DOI:
10.1039/C5RA15313A
(Paper)
RSC Adv., 2015,
5, 77986-77995
Co-delivery of doxorubicin hydrochloride and verapamil hydrochloride by pH-sensitive polymersomes for the reversal of multidrug resistance†
Received
1st August 2015
, Accepted 9th September 2015
First published on 9th September 2015
Abstract
In this paper, we synthesized the pH-sensitive and biodegradable amphiphilic polypeptide-based block copolymer methoxy-poly(ethylene glycol)2K-poly(ε-caprolactone)4K-poly(glutamic acid)1K (mPEG2K-PCL4K-PGA1K). mPEG2K-PCL4K-PGA1K had low critical aggregation concentration and could self-assemble into polymersomes in aqueous solution revealed by transmission electron microscopy. Therefore, two hydrophilic drug doxorubicin hydrochloride (DOX) and verapamil hydrochloride (VER) were encapsulated into the mPEG2K-PCL4K-PGA1K polymersomes to form poly(DOX + VER) co-delivery system to reverse the multidrug resistance by inhibiting the expression of P-glycoprotein and improve the anti-cancer effect of DOX. The in vitro cytotoxicity experiments indicated the obviously higher inhibition ratio to MCF-7/ADR resistant cells of poly(DOX + VER) compared with that of free DOX solution and polyDOX. The release rate of the two drugs from poly(DOX + VER) were much slower than that from the free drug solutions, and their release behaviors exhibited high pH-sensitive character. Furthermore, the low hemolysis ratio of mPEG2K-PCL4K-PGA1K confirmed that the copolymer could be applied for intravenous injection safely. Therefore, all these findings indicated that the co-delivery of DOX and VER by mPEG2K-PCL4K-PGA1K polymersomes is very promising for cancer therapy.
1. Introduction
These days, serious side effects and multidrug resistance (MDR) have been the two most important issues for chemotherapeutic drugs such as doxorubicin hydrochloride (DOX). DOX is an effective anti-cancer drug and has been used for various tumor treatment on clinic. However, it can induce severe cardiotoxicity because of the lack of target ability,1 which limits the dosage of administration. Though DOX-loaded drug delivery systems with target ability can send DOX into tumor issue to reduce its cardiotoxicity,2 the MDR is still a difficult problem to overcome. DOX is known as the P-glycoprotein (P-gp) substrate3 and it can also active the expression of P-gp.4 The over-expression of P-gp can pump drugs out of the plasma membrane and lower the levels of drugs in the cytoplasm to decrease the anti-tumor effect.5 Verapamil hydrochloride (VER), a kind of calcium channel antagonist, has been applied as antiarrhythmic drugs on clinic. In these years, many studies have shown that VER can also act as P-gp inhibitor to increase the accumulation of anti-cancer drugs within the cancer cells.6,7 It can also reduce the clearance of DOX, resulting in the increased AUC and elongated half-life.8 However, the free VER administration can cause an additive cardiotoxicity.9 While the VER loaded drug delivery system is able to reduce the cardiotoxicity. Therefore, the combination of DOX and VER is desirable for cancer therapy. The combination therapy using drug delivery systems mainly include two ways: (1) one drug-loaded delivery system used with another drug-loaded delivery system; (2) the (drug + drug)-loaded system which is also defined co-delivery system. It has been reported that the cardiotoxicity of VER can be obviated and the anti-cancer effect of DOX can be improved by combining the VER-loaded and DOX-loaded delivery systems (VER and DOX are loaded respectively).10 However, the combination of the respective drug-loaded systems cannot deliver the drugs to the target sites at the same time to obtain the drug ratio as designed for guaranteeing the effective cancer therapy. Therefore, the co-delivery of DOX and VER are studied in our manuscript.
Polymersomes are self-assembled vesicles with large hydrophilic core which can load hydrophilic agents11 such as DOX and VER. They are usually prepared by synthetic biodegradable amphiphilic block copolymers. Compared with liposome8,12 which also possess a hydrophilic core and is prepared by lipid, the polymersomes has a much tougher membrane to guarantee the better stability. So less leakage occurs in polymersomes than that in liposomes. In addition, the polymersomes often show a high drug loading efficiency for hydrophilic drugs compared with other drug delivery formulations.13,14 And the physical and chemical properties of polymersomes including particle size, drug loading content, particle morphology and others can also be tuned by the molecular weight, preparation and et alia for various purposes.15–17 On the other hands, the stable polymersomes may inhibit the release of drugs. Therefore, a stimuli-response polymersomes is desirable for delivering drugs.18,19
Therefore, in the present study we prepared the pH-sensitive polymersomes based on the polypeptide-based mPEG2K-PCL4K-PGA1K we synthesized. MPEG and PCL are selected as hydrophilic block and hydrophobic block respectively for the good biocompatibility and non-cytotoxicity.20–22 PGA, a kind of polypeptide which has been studied broadly for their various stimuli-responsibility23 acts as the pH-sensitive block. Under neutral and basic conditions, the charged PGA is water soluble. At acidic pH, the neutralized PGA shows a great decrease in solubility.24 The decrease of PGA in solubility makes the change of proportions between hydrophilic block and hydrophobic block in the copolymer which can further vary the structure of the aggregate to induce the release of drugs. In addition, it has been confirmed that the pH-sensitive PGA can facilitate the endosomal escape25–27 and guarantee the anticancer effect of drugs. Therefore, the pH-sensitive mPEG2K-PCL4K-PGA1K polymersomes is desired to improve the accumulation of drugs in acidic tumor issue and decrease the concentration of drugs in blood, improving the anti-cancer effect and decreasing the cardiotoxicity of the anticancer drugs.
Based on the theories mentioned above, we prepared the pH-sensitive poly(DOX + VER) co-delivery systems for the reversal of MDR and high anti-cancer effect. The polypeptide-based block copolymer mPEG2K-PCL4K-PGA1K was synthesized by ring-opening polymerization (ROP) and characterized by 1H NMR. And it could assemble into polymersomes in aqueous solution spontaneously with appropriate particle size for targeting tumor issue via enhanced permeability and retention (EPR) effect.28 The hydrophilic drug DOX and VER were physically encapsulated into the polymersomes via the simple dialysis method. The release test in vitro conducted in pH 7.4 and pH 5.0 PBS buffer indicated that the polymersomes co-delivery systems showed a high pH-sensitivity and sustained release ability. The in vitro cytotoxicity and cellular uptake were evaluated in MCF-7 and MCF-7/ADR resistant cells, and the results confirmed that the poly(DOX + VER) could effectively reverse MDR and improve the anti-cancer effects significantly.
2. Research methods
2.1 Materials
Methoxy-poly(ethylene glycol) (mPEG2K) was purchased from Sigma-Aldrich; ε-caprolactone, stannous octoate, dicyclohexylcarbodiimide (DCC), 4-dimethylamiopyridine (DMAP), N-(tert-butoxycarbonyl)-L-phenylalanine (Phe-NBOC), trifluoroacetic acid (TFA), trifluoromethane-sulfonic acid (TFMSA), thioanisole were purchased from Aladdin; γ-benzyl-L-glutamate N-carboxyanhydride (NCA-BLG) was supplied by Chengdu Enlai Biological Technology Co., Ltd; doxorubicin hydrochloride and verapamil hydrochloride were purchased from Dalian Meilun Biotech Co., Ltd. 3-(4,5-Dimethylthiazol-2-yl)-2,5-diphenyltetrazoliumbromide (MTT) was purchased from Sigma-Aldrich (China). RPMI-1640 medium, fetal bovine serum (FBS) and phosphate buffer solution (PBS) were purchased from Gibco BRL (Gaithersberg, MD, USA). MCF-7 and MCF-7/ADR resistant cells were kindly donated by the Department of Pharmacology, School of Pharmacy, Shandong University. All the materials were used as received except that ε-caprolactone was purified by vacuum distillation over CaH2 (purchased from Aladdin).
2.2 Synthesis of polypeptide-based copolymer mPEG2K-PCL4K-PGA1K
The synthesis route of mPEG2K-PCL4K-PGA1K is shown in Scheme 1 (the details of chemical synthesis and the determination of molecular weight are described in the ESI†). The first step was to synthesize mPEG2K-PCL4K-OH via ROP of ε-caprolactone using mPEG2K as initiator and stannous octoate as a catalyst.29 The OH end-group of mPEG-PCL–OH was then reacted with Phe-NBOC under the catalysis of DCC and DMAP to synthesize mPEG2K-PCL4K-Phe-NBOC. To obtain mPEG2K-PCL4K-NH2, the BOC group was removed by the acid hydrolysis of TFA. The NCA-BLG was also reacted with the NH2 end-group of mPEG2K-PCL4K-NH2 through ROP reaction to form the mPEG2K-PCL4K-PBLG1K.23 Finally, the benzyl protecting group was removed and the desired product mPEG2K-PCL4K-PGA1K was obtained.
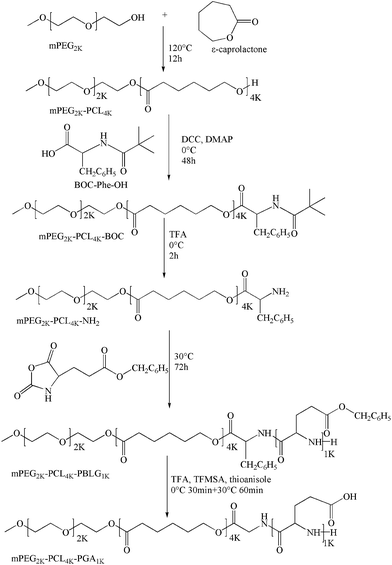 |
| Scheme 1 The synthesis route of mPEG2K-PCL4K-PGA1K. | |
2.3 Characterizations of the synthesized mPEG2K-PCL4K-PGA1K
2.3.1 Nuclear magnetic resonance analysis (1H NMR). To verify the successful synthesis of copolymers mPEG2K-PCL4K-PGA1K, the 1H NMR spectra of the copolymer (in DMSO-D6) were recorded on a Bruker AV400 NMR spectrometer (Bruker, German) at 400 MHz.
2.3.2 Determination of CAC. To determine the critical aggregation concentration (CAC) of the synthesized mPEG2K-PCL4K-PGA1K, the fluorescence techniques was applied using pyrene as probe molecules as we have reported previously.30 Briefly, 40 μL stock pyrene solution (6 × 10−4 mol L−1 in ethanol) was transferred to a series of 10 mL tubes. After the ethanol was evaporated, the desired amount of mPEG2K-PCL4K-PGA1K solution in water was added to tubes to make the final pyrene concentration be 6 × 10−6 mol L−1 and the mPEG2K-PCL4K-PGA1K range from 0.001 to 20 mg mL−1. Then all the tubes were equilibrated with ultrasonication for 1 h and stood overnight to make the pyrene molecules solubilize in the aggregates. After setting the excitation wavelength at 334 nm, the fluorescence spectra of the samples were recorded from 350 to 450 nm with Hitachi F-7000 fluorescence spectrophotometer and the slit width was set at 2.5 nm for excitation and emission. After calculating the ratio of fluorescence intensities at 373 nm (denoted as I1) and 384 nm (denoted as I3), the CAC was determined using the plot of pyrene I1/I3 ratio versus different concentration of copolymers.
2.3.3 Morphology of mPEG2K-PCL4K-PGA1K in water. mPEG2K-PCL4K-PGA1K could spontaneously assemble in aqueous solution and the morphology of the aggregates was confirmed by transmission electron microscopy (TEM). Firstly, drops of sample were adsorbed onto the carbon-coated copper grid separately and the redundant sample was removed with filter paper. After dried, the carbon-coated copper grids with samples were observed using a JEOL JEM1400 transmission electron microscope.
2.4 Preparation of drug-loaded polymersomes
The poly(DOX + VER) were prepared via the dialysis method as follows.31 Briefly, 10 mg mPEG2K-PCL4K-PGA1K was dissolved in 0.2 mL DMSO, and then 0.3 mL of aqueous solution containing 1.5 mg VER was added dropwise. Because the residual of acids like TFA and TFMSA introduced in the last synthesis step, appropriate amount of NaOH (1 M) was added to tune the pH of the solution to be neutral. After this, 0.3 mL of water solution containing 1 mg DOX was added and the solution was sonicated for about 1 min to accelerate the entrapment of drugs. Finally, the poly(DOX + VER) was dialysed against deionized water (400 mL) in a dialysis bag with a molecular weight cut-off of 3500 Da for 4 h to remove the free drugs, and the deionized water was changed once. Therefore, there is nearly no DMSO molecules existed in the sample.
For preparing DOX-loaded polymersomes (denoted as polyDOX), the procedures were similar with poly(DOX + VER) preparation except that the VER water solution was replaced by water.
The loading capacity of polymersomes was determined by measuring the amount of DOX and VER in poly(DOX + VER) using high performance liquid chromatography (HPLC). The poly(DOX + VER) was destroyed by DMSO and diluted by mobile phase, and then the sample was filtered through a 0.45 μm filter and eluted through a Phenomenex gemini C18 column (5 μm, 250 mm × 4.6 mm, USA). The conditions for HPLC analysis of DOX is listed below: the detection wavelength was set at 266 nm, the mobile phase consists of methanol
:
0.01 M NH4H2PO4
:
acetic acid (65
:
35
:
0.2, v/v/v) and the flow rate was set as 0.7 mL min−1. As for the other drug VER, the detection wavelength was set at 278 nm, the mobile phase consisting of acetic acid–acetic natrium
:
methanol
:
three-ethylamine (45
:
55
:
1, v/v/v) was delivered at a flow rate of 0.8 mL min−1. Concentration was determined from the calibration curve of drugs in the mixture of DMSO and mobile phase. Drug-loading efficiency (DL) and encapsulation efficiency (EE) were calculated as following:
In order to get high EE and DL for both drugs, various amounts of drugs have been encapsulated into 10 mg mPEG2K-PCL4K-PGA1K (Table S1, ESI†). The results showed that when the ratio of drugs and copolymer was 1
:
1.5
:
10 (DOX
:
VER
:
mPEG2K-PCL4K-PGA1K), the average DL and EE of the two drugs was high. At the selected ratio, the DL and EE of DOX was (5.05 ± 0.26)% and (59.89 ± 3.40)% while that of VER was (10.72 ± 1.08)% and (84.82 ± 9.53)%, respectively.
2.5 Size and size distribution of drug-loaded polymersomes
Dynamic light scattering (DLS) was carried out to determine the size and size distribution of drug-loaded polymersomes using BIC-Brook-Haven (USA) with angle detection at 90°. Before the measurements, all the samples including polyDOX and poly(DOX + VER) were filtered through a 0.45 μm membrane filter to prepare the dust-free solutions.
2.6 Drug release in vitro
To verify the sustained and pH-sensitive release of drugs from drug-loaded polymersomes, polyDOX and poly(DOX + VER) which containing the same content of drug were incubated in the dialysis membrane bag with molecular weight cut off of 3500 Da, respectively. Then the dialysis membrane bags were immersed in 20 mL of 0.01 M PBS buffers (pH 7.4 and 5.0) at 37 °C with continuously stirring at 100 rpm. At the designated time intervals, 0.5 mL of the samples was withdrawn from the release medium and the same amount of fresh release medium was added immediately. For comparison, the release profile of free drugs DOX and VER were also tested in pH 7.4 and 5.0 PBS. The release solution was filtered through a 0.45 μm membrane filter which was then used to measure the amount of released DOX and VER by HPLC. The concentration was determined from the calibration curve of DOX and VER in PBS (pH 7.4 and 5, respectively) and the cumulative amount of drugs was calculated with the following equation:
Mt refers to the amount of the released drug from drug-loaded polymersomes at time t and Mtotal refers to the total amount of drugs loaded in drug-loaded polymersomes.
2.7 Cytotoxicity assay in vitro
The in vitro cytotoxicity of free DOX, polyDOX and poly(DOX + VER) to MCF-7 and MCF-7/ADR resistant cells were determined by MTT method.32 Briefly, MCF-7 and MCF-7/ADR resistant cells were transferred to 96-well tissue culture plates at a seeding density of 5000 cells per well (0.1 mL medium). Following by attachment overnight, the culture medium in each well was carefully replaced with medium containing serial dilutions of treatment drugs including free DOX, polyDOX and poly(DOX + VER). The concentrations of DOX were ranging from 0.01 μg mL−1 to 20 μg mL−1 (0.01 μg mL−1, 0.1 μg mL−1, 1 μg mL−1, 10 μg mL−1 and 20 μg mL−1). At scheduled time intervals (24 h, 48 h and 72 h), 10 μL of 5 mg mL−1 MTT dissolved in PBS was added to each well and the plates were incubated for 4 h at 37 °C. After removing the medium, 150 μL of DMSO was added to each well to dissolve the formed purple crystals derived from MTT with vigorously stirring the plates. The absorbance of each well was read on a microplate reader (Enspire instruments, Perkin Elmer, America) at a wavelength of 490 nm.
In the study, untreated cells served as control and were taken as 100% viability and all the samples were performed in triplicate to give the average and standard deviation (SD). Based on the absorbance of each well, the percentage of cell growth inhibition was calculated as follows: inhibitory rate = (Acontrol − Asample)/(Acontrol − Ablank) × 100%. Acontrol and Ablank referred to the absorbance of the culture medium in the presence and absence of cells; Asample referred to the absorbance of the cells respectively treated with free DOX, polyDOX and poly(DOX + VER).
2.8 Cellular uptake and flow cytometric analysis
The cellular uptake of free DOX and drug-loaded polymersomes including polyDOX and poly(DOX + VER) was tested using inverted fluorescence microscope. Since DOX showed the red color and could act as a fluorescent probe, all the samples were tested directly without using another fluorescence molecule.33 MCF-7 and MCF-7/ADR resistant cells were seeded into 6-well culture plates at a density of 2 × 105 cells per well and incubated overnight. The cells were then respectively treated with free DOX, polyDOX or poly(DOX + VER) at a final DOX concentration of 3 μg mL−1 and allowed to be incubated for 2 h. After washed three times with PBS, all the samples were imaged using inverted fluorescence microscope.
To determine the cellular uptake of free DOX, polyDOX or poly(DOX + VER) quantitatively, the fluorescence intensity in the cells treated with different samples was tested using flow cytometry. The cells treated as described above were also seeded into 6-well culture plates at a density of 2 × 105 cells per well and incubated overnight. After being incubated for 2 h and harvested by trypsinization with centrifugation, the cells were suspended in 200 μL of PBS. Finally, the fluorescence intensity in the cells was determined using a flow cytometer. The number of cells collected was ten thousand, and the experiments were run in triplicate.
2.9 Hemolysis test
The hemoglobin released from rabbit blood was used to evaluate the hemolytic activities of blank polymersomes by spectrophotometry. Whole rabbit blood samples were centrifugated and resuspended in normal saline to get the red blood cells (RBCs 2%). 1.25 mL RBCs suspension mixed with 1.25 mL normal saline solution and 1.25 mL distilled water were served as negative control (producing no hemolysis) and positive control (producing 100% hemolysis), respectively. 0.15 mL of blank polymersomes solution with different concentration (0.014 mg mL−1, 0.07 mg mL−1, 0.14 mg mL−1, 0.7 mg mL−1 and 1.4 mg mL−1) were added into the mixture of 1.25 mL RBCs suspension and 1.1 mL normal saline solution. After kept at (37.0 ± 1.0) °C for 3 h, all the samples were centrifuged at 1500 rpm for 15 minutes. The absorbance of supernatants was measured with UV spectrophotometer at 540 nm and the normal saline was used as blank.
The hemolysis ratio of RBSs was calculated using the following formula: hemolysis (%) = (Asample − Anegative)/(Apositive − Anegative) × 100%, where Asample, Anegative, Apositive refer to the absorption of blank polymersomes, negative control and positive control at 540 nm, respectively.
3. Results and discussion
3.1 Characterization of the synthesized mPEG2K-PCL4K-PGA1K
3.1.1 Nuclear magnetic resonance analysis (1H NMR). The characterization of mPEG2K-PCL4K-PGA1K was shown in Fig. 1. The location of the peaks and assignment of the letters shown in 1H NMR spectra were listed as below: mPEG: (a) (δ 3.32) CH3OCH2CH2O, (b) (δ 3.51) CH3OCH2CH2O; PCL: (c) (δ 2.26) COCH2CH2CH2CH2CH2O, (d) (δ 1.54) COCH2CH2CH2CH2CH2O, (e) (δ 1.29) COCH2CH2CH2CH2CH2O, (f) (δ 3.97) COCH2CH2CH2CH2CH2O; BOC-Phe-OH: (g) (δ 7.26) COCH(CH2C6H5)NH; PBLG: (g) (δ 7.26)COCH(CH2CH2COOCH2C6H5)NH, (h) (δ 5.06) COCH(CH2CH2COOCH2C6H5)NH.
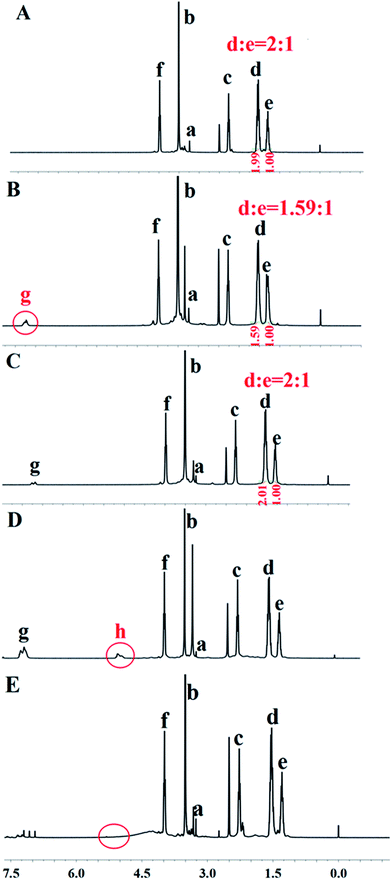 |
| Fig. 1 The 1H NMR spectra of (A) mPEG2K-PCL4K-OH (B) mPEG2K-PCL4K-BOC (C) mPEG2K-PCL4K-NH2 (D) mPEG2K-PCL4K-PBLG1K (E) mPEG2K-PCL4K-PGA1K in DMSO-D6. | |
The Fig. 1A was the spectrum of mPEG2K-PCL4K-OH. Based on the comparison of the peak areas at 3.51 ppm (b) and 2.26 ppm (c),34 the molecular weight of PCL was estimated to be 4K. Due to the fact that the peak of COC(CH3)3 in BOC-Phe-OH was overlapped with the peak of H labeled with “e” in the PCL block at 1.29 ppm, the ratio of peak at 1.54 ppm (d) and 1.29 ppm (e) decreased from 2
:
1 to 1.59
:
1 confirmed the successful conjugation of BOC-Phe-OH which was also proved by the appearance of the peak at 7.26 ppm (g).35 After removing the BOC group to get mPEG2K-PCL4K-NH2 by acidolysis, the ratio of the peak at 1.54 ppm (d) and 1.29 ppm (e) got back to 2
:
1. To back up the successful synthesis of mPEG2K-PCL4K-PBLG1K, the representative peak of COCH(CH2CH2COOCH2C6H5)NH was show at 5.06 ppm (h). Based on the comparison of the peak areas at 5.06 ppm (h) and 2.26 ppm (c), the molecular weight of PBLG was estimated to be 1K. In the last step, the disappearance of the peak at 5.06 ppm (h) confirmed that the benzyl group of PBLG was removed and the target product mPEG2K-PCL4K-PGA1K was synthesized successfully.
3.1.2 Determination of CAC. Pyrene was the most widely used fluorescent molecule for determining the CAC and its fluorescence spectrum was shown Fig. S1 (ESI†). The I1/I3 ration of pyrene emission spectra was related with the polarity where the pyrene located.36 The I1/I3 ratio of fluorescence emission spectra of pyrene versus concentration of mPEG2K-PCL4K-PGA1K was shown in Fig. 2. It could be seen that at the beginning I1/I3 ratio slightly decreased and then abruptly decreased with the increase of copolymer concentration. From the break point the CAC of the copolymer could be determined about 0.0776 mg mL−1 (1.1086 × 10−5 mol L−1). At the beginning, the pyrene molecules was in the polar environment (water), with the polymer concentration increasing, there was aggregates formed in the solution and the pyrene could be solubilized in the hydrophobic domain of the aggregates, leading to the decrease of I1/I3 ratio. The low CAC of mPEG2K-PCL4K-PGA1K guarantee the stability of the polymersomes diluted in the vivo.
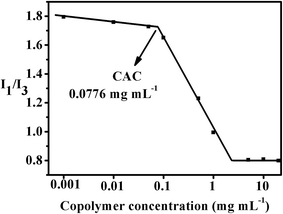 |
| Fig. 2 The I1/I3 ratio of fluorescence emission spectra of pyrene versus concentration of mPEG2K-PCL4K-PGA1K. | |
3.1.3 Morphology of mPEG2K-PCL4K-PGA1K in water. The copolymer mPEG2K-PCL4K-PGA1K could self-assemble in water and the solution was blue-opalescent (Fig. 3A) and the morphology of the aggregates was examined by TEM images (Fig. 3B and S2 in ESI†). It could be seen that the uniform spherical aggregates with the diameter about 50–60 nm were formed in the solution. From Fig. 3B and S2† it could be clearly seen that the polymer assembly were hollow spheres containing an aqueous solution in the core surrounded by a bilayered membrane. A characteristic dark rim on the outer surface was an obvious indication of the hollow morphology of polymersomes.37 Therefore, they could be applied to deliver hydrophilic drugs efficiently. The appropriate size of polymersomes facilitates the EPR effect for passive targeting for the tumor issue.
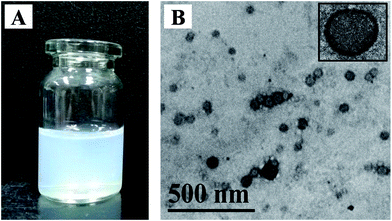 |
| Fig. 3 (A) Photograph of mPEG2K-PCL4K-PGA1K aqueous solution. (B) Morphology of mPEG2K-PCL4K-PGA1K self-assembly in water. Insert: the TEM blowup of a single polymersome. | |
3.2 Size and size distribution of drug-loaded polymersomes
The size and size distribution of drug-loaded polymersomes were characterized by DLS. As shown in Fig. 4, both polyDOX and poly(DOX + VER) showed a unimodal and narrow size distribution (PDI: 0.04–0.09), and the diameter were (58.8 ± 0.3) nm and (44.9 ± 0.5) nm, respectively. The size of the drug-loaded polymersomes was appropriate for EPR effect to achieve passive targeting.
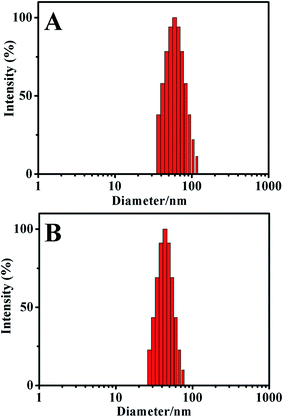 |
| Fig. 4 The size and size distribution of drug-loaded polymersomes (A) polyDOX and (B) poly(DOX + VER). | |
3.3 Drug release in vitro
The drug release profiles in PBS with different pH value were shown in Fig. 5. It could be seen from Fig. 5A that firstly the release of DOX from polyDOX and poly(DOX + VER) was more sustained than that from the free DOX solution. For example, free DOX released almost completely in 24 h while polyDOX and poly(DOX + VER) only released 19.03% and 18.94% in pH 7.4 PBS. Secondly, the addition of VER has no effect on the release of DOX from the polymersomes, which was proved by the almost same release plots for the polyDOX and poly(DOX + VER) in pH 7.4 and pH 5.0 PBS. Thirdly, the drug-loaded polymersomes showed the pH-sensitive release behavior and the release of DOX from polyDOX and poly(DOX + VER) was higher in pH 5.0 PBS than that in pH 7.4 PBS. Though the low pH could facilitate the solubility and accelerate the release of DOX, the faster release rate of DOX from polyDOX and poly(DOX + VER) at pH 5.0 than that at 7.4 was mainly due to the structure breakage of the polypeptide-based polymersomes in acid environment. Conclusively, the prepared polymersomes showed good pH-sensitive property and the obvious sustained release behaviour, which was desirable for the efficient cancer therapy.
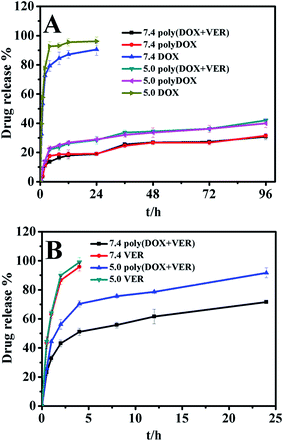 |
| Fig. 5 The in vitro release profiles of (A) DOX and (B) VER from polymersomes in different PBS (pH 7.4 and 5.0). The release profiles of free DOX and VER solutions were investigated as control. Data as mean ± SD, n = 3. | |
Fig. 5B showed VER release behaviour from the poly(DOX + VER). Similarly, VER also showed a sustained and pH-sensitive release from poly(DOX + VER). The released VER amounts were about 91.74% and 71.63% from the poly(DOX + VER) in pH 5.0 and pH 7.4 PBS after 24 h, respectively. The sustained-release time of VER from poly(DOX + VER) was not as long as that of DOX was mostly due to the strong electrostatic interactions between DOX and PGA.38
Based on the above results, the copolymer mPEG2K-PCL4K-PGA1K could act as a promising drug carrier with high pH-sensitive property. Thus the drug-loaded polymersomes could accelerate the drug release and improve the accumulation of drugs in acidic tumor issue to enhance the anti-cancer effect. In addition, the sustained release of drugs from the polymersomes could make the drug constantly fight against cancer cells inducing the increased cancer cells inhibition.
3.4 Cytotoxicity assay in vitro
To verify the antitumor effect of the drug-loaded polymersomes, the cell inhibition of the blank polymersomes was tested with the concentration of copolymer ranging from 0.1 to 500 μg mL−1. The blank polymersomes had no apparent cytotoxicity on cancer cells as shown in Fig. S3 (ESI†). The in vitro antitumor activity of drug-loaded polymersomes was tested and that of free DOX was used as control. As illustrated in Fig. 6, the inhibition rate of the samples increased with an increasing drug concentration from 0.01 μg mL−1 to 20 μg mL−1 for both MCF-7 and MCF-7/ADR cells. For the MCF-7 cells, free DOX sample showed a little higher inhibition ratio than that of polyDOX or poly(DOX + VER) sample due to the incomplete release of DOX from drug-loaded polymersomes which was confirmed from Fig. 5A. The polyDOX and poly(DOX + VER) exhibited similar inhibition ratio, which indicated that the VER had almost no cytotoxicity to cancer cells.39 However, for the MCF-7/ADR cells, the inhibitory effect of poly(DOX + VER) was much stronger than that of free DOX or polyDOX especially at 48 h and 72 h, indicating that the addition of P-gp inhibitor VER could significantly improve the cytotoxicity of DOX to cancer resistant cells.
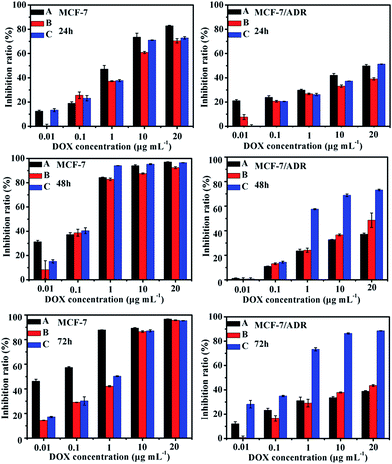 |
| Fig. 6 The inhibition ratio to MCF-7 and MCF-7/ADR cells incubated with different samples (A) DOX (B) polyDOX and (C) poly(DOX + VER). | |
In order to further confirm the enhanced cytotoxicity and resistant ability of poly(DOX + VER) against MCF-7/ADR cells, the IC50 value and resistance reversion index (RRI) were calculated and listed in Table 1. The IC50 values of poly(DOX + VER) were 10.14 ± 3.02 μg mL−1, 2.71 ± 0.35 μg mL−1 and 0.15 ± 0.02 μg mL−1 respectively at 24, 48 and 72 h, much lower than that of free DOX (55.46 ± 3.73 μg mL−1 for 24 h, 31.85 ± 6.82 μg mL−1 for 48 h and 7.32 ± 0.94 μg mL−1 for 72 h). The difference in IC50 values indicated that poly(DOX + VER) has a much higher cytotoxicity in MCF-7/ADR cells than free DOX. RRI was an important parameter for evaluating the reversal activity of MDR reversal agents. At 24 h, 48 h and 72 h, the RRIs of poly(DOX + VER) were 5.47, 11.75 and 48.8, respectively, indicating the poly(DOX + VER) could reverse the resistance of MCF-7/ADR cells to DOX in 72 h successfully. Therefore, the poly(DOX + VER) had high ability to inhibit the proliferation of MCF-7/ADR cells.
Table 1 IC50 (mean ± SD, n = 3) and RRI of poly(DOX + VER) against MCF-7/ADR cellsa
|
IC50 (μg mL−1) |
RRI |
24 h |
48 h |
72 h |
24 h |
48 h |
72 h |
RRI = IC50(free DOX)/IC50(poly(DOX + VER)). |
DOX |
55.46 ± 3.73 |
31.85 ± 6.82 |
7.32 ± 0.94 |
— |
— |
— |
Poly(DOX + VER) |
10.14 ± 3.02 |
2.71 ± 0.35 |
0.15 ± 0.02 |
5.47 |
11.75 |
48.8 |
3.5 Cellular uptake studies and flow cytometric analysis
The cellular uptake of free DOX, polyDOX and poly(DOX + VER) on MCF-7 and MCF-7/ADR cells were visualized quantitatively by inverted fluorescence microscope. As illustrated in Fig. 7, detectable DOX red fluorescence was present in these cancer cells. It could be seen that the red fluorescence intensity of free DOX, polyDOX and poly(DOX + VER) on MCF-7 cells almost showed no difference. This is because that the free DOX could readily diffuse across the cell membrane to induce high cellular uptake.40 Since DOX could be easily pumped out by P-gp in MCF-7/ADR cells membranes,41 an obvious disparity in red fluorescence intensity of free DOX, polyDOX and poly(DOX + VER) has been revealed on MCF-7/ADR cells: the red fluorescence intensity of poly(DOX + VER) was higher than that of polyDOX or free DOX. All these indicated that mPEG2K-PCL4K-PGA1K could deliver drugs into cancer cells successfully, and poly(DOX + VER) could inhibit the expression of P-gp and improve the accumulation of DOX in MCF-7/ADR cells significantly.
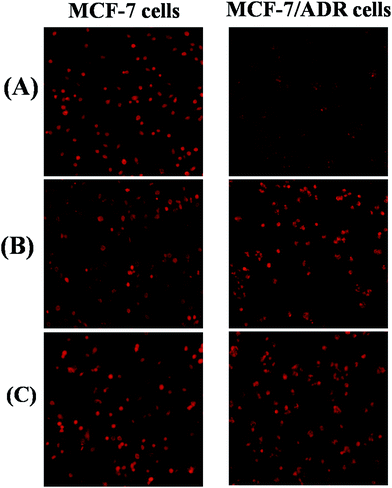 |
| Fig. 7 Fluorescence microscopy images of MCF-7 cells and MCF-7/ADR cells, after incubation for 2 h treated with (A) DOX, (B) polyDOX and (C) poly(DOX + VER) with an equivalent DOX concentration of 3 μg mL−1. | |
Fig. 8 quantitatively supports the results mentioned above by flow cytometry on MCF-7 and MCF-7/ADR cells incubated in free DOX, polyDOX and poly(DOX + VER). The untreated cells served as the control. For the MCF-7 cells, the intracellular fluorescence intensity of free DOX, polyDOX and poly(DOX + VER) was similar. However, the intracellular fluorescence intensity of poly(DOX + VER) was stronger than that of free DOX for MCF-7/ADR cells because the significant decrease in pumpout of free DOX and the different mechanism to enter cells.42 In addition, the intracellular fluorescence intensity of poly(DOX + VER) was also higher than that of polyDOX, indicating that the addition of VER could inhibit the expression of P-gp to reduce the pumpout of DOX significantly. In a word, the poly(DOX + VER) entered into cells via endocytosis mechanism could decrease the pumpout and increase the accumulation of DOX in cancer cells especially resistant cancer cell successfully.
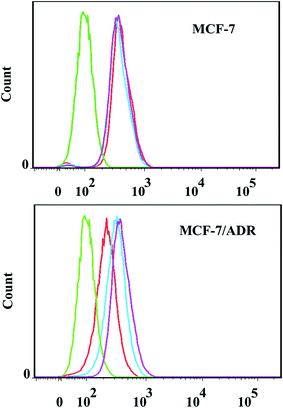 |
| Fig. 8 Fluorescence intensity of MCF-7 and MCF-7/ADR cells analyzed by flow cytometry after treatment with different formulations with an equivalent DOX of 3 μg mL−1 for 2 h. Control (green), DOX (red), polyDOX (blue), poly(DOX + VER) (pink). | |
3.6 Hemolysis test
Hemolysis, defined as the release of hemoglobin into plasma due to damage of erythrocytes membrane, was the important index to evaluate the blood compatibility of copolymers. This is because that most of copolymers were designed to be administrated via intravenous injection for the drug delivery applications.43 The hemolysis ratio was usually tested by the UV absorbance of hemoglobin and it has been reported that the pharmaceutical preparation could be applied for intravenous injection when the hemolysis ratio was under 10%.44 Fig. 9 exhibited the hemolysis ratio of copolymer mPEG2K-PCL4K-PGA1K with different concentration. At all concentration of copolymer from 0.014 to 1.4 mg mL−1, the hemolysis ratio was below 5%, indicating the high blood compatibility of the synthesized copolymer mPEG2K-PCL4K-PGA1K and it was suitable for intravenous injection.
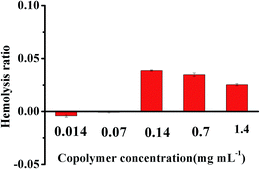 |
| Fig. 9 The hemolysis ratio induced by copolymer mPEG2K-PCL4K-PGA1K with different concentration incubated at (37.0 ± 1.0) °C for 3 h. | |
4. Conclusion
In the present study, a pH-sensitive polypeptide-based block copolymer mPEG2K-PCL4K-PGA1K was synthesized and it could self-assemble into polymersomes to co-deliver the hydrophilic drugs DOX and VER. The size of the polymersomes was about 50–60 nm, which was the appropriate size for EPR effect to achieve passive targeting and improve the accumulation of drugs in tumor issue. In addition, the pH-sensitive property of poly(DOX + VER) made the high accumulation of drugs in target site by accelerating the release of drugs at tumor issue (acidic environment) and simultaneously reducing the amount of drugs in the blood circulation. The poly(DOX + VER) showed much higher cytotoxicity and cellular uptake on MCF-7/ADR resistant cells than polyDOX or free DOX. However, there was nearly no differences for the three formulations on MCF-7 cells, suggesting that VER could inhibit the expression of P-gp on resistant cancer cells successfully and improve the anticancer effect of DOX. In addition, the low hemolysis ratio of copolymer to rabbit RBCs indicated that it could be applied safely for intravenous injection. Conclusively, encapsulating both anticancer drug DOX and P-gp inhibitor VER in pH-sensitive polymersomes could markedly increase the tumor growth inhibition ability and reduce the side effects of the drug during the therapeutic procedure. Therefore, the prepared poly(DOX + VER) could effectively reverse the multidrug resistance and was expected to be a promising drug delivery system for cancer therapy.
Acknowledgements
We gratefully acknowledge the financial support from National Natural Science Foundation of China (NSFC, No. 21373126) and the China–Australia Centre for Health Sciences Research (CACHSR).
References
- T. Genzou and H. Fujiwara, Prog. Cardiovasc. Dis., 2007, 49, 330–352 CrossRef PubMed.
- K. K. Upadhy, A. N. Bhatt, A. K. Mishra, B. S. Dwarakanath, S. Jain, C. Schatz, J. F. L. Meins, A. Farooque, G. Chandraiah, A. K. Jain, A. Misra and S. Lecommandoux, Biomaterials, 2010, 31, 2882–2892 CrossRef PubMed.
- G. Szakács, J. K. Paterson, J. A. Ludwig, C. Booth Genthe and M. M. Gottesman, Nat. Rev. Drug Discovery, 2006, 5, 219–234 CrossRef PubMed.
- A. Abolhoda, A. E. Wilson, H. Ross, P. V. Danenberg, M. Burt and K. W. Scotto, Clin. Cancer Res., 1999, 5, 3352–3356 CAS.
- Y. J. Gu, J. Cheng, C. W.-Y. Man, W. T. Wong and S. H. Cheng, Nanomedicine: Nanotechnology, Biology and Medicine, 2012, 8, 204–211 CrossRef CAS PubMed.
- G. Speelmans, R. W. H. M. Staffhorst, F. A. de Wolf and B. de Kruijff, Biochim. Biophys. Acta, 1995, 1238, 137–146 CrossRef.
- A. Singh, M. Talekar, T. H. Tran, A. Samanta, R. Sundaram and M. Amiji, J. Mater. Chem. B, 2014, 2, 8069–8084 RSC.
- J. C. Wang, X. Y. Liu, W. L. Lu, A. Chang, Q. Zhang, B. C. Goh and H. S. Lee, Eur. J. Pharm. Biopharm., 2006, 62, 44–51 CrossRef CAS PubMed.
- L. Candussio, G. Decorti, E. Crivellato, M. Granzotto, A. Rosati, T. Giraldi and F. Bartoli, Life sci., 2002, 71, 3109–3119 CrossRef CAS.
- M. Qin, Y. E. K. Lee, A. Ray and R. Kopelman, Macromol. Biosci., 2014, 14, 1106–1115 CrossRef CAS PubMed.
- F. Meng and Z. Zhong, J. Phys. Chem. Lett., 2011, 2, 1533–1539 CrossRef CAS.
- J. Wu, Y. Lu, A. Lee, X. Pan, X. Yang, X. Zhao and R. J. Lee, J. Pharm. Pharm. Sci., 2007, 10, 350–357 CAS.
- J. M. Shen, F. Y. Gao, T. Yin, H. X. Zhang, M. Ma, Y. J. Yang and F. Yue, Pharmacol. Res., 2013, 70, 102–115 CrossRef CAS PubMed.
- B. Chen, C. Wu, R. X. Zhuo and S. X. Cheng, RSC Adv., 2015, 5, 6807–6814 RSC.
- Y. C. Huang, Y. S. Yang, T. Y. Lai and J. S. Jan, Polymer, 2012, 53, 913–922 CrossRef CAS PubMed.
- J. Gaspard, J. A. Silas, D. F. Shantz and J. S. Jan, Supramol. Chem., 2010, 22, 178–185 CrossRef CAS PubMed.
- M. T. Popescu, M. Korogiannaki, K. Marikou and C. Tsitsilianis, Polymer, 2014, 55, 2943–2951 CrossRef CAS PubMed.
- H. Oliveira, E. Pérez Andrés, J. Thevenot, O. Sandre, E. Berra and S. Lecommandoux, J. Controlled Release, 2013, 169, 165–170 CrossRef CAS PubMed.
- H. Yin, H. C. Kang, K. M. Hu and Y. H. Bae, Colloids Surf., B, 2014, 116, 128–137 CrossRef CAS PubMed.
- P. Ni, Q. Ding, M. Fan, J. Liao, Z. Qia, J. Luo, X. Li, F. Luo, Z. Yang and Y. Wei, Biomaterials, 2014, 35, 236–248 CrossRef CAS PubMed.
- T. K. Endres, M. Beck Broichsitter, O. Samsonova, T. Renette and T. H. Kissel, Biomaterials, 2011, 32, 7721–7731 CrossRef CAS PubMed.
- G. Gu, H. Xia, Q. Hu, Z. Liu, M. Jiang, T. Kang, D. Miao, Y. Tu, Z. Pang, Q. Song, L. Yao, H. Chen, X. Ga and J. Chen, Biomaterials, 2013, 34, 196–208 CrossRef CAS PubMed.
- J. Huang and A. Heise, Chem. Soc. Rev., 2013, 42, 7373–7390 RSC.
- R. H. Juan and S. Lecommandoux, J. Am. Chem. Soc., 2005, 127, 2026–2027 CrossRef PubMed.
- W. Li, F. Nicol and F. C. Szoka, Adv. Drug Delivery Rev., 2004, 56, 967–985 CrossRef CAS PubMed.
- H. Hatakeyama, E. Ito, H. Akita, M. Oishi, Y. Nagasaki, S. Futaki and H. Harashima, J. Controlled Release, 2009, 139, 127–132 CrossRef CAS PubMed.
- B. F. Lin, D. Missirlis, D. V. Krogstad and M. Tirrell, Biochemistry, 2012, 51, 4658–4668 CrossRef CAS PubMed.
- K. Sano, T. Nakajima, P. L. Choyke and H. Kobayashi, ACS Nano, 2012, 7, 717–724 CrossRef PubMed.
- N. E. Kamber, W. Jeong, S. Gonzalez, J. L. Hedrick and R. M. Waymouth, Macromolecules, 2009, 42, 1634–1639 CrossRef CAS.
- X. Ji, C. Shi, L. Qi, Y. Guo, N. Li, Z. Li and Y. Luan, RSC Adv., 2014, 4, 62698–62707 RSC.
- Z. Tian, M. Wang, A. Y. Zhang and Z. G. Feng, Polymer, 2008, 49, 446–454 CrossRef CAS PubMed.
- L. Qi, Y. Guo, J. Luan, D. Zhang, Z. Zhao and Y. Luan, J. Mater. Chem. B, 2014, 2, 8361–8371 RSC.
- X. Yang, H. Hong, J. J. Grailer, I. J. Rowland, A. Javadi, S. A. Hurley, Y. Xiao, Y. Yang, Y. Zhang, R. J. Nickles, W. Cai, D. A. Steeber and S. Gong, Biomaterials, 2011, 32, 4151–4160 CrossRef CAS PubMed.
- W. Zhu, Y. Li, L. Liu, Y. Chen and F. Xi, Int. J. Pharm., 2012, 437, 11–19 CrossRef CAS PubMed.
- P. Liu, H. Yu, Y. Sun, M. Zhu and Y. Duan, Biomaterials, 2012, 33, 4403–4412 CrossRef CAS PubMed.
- A. A. Dar, A. Garai, A. R. Das and S. Ghosh, J. Phys. Chem. A, 2010, 114, 5083–5091 CrossRef CAS PubMed.
- S. Das, D. K. Sharma, S. Chakrabarty, A. Chowdhury and S. S. Gupta, Langmuir, 2015, 31, 3402–3412 CrossRef CAS PubMed.
- S. Lv, M. Li, Z. Tang, W. Song, H. Sun, H. Liu and X. Chen, Acta Biomater., 2013, 9, 9330–9342 CrossRef CAS PubMed.
- F. Wang, D. Zhang, Q. Zhang, Y. Chen, D. Zheng, L. Hao, C. Duan, L. Jia, G. Liu and Y. Liu, Biomaterials, 2011, 32, 9444–9456 CrossRef CAS PubMed.
- X. Yang, H. Hong, J. J. Grailer, I. J. Rowland, A. Javadi, S. A. Hurley, Y. Xiao, Y. Yang, Y. Zhang, R. J. Nickles, W. Cai, D. A. Steeber and S. Gong, Biomaterials, 2011, 32, 4151–4160 CrossRef CAS PubMed.
- W. M. Li, C. W. Su, Y. W. Chen and S. Y. Chen, Acta Biomater., 2015, 15, 191–199 CrossRef CAS PubMed.
- X. Zeng, R. Morgenstern and A. M. Nyström, Biomaterials, 2014, 35, 1227–1239 CrossRef CAS PubMed.
- D. Li, H. Sun, J. Ding, Z. Tang, Y. Zhang, W. Xu, X. Zhuang and X. Chen, Acta Biomater., 2013, 9, 8875–8884 CrossRef CAS PubMed.
- H. Xu, D. Yang, C. Cai, J. Gou, Y. Zhang, L. Wang, H. Zhong and X. Tang, Acta Biomater., 2015, 16, 156–168 CrossRef CAS PubMed.
Footnote |
† Electronic supplementary information (ESI) available. See DOI: 10.1039/c5ra15313a |
|
This journal is © The Royal Society of Chemistry 2015 |