DOI:
10.1039/C5RA14678J
(Paper)
RSC Adv., 2015,
5, 77689-77698
Identification of the toxic components in Semen Strychni and their metabolites in rat serum by high performance liquid chromatography coupled with a Q Exactive high-resolution benchtop quadrupole Orbitrap mass spectrometer†
Received
24th July 2015
, Accepted 4th September 2015
First published on 8th September 2015
Abstract
Strychnos alkaloids (SAs) are considered to be the main toxic constituents in the traditional Chinese medicine, Semen Strychni. However, a general method for rapid identification of SAs in Semen Strychni and relative metabolites in serum has been time-consuming and difficult to develop due to the high similarity of their structures and physicochemical properties. Hence, this study was conducted to allow rapid and reliable characterization of the alkaloids in SAs extract, the constituents absorbed into blood as well as their metabolites in serum after oral administration of SAs extract based on high performance liquid chromatography coupled with a Q Exactive high-resolution benchtop quadrupole Orbitrap mass spectrometer. The method we developed in the present study only required 16 min with high accurate multiple-stage mass spectrometry (MSn) data. A total of 14 strychnos alkaloids, 5 of which were absorbed into serum, were detected and identified. Moreover, 22 metabolites in serum, including 19 phase I and 3 phase II metabolites were observed and characterized. Results demonstrated that the use of the Q Exactive hybrid quadrupole-Orbitrap mass spectrometer proved to be a very feasible and efficient approach for the rapid separation and identification of the complex constituents in the extract and relative metabolites in vivo. In summary, our study will provide a deep insight into the toxic substances of Semen Strychni and deliver beneficial information for further understanding the in vivo behavior of strychnos alkaloid analogues.
1. Introduction
Traditional Chinese medicines (TCMs) are used widely around the world. With their extensive usage, more and more attention has been paid to the toxicity of TCMs in the last couple of years.1,2 However, the development of a reliable, scientific and objective strategy to investigate the toxic ingredients in TCMs can be very challenging due to the complex constituents of TCMs.3 Serum pharmacochemistry has been increasingly applied in the discovery of bioactive components in TCMs recently.4–6 Based on the theory of serum pharmacochemistry, the constituents absorbed into blood from TCMs maintain considerable levels in the target organ,7 and then have the opportunities to exert toxic effects. Therefore, serum pharmacochemistry also contributes to screening the toxic components in TCMs significantly.
In the past decades, mass spectrometry has become a powerful tool for the rapid and sensitive detection of compounds in a variety of matrices. But two challenges exist in the analysis of compounds in vivo. Firstly, drug metabolites often keep the core structure of the parent drug after biotransformation and possess nearisobaric or isobaric molecular weight. It is urged to provide accurate mass data to identify the compounds with similar or even identical molecular weight in the biological matrix. Secondly, the degree of selectivity should be improved so as to reliably discriminate the targeted analytes from background interferences or endogenous components. To overcome these challenges, the Q-Exactive mass spectrometer can potentially address these issues.8 Up to now, this instrument utilizing high-resolution mass spectrometry (HRMS) has been used for the detection of drugs,9 metabolomics10 and proteomics.11 Combined with serum pharmacochemistry, the platform may greatly assist in the analysis of absorbed toxic constituents and their metabolites in serum with the ability of distinguishing minor isotopes, high resolving power and superior mass accuracy.
Semen Strychni, the seed of Strychnos nux-vomica L., has been used in Chinese folk medicine for hundreds of years with the therapeutic activities of inflammatory, rheumatoid arthritis, facial nerve paralysis and tumor.12–14 However, the use of Semen Strychni is limited for the reason that it is liable to cause various toxicities, including cardiac arrest, epileptic seizures and nephrotoxicity.15–17 It is reported that strychnos alkaloids (SAs), a class of dihydroindole-type alkaloids, are believed to be responsible for the toxic properties of Semen Strychni.18 The metabolism and action mechanism of strychnine and brucine (two typical kinds of SAs) have been preliminarily investigated.19 However, a rapid and reliable approach to reveal the SAs constituents absorbed into blood and their behavior in vivo is still not well developed, which restricts their clinical application and further development.
In the present study, the methodology of serum pharmacochemistry was adopted to analyze the toxic components in Semen Strychni. An HPLC coupled with a Q Exactive hybrid quadrupole-Orbitrap mass spectrometer was applied to separate and identify components in SAs extract and biosamples. Based on the technology a total of 14 SAs were characterized in vitro and 22 metabolites in serum were screened and analysed. Totally 5 original SAs were observed to be absorbed from SAs extract into rat serum, which were probably responsible for the toxic effects of Semen Strychni. Under the optimized conditions, the accurate and rapid simultaneous characterization of the alkaloids in SAs extract and serum as well as the metabolites in vivo was achieved only within 16 min. To our knowledge, this investigation will greatly improve understanding of the real toxic components in Semen Strychni and the short analysis time of the newly developed method would be extremely promising to forensic cases and clinically toxicological relevant researches.
2. Experiment section
2.1 Chemicals and materials
The dried seeds of S. nux-vomica were purchased from Anguo Lengbei Co. Ltd (Hebei, China) and authenticated by Professor Ying Jia (Pharmacognosy Department, Shenyang Pharmaceutical University). The authentic standards of strychnine and brucine were obtained from the National Institute for Control of Pharmaceutical and Biological Products (Beijing, China). The authentic standard strychnine-N-oxide was purchased from Sigma-Aldrich (MO, USA). The purities of all authentic standards are not less than 98.5%. HPLC-grade acetonitrile and methanol were purchased from Fisher Scientific (Nanjing, China). Distilled water, prepared with demineralized water, was used for all aqueous solutions throughout the study.
2.2 Instrument and conditions
A Thermo Scientific™ Ultimate™ 3000 RS system was applied to the assay. The chromatographic separation was carried out on a Thermo Scientific Syncronis C18 (150 × 2.1 mm, 3 μm) column. The mobile phase consisted of acetonitrile (A) and 0.1% formic acid (B). The gradient program was 5% A from 0 to 3 min, 5–45% A from 3 to 16 min, and then went back to 5% A immediately. An aliquot of 10 μL was injected into the Q Exactive hybrid quadrupole-Orbitrap mass spectrometer for HPLC-MS/MS analysis. The flow rate of mobile phase was set at 3 μL min−1.
The optimal conditions of MSn analysis in electrospray ionization (ESI) mode were as follows: probe heater temperature, 350 °C; spray voltage, 3.5 kV for the positive mode; sheath gas, 30 arb; auxiliary gas, 10 arb. Capillary temperature was set at 320 °C and S-lens was 55. The full-scan MS data was produced across the mass range of 100–1000 Da and the resolution was set at 70
000. The stepped normalized collision energy (NCE) setting was 20, 30, 40. All MS spectra were acquired using a Q-Exactive mass spectrometer controlled by the Xcalibur 3.0 software (Thermo Fisher Scientific).
2.3 Preparation of SAs extract
About 0.2 kg of dried seeds were smashed and extracted by reflux with 10, 8 and 6 folds volumes of 50% ethanol in a reflux flask for 60 min sequentially. The solutions were combined together, filtrated, and then concentrated in vacuum to get the crude sample. Then it was suspended into water and acidified to pH = 2 with HCl (1 mol L−1). The mixed solution was extracted with methylene chloride. And the aqueous layer was subjected to be alkalized to pH = 10 with 4 mol L−1 NaOH and was extracted with methylene chloride once more. The methylene chloride layer was treated by rotary evaporation concentration and the resultant residue was the SAs extract.20 Finally, the SAs extract was dissolved in 0.5% carboxymethyl cellulose sodium (CMC-Na) for oral administration.
2.4 Animal treatment and sample collection
All experiments involving rats were carried out in accordance with the approved animal protocols. 12 male pathogen-free Sprague-Dawley rats, weighing 200–240 g, were kindly supplied by the Experimental Animal Center of Shenyang Pharmaceutical University. Animals were housed and handled under suitable humidity, temperature and a 12 h light-dark cycle. All the rats were acclimated for one week with free access to water and standard rodent food at first. 12 rats were randomly divided into two groups, six for each group. Rats in blank group were orally administrated the vehicle (0.5% CMC-Na). And rats in SAs extract group were orally administrated SAs extract for 7 days at a dose of 12 mg kg−1 per day. This dosing protocol was chosen because LD50 value of strychnine in adult rat was about 12 mg kg−1 according to literature.21 Furthermore, histopathological study revealed that a successive administration of this dose for 7 days could induce renal disfunction in our preliminary experiments (ESI Fig. S1†). Blood samples were collected 0.5 h after oral administration of SAs extract from the suborbital vein on the 7th day. Then the blood was drawn and allowed to clot for one hour at room temperature. The serum was obtained by centrifugation at 1435 g for 15 min, and then stored at −80 °C until analysis.
2.5 Preparation of serum samples
The serum samples were thawed at room temperature and precipitated by adding twice the volumes of methanol, then vortexing for 3 min. After centrifugation (11
400g) for 5 min, the supernatant was immediately transferred to a polypropylene tube and evaporated to dryness under a gentle stream of nitrogen at room temperature, then reconstituted with acetonitrile–water (5
:
95, v/v). And an aliquot of 10 μL was injected for HPLC-MS/MS analysis.
2.6 Data processing
MS data processing was carried out using the QualBrowser of Xcalibur 3.0 Software (Thermo Fisher Scientific), including extracted ion chromatograms and calculation of elemental compositions with mass errors within 5 ppm. The chemical structures of all analytes were elucidated and confirmed based on their elemental compositions, accurately measured mass value, the elution order on RP-C18 column, fragmentation behavior, the comparison with authentic standards and literature as far as possible. Meanwhile, the extract ion chromatograms were also used for distinguishing mixed peaks with similar retention time.
3. Result and discussion
3.1 Optimization of LC-ESI-MS conditions
The LC-ESI-MS conditions were tested using the solutions of authentic standards, SAs extract and the serum sample after oral administration of SAs extract. Several trials were investigated to optimize the chromatographic conditions to achieve the desired run time. The addition of formic acid in the mobile phase was found to be helpful to enhance the sensitivity and get better peak shape. MS spectra were obtained in positive ion mode. The positive ion mode was employed for the reason that the structures of most SAs have a basic nitrogen atom, which made them predestined to be monitored by ESI in positive ion mode. Fig. 1 showed the total ion chromatograms of SAs extract (Fig. 1A), blank serum (Fig. 1B) and the rat serum after oral administration SAs extract (Fig. 1C). Under the optimal LC-MS conditions, most of the peaks could be characterized within 16 min without the need of total chromatographic separation due to the high efficiency, sensitivity and superior selectivity of high-resolution mass spectrometry. So the run time of the method we established was reduced considerably than the previous reports, which required more than 80 min.19,22,23 The results indicated that the developed method was reliable and efficient for the study of the absorbed constituents and their metabolites in rat serum after oral administration of SAs extract.
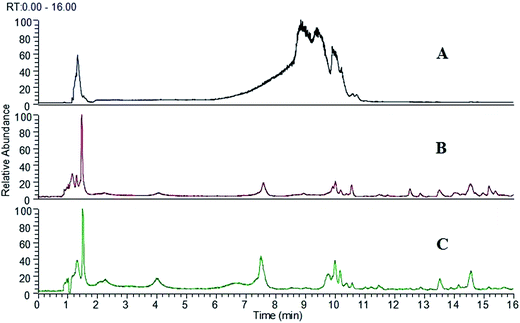 |
| Fig. 1 The total ion chromatograms of SAs extract (A), blank serum (B) and the rat serum after oral administration of SAs extract (C). | |
3.2 Tentative identification of the SAs extract
3.2.1 Tentative identification of strychnine, brucine and strychnine-N-oxide. Because the basic skeletons of SAs were similar, it was reasonable to assume that they had similar MS responses and fragment behavior. Hence, the fragmentation behavior of three authentic standards (strychnine, brucine and strychnine-N-oxide) in positive ion mode was elucidated at first in order to facilitate the structural characterization of the analytes in the subsequent study.
Fragmentation behavior of strychnine. Based on the fragment ion peaks detected in the MS2 spectrum, the possible fragmentation pathways of strychnine were explained and shown in Fig. 2. The quasi-molecular ion [M + H]+ of strychnine was observed at m/z 335.17471 (formula: [C21H23O2N2]+; RT: 9.66 min) and gave a predominant fragment ion at m/z 264.10165 ([C17H14O2N]+), together with a minor fragment ion at m/z 307.14487 ([C19H19O2N2]+) corresponding to a neutral loss of a C2H4 moiety at C17–C18 from the protonated molecule ion. The precursor ion at m/z 264.10165 ([C17H14O2N]+) fragmented into three product ions at m/z 234.09099 ([C16H12ON]+), 222.09081 ([C15H12ON]+) and 184.07545 ([C12H10ON]+) through the elimination of CH2O (C23–C24), C2H2O and C5H4O in the seven-member ring, respectively. These product ions, especially the ion at m/z 264.10165 ([C17H14O2N]+) and the ion at m/z 234.09099 ([C16H12ON]+) were very important characteristic fragment ions for identifying the structure of all analytes.
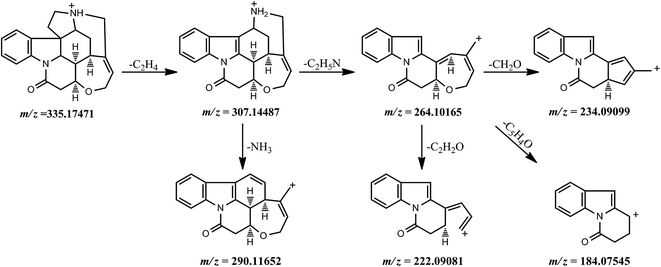 |
| Fig. 2 The proposed fragment pathway of strychnine in positive mode. | |
Fragmentation behavior of strychnine-N-oxide. Strychnine-N-oxide showed an [M + H]+ ion with m/z 351.16983 (formula: [C21H23O3N2]+; RT: 9.64 min), eluted at the retention time of 9.64 min and produced several fragment ions of significant abundance at m/z 334.16687 ([C21H22O2N2]+), 319.14383 ([C20H19O2N2]+), 306.13611 ([C19H18O2N2]+), 291.14874 ([C19H17O2N]+), 277.10922 ([C18H15O2N]+), 261.11493 ([C18H15ON]+) and 220.07542 ([C15H10ON]+). The dominant fragment ion at 334.16687 ([C21H22O2N2]+) corresponded to the loss of an ˙OH radical from the quasi-molecular ion. And it was subjected to fragmenting into these ions at m/z 319.14383 ([C20H19O2N2]+), 306.13611 ([C19H18O2N2]+) and 291.14874 ([C19H17O2N]+) by the elimination of an methyl radical, a C2H4 group and a C2H5N moiety, respectively. The fragment ion at m/z 306.13611 ([C19H18O2N2]+) led to a further loss of CH3NO or CH3N and then gave the product ions at m/z 261.11493 ([C18H15ON]+) and 277.10922 ([C18H15O2N]+), the latter undergoing the loss of a C3H5O˙ group and producing the fragment ion at m/z 220.07542 ([C15H10ON]+). The possible fragmentation pathway of strychnine-N-oxide was shown in Fig. 3.
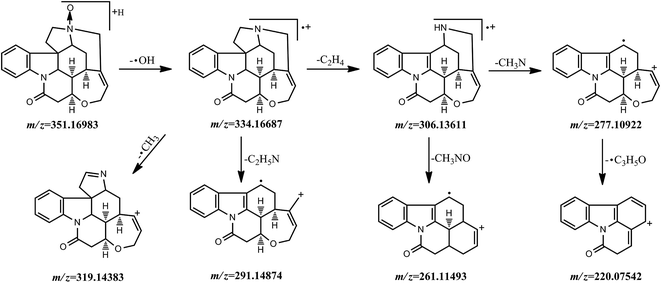 |
| Fig. 3 The proposed fragment pathway of strychnine-N-oxide in positive mode. | |
Fragmentation behavior of brucine. Brucine showed very similar fragmentation behavior with strychnine since it has two additional methoxyl groups in the benzene ring. Hence, its protonated molecule ion at m/z 395.19611 (formula: [C23H27O4N2]+; RT: 9.80 min) and major ion peaks were 60 Da (two methoxyl groups) higher than those of strychnine. However, these fragment ions at m/z 379.16379 ([C22H23O4N2]+), 263.09393 ([C17H13O2N]+), 229.07227 ([C13H11O3N]+) and 213.07863 ([C13H11O2N]+), were only observed in the MS2 spectrum of brucine. And the fragment ion at m/z 263.09393 ([C17H13O2N]+) was produced by a loss of an methoxyl (CH3O˙) radical from the predominant fragment ion at m/z 294.11172 ([C18H16O3N]+). Meanwhile, the precursor ion at m/z 244.09685 ([C14H14O3N]+) fragmented into product ions at m/z 229.07227 ([C13H11O3N]+) and 213.07863 ([C13H11O2N]+) by the loss of methyl (CH3˙) and methoxyl (CH3O˙) radicals, respectively. Fig. 4 depicted the fragmentation process of brucine in detail.
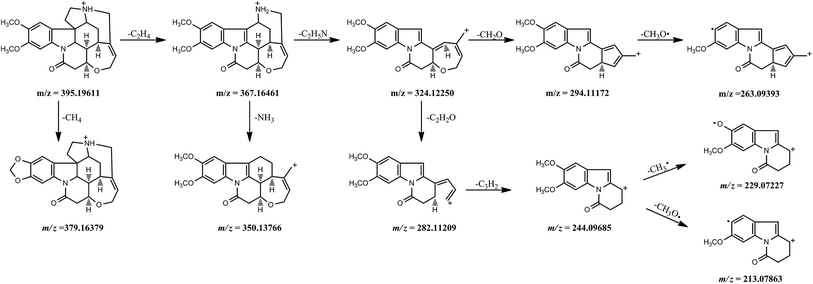 |
| Fig. 4 The proposed fragment pathway of brucine in positive mode. | |
3.2.2 Identification and analysis of herb components in SAs extract. Under the chromatographic and MS conditions as mentioned above, a total of 14 dihydroindole-type alkaloids were identified in SAs extract. Their chemical structures were shown in ESI Fig. S2.† And the information about the analyzed and identified compounds was summarized in Table 1.
Table 1 Major chemical constituents identified in SAs extract
No. |
[M + H]+ (m/z) |
Formula [M + H]+ |
Error (ppm) |
RT (min) |
Fragment ions (m/z) MS/MS |
Identification |
A1 |
335.17502 |
C21H23O2N2 |
1.15 |
9.41 |
307.14447, 272.10593, 264.10217, 234.09163, 222.09109, 184.07576 |
Isostrychnine |
A2 |
335.17505 |
C21H23O2N2 |
1.06 |
9.62 |
307.14621, 290.02399, 264.10677, 234.09071, 222.09094, 184.07676 |
Strychnine |
A3 |
351.16974 |
C21H23O3N2 |
1.65 |
8.27 |
333.15886, 280.09686, 250.08620, 200.07051, 184.07538 |
2-Hydroxystrychnine |
A4 |
351.16971 |
C21H23O3N2 |
1.74 |
9.63 |
333.15997, 280.09586, 250.08653, 200.07033, 184.07544 |
3-Hydroxystrychnine |
A5 |
365.18558 |
C22H25O3N2 |
1.07 |
10.14 |
337.15417, 320.12698, 294.11282, 264.10135, 252.10143, 214.08603 |
α (β)-Colubrine |
A6 |
365.18558 |
C22H25O3N2 |
1.07 |
10.34 |
337.15439, 320.12735, 294.11163, 264.10187, 252.10608, 214.08611 |
α (β)-Colubrine |
A7 |
365.18732 |
C22H25O3N2 |
−3.70 |
10.96 |
308.12784, 280.13385, 250.12221, 238.08571, 222.09215 |
N-Methyl-hydroxystrychnine |
A8 |
365.18533 |
C22H25O3N2 |
1.75 |
9.58 |
349.15366, 308.12659, 290.11780, 264.10202, 248.10608, 236.07069 |
Icajine |
A9 |
381.18039 |
C22H25O4N2 |
1.30 |
10.07 |
363.16986, 306.11203, 252.06554 |
16-OH-α (β)-colubrine |
A10 |
381.18015 |
C22H25O4N2 |
1.93 |
10.27 |
324.12262, 306.10999, 280.09698, 264.10138 |
Vomicine |
A11 |
395.19601 |
C23H27O4N2 |
1.33 |
9.36 |
367.16428, 332.12601, 324.12244, 317.10245, 294.11206 |
Isobrucine |
A12 |
395.19601 |
C23H27O4N2 |
1.33 |
9.77 |
367.16571, 350.13733, 324.12268, 294.11026, 282.11230, 244.09666 |
Brucine |
A13 |
411.19122 |
C23H27O5N2 |
0.56 |
9.92 |
394.18777, 379.16458, 321.15781, 280.09595 |
Brucine-N-oxide |
A14 |
425.20654 |
C24H29O5N2 |
1.31 |
9.21 |
409.17398, 368.14835, 324.12280, 308.12769 |
Novacine |
Identification of components A1 and A2. They exhibited an average experimental [M + H]+ at m/z 335.17503 ([C21H23O2N2]+) and similar dominant fragment ions, indicating that they were isomers. A2 was confirmed as strychnine by comparing the retention time and MS2 fragment ions with the authentic standard. As for A1, the typical fragment ion at m/z 272.10593 was yielded via successive losses of a C2H4 moiety and NH3·H2O from its protonated molecule ion, suggesting that a hydroxyl group was contained in the chemical structure. Therefore, A1 was tentatively identified to be isostrychnine according to its accurate molecular mass and structural characteristic.
Identification of components A3 and A4. A3 and A4 showed an average experimental [M + H]+ at m/z 351.16973 ([C21H23O3N2]+), 16 Da higher than that of strychnine, which indicated that they were isomers of hydroxylation or oxidation products of strychnine. The fragment ions at m/z 280.09686 and 250.08620 were observed in the MS2 spectra of A3, 16 Da higher than the corresponding ions of strychnine, suggesting that the hydroxylation took place at the benzene ring. Meanwhile, the situation of A4 was the same with A3. Thus, A3 and A4 were proposed to be 2-hydroxystrychnine or 3-hydroxystrychnine. However, A3 was more hydrophilic than A4 due to its shorter retention time on the RP-C18 column. Under the consideration of the electronic effect and their hydrophilicity, A3 and A4 were confirmed as 2-hydroxystrychnine and 3-hydroxystrychnine, respectively.19
Identification of components A5–A8. The average experimental [M + H]+ of A5–A8 was at m/z 365.18595 ([C22H25O3N2]+), suggesting that a methoxy group (30 Da) existed in their chemical structure probably. Some major fragment ions, 30 Da higher than those of strychnine, were observed in the MS2 spectra of A5 and A6, indicating the presence of a methoxy group in their benzene ring. Hence, A5 and A6 were tentatively identified as α-colubrine or β-colubrine. In the MS2 spectra of A7, the fragment ions at m/z 280.13385 and 250.12221, 16 Da higher than the corresponding ions of strychnine, confirmed the existence of a hydroxyl group in the benzene ring. A7 was more likely to be N-methyl-hydroxystrychnine, which was consistent with the characteristic fragment at m/z 308.12784, produced by a loss of a C3H7N moiety from the quasi-molecular ion. In case of A8, the typical ion at m/z 308.12659 was generated in the same way with A7, supporting the existence of a N-methyl group. The presence of fragment ions at m/z 290.11780 and 264.10202 contributed to identifying A8 as icajine on the basis of literature data.23
Identification of components A9 and A10. A9 and A10 showed an average experimental [M + H]+ at 381.18027 ([C22H25O4N2]+), a mass shift of 14 Da contrast with that of brucine, and might be the analogues of demethyl brucine. In case of A9, the predominant fragment ion at m/z 363.16986 was yielded by a loss of an H2O from the protonated molecule ion, suggesting the presence of a hydroxyl group. In addition, the cleavage of the bonds between C7 and C17, C16 and C19, C20 and C21 generated the major fragment ion at m/z 306.11203 and described the position of the hydroxyl group only to be C16. Therefore, A9 was confirmed as 16-OH-α-colubrine or 16-OH-β-colubrine. In the MS2 spectrum of A10, these characteristic fragment ions at m/z 324.12262, 306.1099, 280.09698 and 264.10138, totally 16 Da higher than those of icajine (A8), were observed. Therefore, A10 was tentatively identified to be 4-OH-icajine, namely, vomicine, with the assistance of literature.24
Identification of components A11 and A12. Both of them exhibited the quasi-molecular ion [M + H]+ at m/z 395.19601 ([C23H27O4N2]+). Several characteristic fragment ions at m/z 367.16428, 332.12601 and 324.12244 were observed in the spectra of A11. These fragment ions totally shifted to higher molecular values by 60 Da comparing with those of isostrychnine. Thus, A11 was likely to be isobrucine. And A12 was deduced to be brucine from its possessing the similar protonated molecule ion, full-scan MS/MS ion chromatogram and retention time with the authentic standard brucine.
Identification of components A13 and A14. A13 was reasonably identified as brucine-N-oxide because its protonated molecule ion at m/z 411.19122 and fragment ions m/z 394.18777, 379.16458, 321.15781, 280.09595 were completely 60 Da higher than those of authentic strychnine-N-oxide. Similarly, this method was also suitable for the identification of the component A14. Its quasi-molecular ion and major fragment ions were 60 Da higher than those of icajine. Therefore, A14 was identified to be novacine.
3.3 Screening the components absorbed from SAs extract in rat serum
In order to elucidate the constituents absorbed into serum, the extract ion chromatograms were employed to screen the components of interest in the complex biological chromatogram. The components absorbed from SAs extract were tentatively identified through the following steps. First of all, the full scan MS/MS ion chromatogram of the component identified from SAs extract was achieved. Next, comparing with the corresponding ion chromatogram of the blank serum was conducted to remove the interference of endogenous substances. Then the constituent from SAs extract was recognized in serum on the basis of its precise molecular mass, characteristic ion peaks and the chromatographic retention time at last. Efforts were made following the method mentioned above and totally 5 dihydroindole-type alkaloids, namely, A1, A2, A5, A6, A12, were revealed to be absorbed from SAs extract into rat serum. The result indicated that the 5 original constituents that occurred in blood after administration of herbal extracts were probably responsible for the toxic effects of Semen Strychni.
3.4 Tentative identification of the metabolites of components absorbed from SAs extract
The absorbed herb components could be further metabolized by a variety of metabolic enzymes. The biochemical transformation or metabolism is commonly divided into two groups called phase I reactions (oxidation, reduction, and hydrolysis) and phase II reactions (conjugation).25 These metabolites in vivo may be also conducive to the occurrence and development of renal failure. So it is required to investigate the proposed metabolic behavior of the components absorbed into serum. Generally, the absorbed components are subjected to being oxygenized, reduced and hydrolyzed to become phase I metabolites, or conjugated with endogenous molecules (glucuronic acid, amino acid, etc.)26 to form conjugated metabolites (phase II metabolites). Based on the above metabolism rules and relative MS/MS data, a total of 19 phase I metabolites as well as 3 phase II metabolites in serum were identified or tentatively characterized in our present study. The information about the metabolites of the components absorbed from SAs extract into rat serum was summarized in Table 2. By the way, the process of the identification of metabolites was similar to that of the components absorbed into blood.
Table 2 The metabolites of components absorbed from the SAs extract in rat serum
No. |
[M + H]+ (m/z) |
Molecular formula |
Error (ppm) |
RT (min) |
Fragment ions (m/z) MS/MS |
Identification |
M1 |
333.15933 |
C21H21O2N2 |
1.27 |
9.58 |
315.15097, 278.11768, 264.10150, 234.09155, 222.09140, 184.07571 |
11,12-Dehydrostrychnine |
M2 |
333.16120 |
C21H21O2N2 |
−4.34 |
10.80 |
287.14996, 255.06516, 241.14481, 228.13640, 212.10664, 184.11157 |
Dehydrostrychnine |
M3 |
351.16989 |
C21H23O3N2 |
1.22 |
8.50 |
333.15979, 322.14169, 305.12683, 262.12219, 238.084466, 222.09076, 200.07109 |
Strychnine-21,22-epoxide |
M4 |
351.16989 |
C21H23O3N2 |
1.22 |
8.96 |
272.10657, 262.12219, 234.09091, 220.07571, 184.07570 |
22-OH-strychnine |
M5 |
351.16977 |
C21H23O3N2 |
1.56 |
9.63 |
333.15933, 278.11685, 264.10184, 234.09070, 222.09126, 184.07553 |
16-OH-strychnine |
M6 |
351.16977 |
C21H23O3N2 |
1.56 |
9.62 |
334.16711, 319.14398, 306.13611, 277.10916, 261.11386, 220.07553 |
Strychnine-N-oxide |
M7 |
351.16883 |
C21H23O3N2 |
4.24 |
3.34 |
333.14426, 315.13196, 269.09128, 233.09664, 213.08664, 201.10234 |
Hydroxyl-strychnine |
M8 |
353.18552 |
C21H25O3N2 |
1.27 |
8.90 |
290.11609, 272.10739, 248.1076, 234.09240, 184.07570 |
21-Hydro-22-hydroxystrychnine or 22-hydro-21-hydroxystrychnine |
M9 |
367.16473 |
C21H23O4N2 |
1.37 |
9.48 |
349.15421, 335.13553, 321.15903, 294.11151, 197.08273, 220.07530, 184.07573 |
21,22-Epoxide-N-oxide |
M10 |
367.16492 |
C21H23O4N2 |
0.85 |
7.88 |
350.14163, 349.13831, 322.11148, 303.13336 |
Hydroxyl-strychnine-N-oxide |
M11 |
367.16425 |
C21H23O4N2 |
2.68 |
8.09 |
350.14462, 349.14139, 322.11029, 303.13464 |
Hydroxyl-strychnine-N-oxide |
M12 |
367.16479 |
C21H23O4N2 |
1.21 |
9.01 |
349.15543, 322.10687, 304.09506, 286.08521, 258.09116, 220.07545 |
Dihydroxystrychnine |
M13 |
367.16483 |
C21H23O4N2 |
1.10 |
9.27 |
349.15396, 322.11276, 291.50165, 279.14807, 220.07553 |
Dihydroxystrychnine |
M14 |
369.18036 |
C21H25O4N2 |
1.42 |
8.55 |
351.16983, 333.15921, 257.12451, 212.10698, 184.11200 |
21,22-Dihydroxy-22-hydrostrychnine |
M15 |
381.18066 |
C22H25O4N2 |
0.59 |
9.06 |
353.14902, 336.11874, 310.10745, 268.09647, 230.08089 |
2-OH-3-methoxystrychnine or 3-OH-2-methoxystrychnine |
M16 |
381.18066 |
C22H25O4N2 |
0.59 |
9.97 |
365.14902, 337.15298, 321.12518, 318.11316, 303.11288, 286.08551, 258.09116 |
Demethylated brucine |
M17 |
411.19113 |
C23H27O5N2 |
0.77 |
9.21 |
383.16071, 366.13315, 354.13373, 322.10547, 298.10831, 280.09650, 244.09653, 213.07863 |
22-OH-brucine |
M18 |
411.19083 |
C23H27O5N2 |
1.50 |
9.70 |
393.18069, 367.16513, 338.13690, 324.12280, 294.11166, 281.11261, 244.09662, 213.07864 |
16-OH-brucine |
M19 |
429.20175 |
C23H29O6N2 |
0.61 |
8.76 |
411.19107, 393.18306, 272.12772, 244.13301 |
21,22-Dihydroxy-22-hydrobrucine |
M20 |
511.20670 |
C27H31O8N2 |
1.55 |
8.82 |
493.24326, 335.17456 |
Glucuronide conjugate of strychnine |
M21 |
527.20166 |
C27H31O9N2 |
1.42 |
8.05 |
351.17801 |
Glucuronide conjugate of hydroxyl-strychnine |
M22 |
571.22935 |
C29H35O10N2 |
−1.28 |
7.82 |
324.15579 |
Glucuronide conjugate of brucine |
3.4.1 Identification of metabolites M1 and M2. M1 and M2 displayed an average experimental [M + H]+ at m/z 333.16026, suggesting that they were the dehydrogenation products of strychnine. The characteristic fragment ions of M1 at m/z 315.15097 corresponded to the loss of an H2O from the protonated parent molecule, which was supposed to be the presence of a keto–enol tautomer.27 This information could assist in narrowing down the dehydrogenation site to be at C11–C12. Thus, M1 was proposed to be 11,12-dehydrostrychnine. However, the exact dehydrogenation site of M2 was not identified according to its fragment ion information from the MS2 spectra.
3.4.2 Identification of metabolites M3–M7. Obviously, M3–M7 were isomers of mono-oxidized metabolites of strychnine with an average experimental [M + H]+ ion at m/z 351.16963. M3 gave rise to the protonated molecule [M + H]+ at m/z 351.16989. The typical fragment ions at m/z 305.12683 and 262.12219 in the MS2 spectra of M3 shifted to lower molecular values by 2 Da comparing with the corresponding fragment ions of strychnine. It was possibly due to the presence of the C–C double bond formed by the elimination of an H2O. In addition, the fragment ion at m/z 333.15979 was yielded by the loss of an H2O from the quasi-molecular ion and could explain the case. Thus, M3 was identified to be strychnine-21,22-epoxide.M4 showed the protonated quasi-molecule [M + H]+ at m/z 351.16989. Its typical fragment ions at m/z 272.10657, 234.09091, 220.07571 and 184.07570 described that the hydroxylation reaction might take place at the seven-member ring. And with the assistance of the MS/MS data in the literature,28–30 M4 was tentatively identified as 22-OH-strychnine.
In the MS2 spectra of the metabolite M5, the quasi-molecular ion [M + H]+ at m/z 351.16977 and some fragment ions at m/z 264.10184, 234.09070, 222.09126 and 184.07553 were observed, which suggested that the oxidation did not occur at any carbon among C1– C14 or C21–C23 of the basic skeleton. Moreover, the cleavage of the bonds between C7 and C17, C16 and C19, C20 and C21 yielded the product ion at m/z 278.11685 and reasonably explained that the chemical structure of M5 was likely to be 16-OH-strychnine.
As for the metabolite M6, its protonated molecule ion peaks as well as the feature of fragment ions all coincided with the authentic strychnine-N-oxide. Then M6 was unambiguously identified as strychnine-N-oxide.
M7 displayed the protonated molecule ion at m/z 351.16883. The predominant fragment ion at m/z 333.14426 was formed by a neutral loss of an H2O, indicating the existence of a hydroxyl group. However, the site of the hydroxyl group still remained vague and its exact chemical structure was not elucidated.
3.4.3 Identification of the metabolite M8. M8 showed a protonated quasi-molecule [M + H]+ at m/z 353.18552, corresponding to the hydrolysis product of strychnine. The major fragment ions at m/z 234.09240 and 184.07570 described that the lactam moiety of the basic skeleton kept unchanged and that the hydrolysis did not occur on the amide bond. Based on the structural characteristic of strychnine, the hydrolysis site was tentatively identified to be on the C21–C23 double bond. And then this deduction was confirmed by the low-abundance fragment ions (the cleavage of the C21–C22 bond) and literature data.19,28 Consequently, M8 was proposed to be 21-hydro-22-hydroxystrychnine or 22-hydro-21-hydroxystrychnine.
3.4.4 Identification of metabolites M9–M13. M9–M13 gave rise to an average protonated molecule ion [M + H]+ at m/z 367.16470, an addition of 32 Da to the molecular weight of strychnine, suggesting that these metabolites were generated by the successive oxidation or hydroxylation of strychnine. M9 showed the protonated molecule [M + H]+ at m/z 367.16473 and fragmented into these product ions at m/z 335.13553, 321.15903, 294.11151 and 197.08273. The characteristic fragment ions totally agreed with the data reported in the literature.19 This result described the cleavage of the C20–C21 bond and the presence of the 21,22-epoxide constitution. Therefore, M9 was tentatively elucidated as strychnine 21,22-epoxide-N-oxide.In the MS2 spectra of the metabolite M10, a major ion peak at m/z 349.13831 ([M + H − H2O]+), accompanying with a minor ion peak at m/z 350.14163 ([M + H − OH]+˙) was observed. Hence, we deduced that the hydroxylation seemed to occur at the strychnine-N-oxide. For the metabolite M11, its situation was the same with M10. Therefore, M10 and M11 were proposed to be hydroxyl-strychnine-N-oxide. As for metabolites M12 and M13, they were consistent with the sequential oxidation of strychnine to a large degree according to their quasi-molecular ions and major fragment ions. Thus, they were tentatively identified to be dihydroxystrychnine.
3.4.5 Identification of the metabolite M14. The quasi-molecular ion [M + H]+ of M14 at m/z 369.18036, 2 Da higher than the average protonated molecule ion of M9–M13, suggested that M14 was the hydrolysis–oxidation metabolite of strychnine. The fragment ions at m/z 351.16983 and 333.15921 were yielded via a successive neutral loss of an H2O from the corresponding precursor ion, which indicating the presence of two hydroxyl groups in the chemical structure. Besides, there were two conjugating sites at C21 and C22 in terms of the chemical structure. According to the analysis above, M14 were tentatively identified as 21,22-dihydroxy-22-hydrostrychnine.
3.4.6 Identification of metabolites M15 and M16. Both of them showed a protonated molecule ion at m/z 381.18066, 14 Da less than that of brucine, indicating that they were the isomers of demethylated brucine. The characteristic ion peaks at m/z 353.14902, 336.11874, 310.10745 and 268.09647 were observed in the MS2 spectra of the metabolite M15. These peaks displayed a mass shift to the low value by 14 Da comparing with those of brucine, which explained that a single demethylation took place at the benzene ring of brucine. Since there were two methoxyl groups located at the C2 and C3, we could not figure out which one was subjected to the demethylation. Therefore, M15 were tentatively identified as 2-OH-3-methoxystrychnine or 3-OH-2-methoxystrychnine. However, in case of M16 its exact chemical structure was not characterized.
3.4.7 Identification of metabolites M17–M18. M17 and M18 showed an average protonated molecule ion [M + H]+ at m/z 411.19098, an addition 16 Da to the quasi-molecular ion of brucine, indicating that they were the hydroxylation or single oxidation products. The low mass ion peaks in the MS2 spectra of M17 and M18 were identical to those of brucine. Hence, the sites where the reaction occurred were confined to be C7–C23. For the metabolite M17, these fragment ions at m/z 322.10547, 280.09650, 244.09653, were 60 higher than those of 22-OH-strychnine. Furthermore, the characteristic fragment ions at m/z 383.16071 and 366.13315 suggested that the hydroxylation point was on the C22. Thus, M17 was tentatively identified as 22-OH-brucine. And M18 was characterized as to be 16-OH-brucine in the same way as M17 because some typical product ions at m/z 393.18069, 367.16513, 338.13690 and 324.12280 were found in the MS2 spectra of M18 and that these ions were overall 60 Da higher than those of 16-OH-strychnine identified above.
3.4.8 Identification of the metabolite M19. M19 showed a protonated quasi-molecule [M + H]+ at m/z 429.20175 and was proposed to be 21,22-dihydroxy-22-hydrobrucine due to the comparison with the metabolite 21,22-dihydroxy-22-hydrostrychnine in a similar manner to M17 and M18.
3.4.9 Identification of metabolites M20–M22. M20 and M22 gave rise to the protonated quasi-molecule [M + H]+ at m/z 511.20670 and 571.22935, a 176 Da addition of strychnine and brucine, respectively, showing the presence of a glucosyl group. The characteristic fragment ions at m/z 335.17456 and 324.15579 further confirmed that they seemed to be glucuronide conjugate of strychnine and glucuronide conjugate of brucine individually. As to M21, the quasi-molecular ion [M + H]+ at m/z 527.20166 and the fragment ion at m/z 351.17801 were observed in the spectrum. The obtained information corresponded to the occurrence of hydroxylation–glucuronidation. Finally, M21 was proposed to be glucuronide conjugate of hydroxyl-strychnine.These results above demonstrated that the components absorbed could be metabolized diversely. The observed biotransformation pathways of the components absorbed involved hydroxylation, demethylation, N-oxidation, methoxylation, hydrolysis–oxidation and glucuronidation.
4. Conclusion
In the present study, an HPLC coupled with a Q Exactive high-performance benchtop quadrupole-Orbitrap mass spectrometer method was developed and applied to elucidate and identify the herb components in the SAs extract, the absorbed compounds and their metabolites in rat serum after oral administration of the SAs extract. A total of 14 herb components, 5 of which were absorbed into serum, were detected and tentatively identified. Furthermore, the metabolic profile of the constituents absorbed was investigated in detail. A total of 19 phase I and 3 phase II metabolites were observed and characterized based on the general metabolism rules and the comparison of the MS/MS spectra with those corresponding components. Our results demonstrated that the absorbed constituents and their metabolites identified in the serum may be responsible for the toxicological actions of Semen Strychni. Meanwhile, the Q-Exactive mass spectrometer proved to be a powerful tool to investigate the unknown components in a complex matrix due to its features of high mass resolution and accurate mass measurement. In conclusion, this study would contribute to providing an improved understanding of the toxic substances, the metabolic behavior in vivo, and the toxic actions of Semen Strychni.
Abbreviations
SAs | Strychnos alkaloids |
TCMs | Traditional Chinese medicines |
HRMS | High-resolution mass spectrometry |
NCE | Normalized collision energy |
CMC-Na | Carboxymethyl cellulose sodium |
HPLC | High performance liquid chromatography |
MS | Mass spectrometry |
ESI | Electrospray ionization |
Acknowledgements
Conceived and designed the experiments: S. J. Li, X. H. Chen. Performed the experiments: S. J. Li, M. Y. Zhang, C. Z. Hou. Analyzed the data: S. J. Li, P. Y. Hou, R. W. Zhang. Contributed reagents/materials/analysis tools: S. J. Li, K. S. Bi, X. H. Chen. Wrote the paper: S. J. Li.
References
- D. Shaw, Planta Med., 2010, 76, 2012–2018 CrossRef CAS PubMed.
- L. Wen, J. H. Piao and J. Jiang, Expert Opin. Drug Saf., 2012, 11, 985–1002 Search PubMed.
- W. Li, Z. Chen, Y. Liao and H. Liu, Anal. Sci., 2005, 21, 1019–1029 CrossRef CAS.
- J. H. Liu, H. Sun, A. H. Zhang, G. L. Yan, Y. Han, C. S. Xue, X. H. Zhou, H. Shi and X. J. Wang, Biomed. Chromatogr., 2014, 28, 500–510 CrossRef CAS PubMed.
- X. Y. He, Q. C. Liu, W. Peng, Y. L. Huang and C. J. Wu, Pharm. Biol., 2013, 51, 629–634 CrossRef CAS PubMed.
- X. Ying, M. Liu, Q. Liang, M. Jiang, Y. Wang, F. Huang, Y. Xie, J. Shao, G. Bai and G. Luo, J. Ethnopharmacol., 2013, 150, 324–338 CrossRef CAS PubMed.
- R. Teschke, A. Wolff, C. Frenzel and J. Schulze, Aliment. Pharmacol. Ther., 2014, 40, 32–50 CrossRef CAS PubMed.
- Q. Hu, R. J. Noll, H. Li, A. Makarov, M. Hardman and R. Graham Cooks, J. Mass Spectrom., 2005, 40, 430–443 CrossRef CAS PubMed.
- M. Solliec, A. Roy-Lachapelle and S. Sauvé, Anal. Chim. Acta, 2015, 853, 415–424 CrossRef CAS PubMed.
- X. Liu, Z. Ser and J. W. Locasale, Anal. Chem., 2014, 86, 2175–2184 CrossRef CAS PubMed.
- S. Gallien, E. Duriez, C. Crone, M. Kellmann, T. Moehring and B. Domon, Mol. Cell. Proteomics, 2012, 11, 1709–1723 Search PubMed.
- Chinese Pharmacopoeia Commission, Pharmacopoeia of the People's Republic of China, Chemical Industry Press, Beijing, China, 2010 Search PubMed.
- X. K. Deng, W. Yin, W. D. Li, F. Z. Yin, X. Y. Lu, X. C. Zhang, Z. C. Hua and B. C. Cai, J. Ethnopharmacol., 2006, 106, 179–186 CrossRef CAS PubMed.
- W. Yin, T.-S. Wang, F.-Z. Yin and B.-C. Cai, J. Ethnopharmacol., 2003, 88, 205–214 CrossRef CAS.
- G. Philippe, L. Angenot, M. Tits and M. Frédérich, Toxicon, 2004, 44, 405–416 CrossRef CAS PubMed.
- M. Wu, M. Fang, Y. Hu and X. Wang, Seizure, 2012, 21, 311–315 CrossRef PubMed.
- L. Gu, X. Wang, Y. Zhang, Y. Jiang, H. Lu, K. Bi and X. Chen, J. Sep. Sci., 2014, 37, 1058–1066 CrossRef CAS PubMed.
- J. Chen, Y. Qu, D. Wang, P. Peng, H. Cai, Y. Gao, Z. Chen and B. Cai, Molecules, 2014, 19, 4395–4408 CrossRef PubMed.
- J.-X. Tian, C. Peng, L. Xu, Y. Tian and Z.-J. Zhang, Biomed. Chromatogr., 2013, 27, 775–783 CrossRef CAS PubMed.
- J. Y. Zhang, N. Li, K. Hu and P. F. Tu, J. Chin. Pharm. Sci., 2012, 21, 187–191 CAS.
- C. R. Mackerer, R. L. Kochman, T. F. Shen and F. M. Hershenson, J. Pharmacol. Exp. Ther., 1977, 201, 326–331 CAS.
- J.-X. Tian, M. Wang, L. Xu, Y. Tian, R. Song, F. G. Xu and Z.-J. Zhang, Bioanalysis, 2014, 6, 137–149 CrossRef CAS PubMed.
- J. X. Tian, Y. Tian, L. Xu, J. Zhang, T. Lu and Z. J. Zhang, Phytochem. Anal., 2014, 25, 36–44 CrossRef CAS PubMed.
- W. Wu, C. Qiao, Z. Liang, H. Xu, Z. Zhao and Z. Cai, J. Pharm. Biomed. Anal., 2007, 45, 430–436 CrossRef CAS PubMed.
- M. Holčapek, L. Kolářová and M. Nobilis, Anal. Bioanal. Chem., 2008, 391, 59–78 CrossRef PubMed.
- W. Chan, L. Cui, G. Xu and Z. Cai, Rapid Commun. Mass Spectrom., 2006, 20, 1755–1760 CrossRef CAS PubMed.
- M. Mishima, Y. Tanimoto, K. Oguri and H. Yoshimura, Drug Metab. Dispos., 1985, 13, 716–721 CAS.
- X. Liu, S. Zheng, Z. Jiang, C. Liang, R. Wang, Z. Zhou, Y. Zhang and Y. Yu, J. Sep. Sci., 2014, 37, 764–774 CrossRef CAS PubMed.
- K. Oguri, Y. Tanimoto, M. Mishima and H. Yoshimura, Xenobiotica, 1989, 19, 171–178 CrossRef CAS PubMed.
- J. Hou, Q. Liu, Y. Li, H. Sun and J. Zhang, BioMed Res. Int., 2014, 2014, 850493 Search PubMed.
Footnote |
† Electronic supplementary information (ESI) available. See DOI: 10.1039/c5ra14678j |
|
This journal is © The Royal Society of Chemistry 2015 |