and outstanding mechanical durability, which could be utilized as a substitute for petroleum-based film for diverse applications.
Introduction
With the over-consumption of non-renewable petroleum-based-polymers, transparent films derived from natural biopolymers with the predominant characteristics of excellent biodegradability, biocompatibility and renewability have captured worldwide attention. Cellulose, one of the most abundant renewable biopolymers on earth, has been widely used for preparing highly transparent films with exceptional mechanical robustness and optical transparency.1–3 Nanocellulose (NC) based films have been found to be ideal platforms to host a range of guest functional materials, which offer the potential to supersede traditional plastic films for food packaging, separators, flexible displays, transistors, transparent conductive electrodes and so on.2–9 The NC-based hybrid films with luminescent properties displayed exciting synergetic characteristics and combined NC with luminescent materials, such as fluorescein, rare-earth up-converting luminescent nanoparticles, lanthanide complexes and semiconductor quantum dots.10–12
As one of the most promising fluorescent carbon nanomaterials, carbon dots (CDs) exhibit strong fluorescence, outstanding chemical stability, biocompatibility, aqueous dispersibility and non-toxicity with a favorable size advantage (below 10 nm),13,14 thus, they are regarded as prominent candidates to replace the traditional rare-earth luminescent materials and semiconductor quantum dots in many applications such as sensors, security printing, bio-imaging, biomedicine and optoelectronic devices.15–21 Traditionally, CDs were prepared by using graphites,22,23 carbon nanotubes,24–26 carbon fibers,27 glycerol,28,29 poly(ethylene glycol),30 nanodiamonds,21,31 candle soot18,32 and petroleum coke33 as carbon sources. Recently, a diverse set of cheap, natural and non-toxic, available materials such as winter melon, food waste, plant leaves, potatoes, chitosan, cow's milk and coffee grounds have also been used for the synthesis of photoluminescent CDs.13,34–39 Generally, there are many hydroxyl, carboxyl or amino moieties on the CDs surface, which can be strategically manipulated for further functionalization and assembly with organic, polymeric, inorganic and biological materials.40 NC is an excellent flexible matrix for the construction of diverse shapes of architecture, such as films, fibers and aerogels. In particular, the primary hydroxyls of NC were commonly converted to the carboxyl form by using tetramethyl-1-piperidinyloxy (TEMPO)-mediated oxidation to enhance the interfacial interaction with other matrix components.41,42
In this study, the luminescent NC-based hybrid film was rapidly assembled using well dispersed, TEMPO-mediated oxidized NC (ONC) and its CDs were derived using crosslinking procedures. As far as is known, no research has been reported for preparing CDs using ONC as the starting material. In this paper, the photoluminescent CDs originated from ONC were directly introduced in to the construction of CD/ONC hybrid films based on the reaction of amino groups on the surface of the CDs and the new TEMPO induced carboxyl groups on the surface of the ONC. The flexible CD/ONC hybrid film shows high transparency in bright field imaging and strong luminescence under ultraviolet (UV) excitation. Meanwhile, the hybrid film demonstrates outstanding thermal stability and mechanical durability after bending for 2000 cycles.
Experimental
Materials
Sodium hydroxide (NaOH), trisodium phosphate (Na3PO4), sodium silicate (Na2SiO3), acetic acid (CH3COOH), citric acid, Triton X-100, nitric acid (HNO3), hydrogen peroxide (H2O2), sodium bromide (NaBr), hydrochloric acid (HCl), 2,2,6,6-tetramethyl-1-piperidinyloxy (TEMPO), and sodium hypochlorite (NaClO) were all purchased from Sinopharm Chemical Reagent Co, Ltd. (Shanghai, China), and sodium chlorite (NaClO2) was purchased from Aladdin. 4,7,10-Trioxa-1,13-tridecanediamine (TTDDA) was obtained from Sigma-Aldrich. All the reagents were of analytical grade and used as received without any further purification.
Preparation of NC
NC was obtained according to a modified protocol proposed in the previous work of Cao et al.43 In brief, the bamboo fibers were mechanically smashed and extracted in 3 wt% aqueous NaOH solution at 100 °C for 5 h, followed by filtration and rinsing with deionized water several times. Delignification was performed with 1 wt% NaOH, 0.4 wt% Na3PO4 and 0.4 wt% Na2SiO3 at 115 °C for 2.5 h under mechanical stirring, and the mixture was filtered and washed with deionized water until a neutral pH was obtained. The delignified fibers were firstly bleached with 3.5 wt% NaOH, 0.7 wt% NaClO2, 150 mL CH3COOH at 100 °C for 2 h, followed by filtration and washing with deionized water four times. Subsequently, 0.05 wt% Triton X-100 and 5 wt% of citric acid were added and the mixture was heated at 70 °C for 4 h, then filtered and washed with deionized water until a neutral pH was obtained. Finally, the resulting aggregates were immersed into the mixed 30 wt% H2O2 and 65 wt% nitric acid (HNO3) solution (2
:
1 v/v) at 70 °C for 2 h for further bleaching under vigorous mechanical stirring, followed by centrifugation and thorough washing with deionized water until a neutral pH was obtained.
TEMPO-mediated oxidation of NC (ONC)
TEMPO-mediated oxidation of NC was presented according to the procedure described in the previous work by Lin et al. and Saito et al.44,45 Typically, 100 g of NC suspension (8.2 wt%) was dispersed in 250 mL of deionized water, 0.1090 g TEMPO, 1.0731 g NaBr and 40 mL NaClO (30 wt%) were added with continuous magnetic stirring at room temperature for 20 h. After oxidation, the suspension was centrifuged, washed and then re-dispersed into 100 mL water with the addition of an aqueous solution of HCl (1 mol L−1) under continuous magnetic stirring at ambient temperature for 2 h, followed by centrifugation and washing until a pH of 7 was obtained. The as-prepared ONC was subjected to a high pressure homogenization process for several cycles at a pressure of 50 MPa.
Preparation of CD suspensions
Photoluminescent CDs were synthesized using ONC as the starting material according to a modified process as shown in previous reports by Liu et al.29,46 In a typical procedure, 10 g of ONC (2.15 wt%) mixed with 0.2 mL TTDDA was transferred to a 50 mL Teflon autoclave and heated in a microwave reaction system (MDS-6, Sineo Microwave Chemistry Technology Co. Ltd). The experimental conditions are shown in Table 1. Subsequently, the as-synthesized products were filtered through a 0.22 μm membrane and further dialyzed by using a dialysis bag to obtain the CD suspensions.
Table 1 Experimental parameters of the microwave reaction
Step |
Temperature (°C) |
Time (min) |
Power (W) |
1 |
50 |
2 |
600 |
2 |
100 |
2 |
600 |
3 |
150 |
2 |
600 |
4 |
180 |
2 |
600 |
5 |
200 |
2 |
600 |
Preparation of CD/ONC hybrid films
CD/ONC (2.0 g) in a given ratio was added into 100 mL of deionized water and mixed ultrasonically (high frequency ultrasonic treatment) at 700 W for 10 min to obtain a homogeneous solution. Then the uniform suspension was transferred to an extruder with a nitrogen pressure of 2.0 MPa and by using the pressured extrusion process,10,11 the transparent CD/ONC hybrid films with a thickness of 20 μm and a diameter of 45 mm were finally fabricated and were then dried further in a vacuum oven for 10 min at 95 °C.
Characterization
Transmission electron microscopy (TEM) measurements were performed on a JEM-200CX (Jeol, Japan) at an accelerating voltage of 120 kV. High resolution transmission electron microscopy (HRTEM) measurements were made using a JEM-2010F (Jeol, Japan) at an operating voltage of 200 kV. Scanning electron microscopy (SEM) images were taken using a field emission scanning electron microscope (JSM-6700F, Jeol, Japan), and the samples were metalized with gold under vacuum. Images of the CD/ONC composite films were made using an atomic force microscope (AFM, Dimension 3100, Veeco Instruments, Inc., USA) in tapping mode. The size distribution of the CDs was determined using dynamic light scattering (DLS) analysis on a Zetasizer NANO-ZS90 (Malvern Instruments, UK). X-ray diffraction (XRD) patterns were taken with a D/Max 2200 X-ray diffractometer (Rigaku) equipped with CuKα (λ = 1.5418 Å) radiation operating at 40 kV and 40 mA. The Fourier transform infrared (FT-IR) spectra were recorded using a Thermo Nicolet 6700 spectrometer (Thermo Fisher Scientific, USA) in the spectral range from 4000 to 400 cm−1 using the pressed potassium bromide (KBr) discs prepared by making finely ground samples mixed with dry KBr powder. Ultraviolet-visible (UV-vis) spectra were recorded on a 2500-PC spectrophotometer (Shimadzu) at ambient temperature. A solid-state 13C nuclear magnetic resonance (13C-NMR) spectrometer (Bruker Avance 500 MHz, Bruker Daltonics, Germany) was used to verify the presence of functional groups in the CD/ONC obtained. A thermogravimetric analyzer (STA 409PC, Netzsch, Germany) was used to study the thermal stability of the prepared samples at a heating rate of 10 °C min−1 in the range of 30–1000 °C under an air atmosphere.
Results and discussion
The formation mechanism of the CD/ONC hybrid films is proposed in Scheme 1. The ONC was firstly oxidized and the reaction was TEMPO-mediated and then treated by microwave pyrolysis to form CDs with lots of amino groups on the surface. Thus, transparent CD/ONC hybrid films with blue luminescence upon UV excitation at 365 nm were successfully achieved because of the covalent crosslinking between the carboxyl groups of ONC and the amino groups of the newly-formed CDs. Under bright field imaging, the CD/ONC hybrid film displayed highly optical transparency. The morphology of the ONC, CDs and CD/ONC composites are shown in Fig. 1. It can be clearly seen from the TEM image of ONC in Fig. 1a that the ONC has a dimension of 1–2 μm in length and about 30 nm in width. The well dispersed ONC nanofibrils with numerous hydroxyl groups were beneficial for making the film, especially transparent films.7,10,11 CDs derived from ONC display a highly uniform spherical morphology with a narrow size distribution, the selected detection results are marked with circles and shown in Fig. 1c. The DLS measurement of size distribution of the CDs indicates that the particle size ranges mainly from 6 to 11 nm (Fig. 1d) which agrees with the HRTEM results. It is obvious that CDs conjoin with ONC nanofibrils as shown in Fig. 1b, indicating that CDs were successfully attached on the ONC surface. The quantum yield (QY) is defined as the number of emitted photons relative to the number of absorbed photons, which depends on the synthetic procedure and surface chemistry. In this research, the QY of CDs terminated with amino groups was determined to be 10.27% using quinine sulfate as a reference.
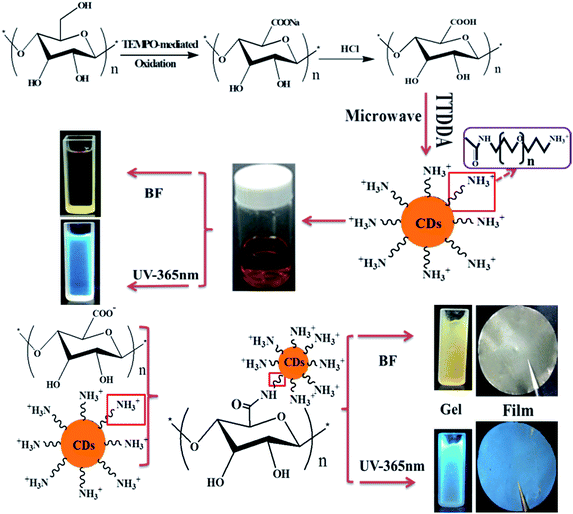 |
| Scheme 1 Schematic illustration of CDs derived from TEMPO-mediated oxidized NC and the resultant CD/ONC hybrid films. | |
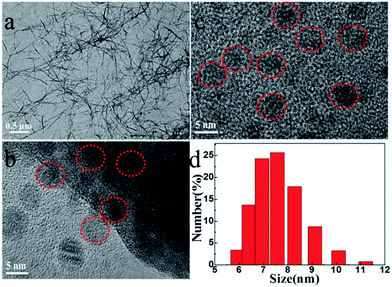 |
| Fig. 1 TEM image of (a) ONC fibrils; HRTEM images of (b) CD/ONC composites and (c) CDs (the detected objects are selected and marked with red circles); (d) size distribution histogram of CDs. | |
After the pressured extrusion of the ONC suspensions and CD/ONC composite suspensions to squeeze out the excess water, a highly transparent ONC film and CD/ONC hybrid film were ultimately fabricated (Fig. 2). As the diameter of ONC is in nanometres, the ONC film and CD/ONC hybrid film display high optical transparency. The school emblem of Shanghai University behind the transparent films is very clearly detected. However, the transmittance of CD/ONC hybrid film slightly decreases with the addition of CDs as shown in UV-vis spectra (Fig. S1, ESI†). SEM images of the surface morphologies of the transparent ONC film and CD/ONC hybrid film are presented in the right-hand columns of Fig. 2. It can be seen that the surface of the ONC film is very smooth, but the flatness of the CD/ONC hybrid film decreases with the combination of CDs into the network of ONC fibrils, the increased surface roughness is also clearly confirmed by the AFM height image (Fig. S2, ESI†).
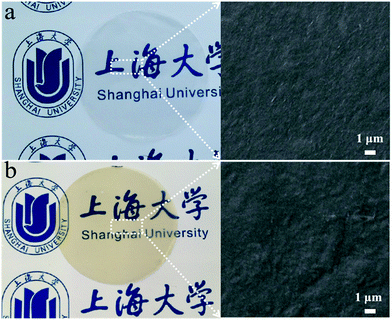 |
| Fig. 2 Digital images (left) and SEM images (right) of ONC film (a) and CD/ONC composite film (b). | |
The phase compositions of the hybrid films were further investigated using XRD analysis. Fig. 3 shows the XRD patterns of NC, ONC and CD/ONC hybrid film. The peaks at 14.7°, 16.5°, 22.7° and 35.0° were attributed to the typical (101), (101), (200), and (040) reflections of cellulose I.47 No significant distinction was observed between NC and ONC, implying that the ONC maintains the typical cellulose I structure after the TEMPO mediated oxidization process. When CDs are attached to the surface of ONC fibrils, an obvious increase could be found in the peak intensity of the XRD patterns of the CD/ONC hybrid film because the CDs give a broad reflection peak centered at 4.3 Å.48
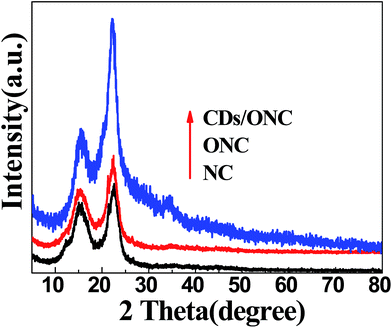 |
| Fig. 3 XRD patterns of NC, ONC film and CD/ONC hybrid film. | |
FT-IR analysis was further used to confirm the combination between CDs and ONC. The FT-IR spectra of NC, ONC and CD/ONC are shown in Fig. 4. The peaks at 3097 and 1559 cm−1 from the FT-IR spectrum of CD/ONC hybrid film are attributed to the N–H vibration, whereas the same signals cannot be observed from FT-IR spectra of ONC and NC.49 The peak at 1736 cm−1 is ascribed to the C
O vibration of carboxyl groups, and the C
ONR vibration at 1644 cm−1 is attributed to the acylation reaction,46,48,50 the formation of C
ONR bonds was also demonstrated at a chemical shift of 170.9 ppm from the 13C-NMR spectrum of CD/ONC hybrid film48 (Fig. S3, ESI†). The peaks at 72.0 ppm (C-2 and C-3), 68.7 ppm and 69.5 ppm (C-5 and C-6), 62.5 ppm (C-6), 30.2 ppm and 37.6 ppm (C-1 of methylene group) were attributed to the six carbon atoms of the ONC unit, respectively.48,51 It is convincingly implied that the acylation reaction between CDs and ONC was clearly verified. The results clearly indicate that CDs were covalently attached on the ONC surface and successfully assembled with ONC to form homogeneous transparent films. The UV-vis absorption and photoluminescence emission spectra were investigated accordingly. As shown in Fig. 5, the as-obtained CDs and CD/ONC hybrid film possess an obvious shoulder peak at about 300 nm in the UV-vis absorption spectra, attributed to the n–π* transition of C
O bonds or other connected groups.52 When excited in the range of 340–400 nm, the CDs exhibit strong blue photoluminescence centered at 456 nm, however, an interesting finding is that the CD/ONC hybrid film presents a slightly stronger blue photoluminescence centered at 460 nm. With the increase of the excitation wavelength to 480 nm, the intensity of the emission of the CDs decreases and the spectra shift from 456 nm to 540 nm.53 However, the luminescent emission intensity for CD/ONC hybrid film is stronger than that of CDs with the increased excitation wavelength (longer than 420 nm). By contrast, no detectable photoluminescence was observed when the pure ONC film was irradiated under the same conditions. It can clearly be seen that the CDs and CD/ONC hybrid film exhibit similar luminescent behaviors, both of them reveal excitation-dependent emissions. The wavelength-dependent behavior may result from the size distribution and surface chemistry of the CDs. Because the CDs and CD/ONC have similar particle sizes, the quantum effects can be excluded from comparison. In fact, the exact photoluminescent mechanism of CDs is currently unresolved and requires further investigation to examine it in depth, it is envisaged that it is associated with passivated surface defects of the core carbon particles. Therefore, it can be deduced that the photoluminescent phenomenon is produced by the numerous functional groups such as amide groups in the CD/ONC surface which introduce plenty of defects as the excitation energy traps and leads to the stronger blue photoluminescence of the CD/ONC hybrid film.54
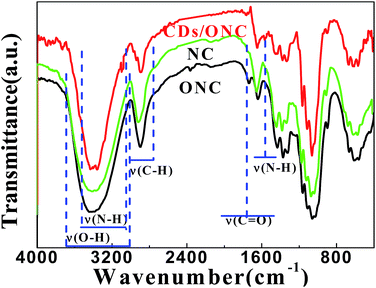 |
| Fig. 4 FT-IR spectra of NC, ONC film and CD/ONC hybrid film. | |
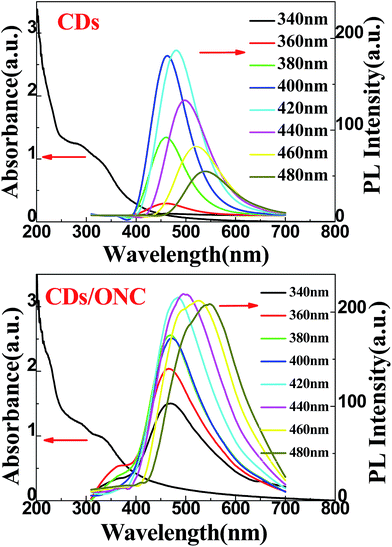 |
| Fig. 5 UV-vis absorption and photoluminescence emission spectra of CDs and CD/ONC hybrid film. | |
Because of the excellent printability, outstanding thermal stability and mechanical flexibility, transparent hybrid films have been demonstrated to be prospective candidates to supersede plastic films for various flexible applications. Fig. 6 shows the photoluminescent emission spectra of CD/ONC hybrid film excited at 360 nm before and after bending at 135° for 500, 1000 and 2000 times. An exciting finding is that the photoluminescent intensity of the hybrid film exhibits no meaningful decrease (less than 5%) after bending for 2000 times (Fig. S4, ESI†). Thermogravimetry (TG) analysis was performed to investigate the thermal stability of CD/ONC hybrid film. Fig. 7 demonstrates the thermal decomposition of NC, ONC film and CD/ONC hybrid film under an air atmosphere. In all the TG curves, the small weight losses below 120 °C apparently result from evaporation of adsorbed water or low molecular weight compounds. The CD/ONC hybrid film exhibits a large weight loss because of the thermal decomposition of NC between 240 °C and 370 °C with 10 wt% residual weights at 1000 °C. Whereas ONC film displays a significant weight loss because of the decomposition of oxygen containing groups in ONC between 120 °C and 390 °C with 3 wt% residual weights at 1000 °C. The results indicate that the incorporation of CDs has a slight effect on the thermal degradation behavior of ONC film, and it can be further concluded that CDs were successfully attached to the ONC surface.44,55
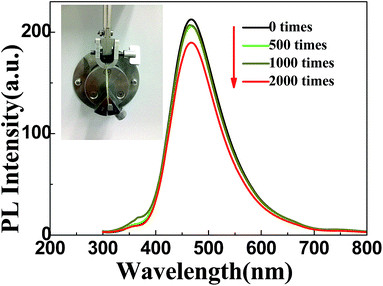 |
| Fig. 6 Photoluminescence emission spectra of CD/ONC hybrid film after bending test (λex = 380 nm). | |
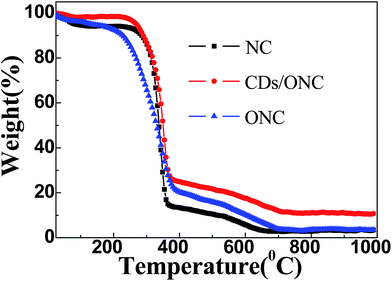 |
| Fig. 7 TG curves of the NC, ONC film and CD/ONC hybrid film under an air atmosphere. | |
Conclusions
In summary, the first use of a TEMPO-mediated oxidized NC as a bioresource to directly synthesize photoluminescent CDs via a typical microwave pyrolysis approach is reported. The ONC fibrils and the derivatives of CDs were covalently crosslinked to construct a transparent CD/ONC hybrid film with blue luminescence by using a pressured extrusion method. The CD/ONC hybrid film presented high transparency, excellent excitation-dependent photoluminescent, behavior, outstanding thermal stability and mechanical flexibility. The PL intensity of the hybrid film exhibits a negligible decrease of less than 5% even after bending for 2000 cycles. In addition, the biocompatible and nontoxic CD/ONC hybrid film should encourage their research and exploration for a multitude of environmentally friendly application areas in future.
Acknowledgements
This work was financially sponsored by Natural Science Foundation of Shanghai (13ZR1415100, 15ZR1415100). We are also grateful to Instrumental Analysis and Research Center of Shanghai University.
Notes and references
- M. Nogi, S. Iwamoto, A. Nakagaito and H. Yano, Adv. Mater., 2009, 21, 1595 CrossRef CAS.
- Z. Fang, H. Zhu, C. Preston, X. Han, Y. Li, S. Lee, X. Chai, G. Chen and L. Hu, J. Mater. Chem. C, 2013, 1, 6191 RSC.
- M. Österberg, J. Vartiainen, J. Lucenius, U. Hippi, J. Seppälä, R. Serimaa and J. Laine, ACS Appl. Mater. Interfaces, 2013, 5, 4640 Search PubMed.
- Y. Jiang, Y. Song, M. Miao, S. Cao, X. Feng, J. Fang and L. Shi, J. Mater. Chem. C, 2015, 3, 6717 RSC.
- F. Jiang, L. Yin, Q. Yu, C. Zhong and J. Zhang, J. Power Sources, 2015, 279, 21 CrossRef CAS.
- J. Huang, H. Zhu, Y. Chen, C. Preston, K. Rohrbach, J. Cumings and L. Hu, ACS Nano, 2013, 7, 2106 CrossRef CAS PubMed.
- H. Koga, T. Saito, T. Kitaoka, M. Nogi, K. Suganuma and A. Isogai, Biomacromolecules, 2013, 14, 1160 CrossRef CAS PubMed.
- H. Koga, M. Nogi, N. Komoda, T. Nge, T. Sugahara and K. Suganuma, NPG Asia Mater., 2014, 6, e93 CrossRef CAS.
- Y. Song, Y. Jiang, L. Shi, S. Cao, X. Feng, M. Miao and J. Fang, Nanoscale, 2015, 7, 13694 RSC.
- J. Zhao, Z. Wei, X. Feng, M. Miao, L. Sun, S. Cao, L. Shi and J. Fang, ACS Appl. Mater. Interfaces, 2014, 6, 14945 CAS.
- M. Miao, J. Zhao, X. Feng, Y. Cao, S. Cao, Y. Zhao, X. Ge, L. Sun, L. Shi and J. Fang, J. Mater. Chem. C, 2015, 3, 2511 RSC.
- J. Xue, F. Song, X. Yin, X. Wang and Y. Wang, ACS Appl. Mater. Interfaces, 2015, 7, 10076 CAS.
- X. Feng, Y. Jiang, J. Zhao, M. Miao, S. Cao, J. Fang and L. Shi, RSC Adv., 2015, 5, 31250 RSC.
- A. Sachdev, I. Matai and P. Gopinath, J. Mater. Chem. B, 2015, 3, 1217 RSC.
- E. Goh, K. Kim, Y. Kim, H. Jung, S. Beack, W. Kong, G. Scarcelli, S. Yun and S. Hahn, Biomacromolecules, 2012, 13, 2554 CrossRef CAS PubMed.
- Q. Zhao, Z. Zhang, B. Huang, J. Peng, M. Zhang and D. Pang, Chem. Commun., 2008, 41, 5116 RSC.
- P. Luo, S. Sahu, S. Yang, S. Sonkar, J. Wang, H. Wang, G. LeCroya, L. Cao and Y. Sun, J. Mater. Chem. B, 2013, 1, 2116 RSC.
- S. Ray, A. Saha, N. Jana and R. Arkar, J. Phys. Chem. C, 2009, 113, 18546 CAS.
- L. Cao, X. Wang, M. Meziani, F. Lu, H. Wang, P. Luo, Y. Lin, B. Harruff, L. Veca, D. Murray, S. Xie and Y. Sun, J. Am. Chem. Soc., 2007, 129, 11318 CrossRef CAS PubMed.
- Y. Dong, N. Zhou, X. Lin, J. Lin, Y. Chi and G. Chen, Chem. Mater., 2010, 22, 5895 CrossRef CAS.
- C. Fu, H. Lee, K. Chen, T. Lim, H. Wu, P. Lin, P. Wei, P. Tsao, H. Chang and W. Fann, Proc. Natl. Acad. Sci. U. S. A., 2007, 104, 727 CrossRef CAS PubMed.
- Y. Sun, B. Zhou, Y. Lin, W. Wang, K. Fernando, P. Pathak, M. Meziani, B. Harruff, X. Wang, H. Wang, P. Luo, H. Yang, M. Kose, B. Chen, L. Veca and S. Xie, J. Am. Chem. Soc., 2006, 128, 7756 CrossRef CAS PubMed.
- H. Li, X. He, Z. Kang, H. Huang, Y. Liu, J. Liu, S. Lian, C. Tsang, X. Yang and S. Lee, Angew. Chem., Int. Ed., 2010, 49, 4430 CrossRef CAS PubMed.
- M. Bottini, C. Balasubramanian, M. Dawson, A. Bergamaschi, S. Bellucci and T. Mustelin, J. Phys. Chem. B, 2006, 110, 831 CrossRef CAS PubMed.
- X. Xu, R. Ray, Y. Gu, H. Ploehn, L. Gearheart, K. Raker and W. Scrivens, J. Am. Chem. Soc., 2004, 126, 12736 CrossRef CAS PubMed.
- J. Zhou, C. Booker, R. Li, X. Zhou, T. Sham, X. Sun and Z. Ding, J. Am. Chem. Soc., 2007, 129, 744 CrossRef CAS PubMed.
- L. Bao, C. Liu, Z. Zhang and D. Pang, Adv. Mater., 2015, 27, 1663 CrossRef CAS PubMed.
- X. Wang, K. Qu, B. Xu, J. Ren and X. Qu, J. Mater. Chem., 2011, 21, 2445 RSC.
- C. Liu, P. Zhang, F. Tian, W. Li, F. Li and W. Liu, J. Mater. Chem., 2011, 21, 13163 RSC.
- A. Jaiswal, S. Ghosh and A. Chattopadhyay, Chem. Commun., 2012, 48, 407 RSC.
- S. Yu, M. Kang, H. Chang, K. Chen and Y. Yu, J. Am. Chem. Soc., 2005, 127, 17604 CrossRef CAS PubMed.
- H. Liu, T. Ye and C. Mao, Angew. Chem., Int. Ed., 2007, 46, 6473 CrossRef CAS PubMed.
- M. Wu, Y. Wang, Y. Wu, C. Hu, X. Wang, J. Zheng, Z. Li, B. Jiang and J. Qiu, Carbon, 2014, 78, 480 CrossRef CAS.
- S. Park, H. Lee, E. Park, S. Lee, J. Lee, S. Jeong, C. Kim, Y. Lee, Y. Huh and J. Lee, ACS Appl. Mater. Interfaces, 2014, 6, 3365 CAS.
- L. Zhu, Y. Yin, C. Wang and S. Chen, J. Mater. Chem. C, 2013, 1, 4925 RSC.
- V. Mehta, S. Jha, R. Singhal and S. Kailasa, New J. Chem., 2014, 38, 6152 RSC.
- X. Gong, W. Lu, M. Paau, Q. Hu, X. Wu, S. Shuang, C. Dong and M. Choi, Anal. Chim. Acta, 2015, 861, 74 CrossRef CAS PubMed.
- L. Wang and H. Zhou, Anal. Chem., 2014, 86, 8902 CrossRef CAS PubMed.
- P. Hsu, Z. Shih, C. Lee and H. Chang, Green Chem., 2012, 14, 917 RSC.
- S. Lim, W. Shen and Z. Gao, Chem. Soc. Rev., 2015, 44, 362 RSC.
- Y. Wang, L. Heim, Y. Xu, G. Buntkowsky and K. Zhang, Adv. Funct. Mater., 2015, 25, 1434 CrossRef CAS.
- H. Orelma, I. Filpponen, L. Johansson, M. Österberg, O. Rojas and J. Laine, Biointerphases, 2012, 7, 61 CrossRef CAS PubMed.
- Y. Cao, Y. Jiang, Y. Song, S. Cao, M. Miao, X. Feng, J. Fang and L. Shi, Carbohydr. Polym., 2015, 131, 152 CrossRef CAS PubMed.
- N. Lin, C. Bruzzese and A. Dufresne, ACS Appl. Mater. Interfaces, 2012, 4, 4948 CAS.
- T. Saito, Y. Nishiyama, J. Putaux, M. Vignon and A. Isogai, Biomacromolecules, 2006, 7, 1687 CrossRef CAS PubMed.
- R. Liu, J. Zhang, M. Gao, Z. Li, J. Chen, D. Wu and P. Liu, RSC Adv., 2015, 5, 4428 RSC.
- Q. Lu, W. Lin, L. Tang, S. Wang, X. Chen and B. Huang, J. Mater. Sci., 2015, 50, 611 CrossRef CAS.
- F. Wang, Z. Xie, H. Zhang, C. Liu and Y. Zhang, Adv. Funct. Mater., 2011, 21, 1027 CrossRef CAS.
- S. Ruan, J. Qian, S. Shen, J. Zhu, X. Jiang, Q. He and H. Gao, Nanoscale, 2014, 6, 10040 RSC.
- M. Bulota, S. Tanpichai, M. Hughes and S. Eichhorn, ACS Appl. Mater. Interfaces, 2012, 4, 331 CAS.
- K. Hattori, E. Abe, T. Yoshida and J. Cuculo, Polym. J., 2004, 36, 123 CrossRef CAS.
- S. Zhu, Y. Song, X. Zhao, J. Shao, J. Zhang and B. Yang, Nano Res., 2015, 8, 355 CrossRef CAS.
- S. Baker and G. Baker, Angew. Chem., Int. Ed., 2010, 49, 6726 CrossRef CAS PubMed.
- Y. Yang, J. Cui, M. Zheng, C. Hu, S. Tan, Y. Xiao, Y. Qu and Y. Liu, Chem. Commun., 2012, 48, 380 RSC.
- J. Cai, S. Kimura, M. Wada and S. Kuga, Biomacromolecules, 2009, 10, 87 CrossRef CAS PubMed.
Footnote |
† Electronic supplementary information (ESI) available: UV-vis spectra of ONC film and CD/ONC hybrid film in Fig. S1; AFM image of CD/ONC hybrid film in Fig. S2; 13C-NMR spectrum of CD/ONC in Fig. S3; photoluminescence emission spectra of the CD/ONC hybrid film after bending for (a) 500, (b) 1000 and (c) 2000 times in Fig. S4. See DOI: 10.1039/c5ra17242j |
|
This journal is © The Royal Society of Chemistry 2016 |