DOI:
10.1039/C5RA11433K
(Paper)
RSC Adv., 2015,
5, 57692-57699
Synergistic effect of donors on tetracyanobutadine (TCBD) substituted ferrocenyl pyrenes†
Received
15th June 2015
, Accepted 26th June 2015
First published on 26th June 2015
Abstract
A set of ferrocenyl substituted pyrenes 2 and 3a–3c were designed and synthesized by the Pd-catalyzed Suzuki and Sonogashira cross-coupling reactions. The [2+2] cycloaddition–retroelectrocyclization reaction of ferrocenyl substituted pyrenes 3a and 3b with tetracyanoethylene (TCNE), resulted in tetracyanobutadine (TCBD) derivatives 4a, and 4b respectively. The synergistic effects of two donors (ferrocene and pyrene) and a TCBD acceptor on their photonic properties, energy levels and donor–acceptor interactions are evaluated. The photophysical and electrochemical properties of 2 and 3a–3c were compared with 4a and 4b. The ferrocenyl substituted pyrene 4b exhibits a strong charge transfer band from pyrene to TCBD and a weak charge transfer band from ferrocene to TCBD, whereas 4a exhibits two strong charge transfer bands, one from ferrocene to TCBD and another from pyrene to TCBD. The characteristic emission pattern of ferrocenyl substituted pyrenes 2, 3a–c, 4a and 4b indicates the emission from the pyrene moiety. The electrochemical properties reveal strong electronic communication between ferrocene and TCBD in 4a, and weak electronic communication in 4b. The experimental observations and conclusions were supported by computational calculations. The single crystal X-ray structure of ferrocenyl substituted pyrene 3b is reported.
Introduction
In recent years research on multistate redox active π-conjugated donor–acceptor (D–A) molecular systems has gained momentum due to their wide range of applications in non-linear optics (NLO), photovoltaic systems, light harvesting antenna, energy transfer cassettes, sensors and memory storage devices.1 The photonic properties of D–A systems can be tuned by slight variations in the nature of the donor, acceptor or the spacer unit.2 The synergistic effect of multiple donors containing a reversible redox active center and an acceptor can further perturb the electronic energy levels of the molecule. We were interested to see the synergistic effect of two donors (ferrocene and pyrene) and the TCBD acceptor on photonic properties, energy levels and D–A interactions.
Pyrene is the smallest peri-fused polycyclic aromatic hydrocarbon widely studied for its unique photonic and optoelectronic applications.3 It is a versatile fluorophore and exhibits strong absorption and high fluorescence quantum yield. It is well known donor but can behave as an acceptor, depending on the nature of the attached substituent.4
The ferrocene is widely studied metallocene with strong electron donating ability, rich electrochemistry and non-linear optical behavior.5 Our group has explored ferrocene as a strong donor and attached to variety of chromophores with varying spacer length.6 Pyrene and ferrocene both are strong donors.7 There are couple of reports, where ferrocenyl coupled pyrenes are used for sensing and NLO applications.8
Diederich et al. has explored the [2+2] cycloaddition–retroelectrocyclization reaction of ferrocenyl substituted alkynes with tetracyanoethylene (TCNE), which results in TCBD substituted ferrocenyl derivatives.9 The literature reveals that there are no reports on the reaction of alkynylated ferrocenyl pyrenes with TCNE, which will result in unsymmetrical, D–A–D′ type molecular system.
Herein we report the design, synthesis and properties of ferrocenyl substituted pyrenes and their TCBD derivatives. The ferrocenyl substituted pyrenes (2 and 3a–3c) of varying spacer length were synthesized by Suzuki and Sonogashira coupling reactions and the TCBD derivatives 4a and 4b were synthesized by [2+2] cycloaddition–retroelectrocyclization reaction. We were interested to see the synergistic effect of two donors (ferrocene and pyrene) and the TCBD acceptor on the photonic properties, energy levels and D–A interactions.
Results and discussion
The ferrocenyl substituted pyrenes 2 and 3a–3c were synthesized by the Pd-catalyzed Sonogashira cross-coupling reactions of 1-bromopyrene 1 with corresponding ferrocenyl counterparts (Scheme 1). The Suzuki cross-coupling reaction of 1-bromopyrene 1 with ferrocenyl boronic acid resulted ferrocenyl substituted pyrene 2 in 70% yield, whereas the Sonogashira cross-coupling reaction of 1-bromopyrene 1 with respective ferrocenyl alkynes (a–c) resulted 3a–3c in 68%, 70% and 61% yields respectively. The ferrocenyl substituted pyrenes were purified by repeated column chromatography and recrystallization.
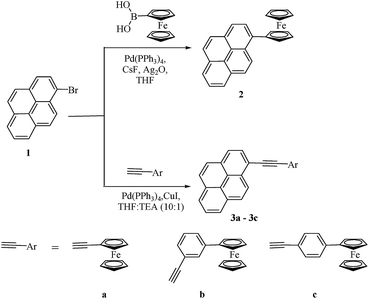 |
| Scheme 1 Synthetic route for the ferrocenyl substituted pyrenes 2 and 3a–3c. | |
The [2+2] cycloaddition–retroelectrocyclization reaction of ferrocenyl substituted pyrene 3a with tetracyanoethylene (TCNE) in dichloromethane at room temperature resulted ferrocenyl substituted pyrene 4a in 80% yield (Scheme 2). Under similar reaction condition ferrocenyl substituted pyrenes 3b and 3c failed to undergo [2+2] cycloaddition–retroelectrocyclization reaction. By increasing the reaction temperature from room temperature to 140 °C, 3b undergoes [2+2] cycloaddition–retroelectrocyclization reaction with TCNE in mixture of solvents dichloroethane and acetonitrile (DCE
:
ACN) (1
:
1) and resulted 4b in 85% yield. Under similar reaction condition ferrocenyl substituted pyrene 3c resulted dark black solid, which was insoluble in common organic solvents. The ferrocenyl substituted pyrenes were well characterized by usual spectroscopic techniques. The 3b was also characterized by single crystal X-ray crystallography.
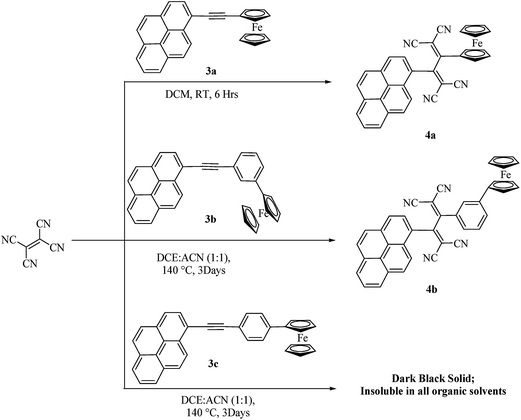 |
| Scheme 2 Synthetic route for the ferrocenyl substituted pyrenes 4a and 4b. | |
Photophysical properties
The color of ferrocenyl substituted pyrenes 2, 3a–3c, 4a and 4b in dichloromethane solution in day light are displayed in Fig. S1 (ESI†). The ferrocenyl substituted pyrenes 3a and 3c exhibit intense yellow color compared to 2 and 3b. The ferrocenyl substituted pyrene 4a exhibits dark gray color due to the presence of two strong charge transfer interactions (from ferrocene to TCBD; pyrene to TCBD) whereas the ferrocenyl substituted pyrene 4b exhibits dark brown color due to single strong charge transfer interaction (from pyrene to TCBD) and weak a charge transfer interaction (from ferrocene to TCBD).
The absorption spectra of ferrocenyl substituted pyrenes 2, 3a–3c, 4a and 4b were recorded in dichloromethane and are displayed in Fig. 1. The S0 → S1 and S0 → S2 absorption bands appear in the regions ∼360 nm and ∼280 nm respectively (Table 1). The successive red shift in the S0 → S1 and S0 → S2 absorption bands of ferrocenyl substituted pyrenes 2, and 3a–3c follows the order 3c > 3b > 3a > 2.
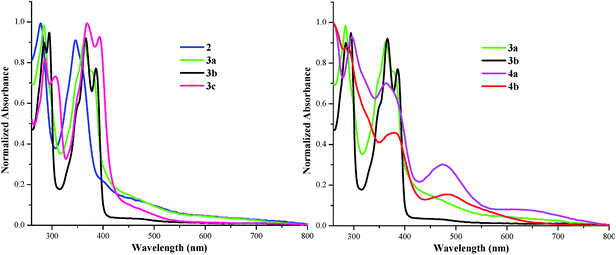 |
| Fig. 1 Normalized electronic absorption spectra of ferrocenyl substituted pyrenes 2, 3a–3c, 4a and 4b in dichloromethane. | |
Table 1 Photophysical properties of ferrocenyl substituted pyrenes 2, 3a–3c, 4a and 4b
|
λmax (nm) |
εa/104 (M−1 cm−1) |
λemb (nm) |
Stokes shift (cm−1) |
ΦFc (%) |
Recorded in dichloromethane solvent. Excited at S0 → S1 absorption band. Determined by using 9,10-diphenyl anthracene as standard (Φ = 0.88, in ethanol). |
2 |
346 |
4.3 |
406 |
4271 |
0.10 |
278 |
4.7 |
3a |
379 (sh) |
4.2 |
406 |
1755 |
0.089 |
363 |
6.0 |
285 |
5.6 |
3b |
385 |
4.1 |
407 |
1404 |
0.099 |
366 |
4.9 |
295 |
5.3 |
285 |
4.9 |
3c |
393 |
4.9 |
407 |
875 |
0.06 |
368 |
5.2 |
306 |
3.8 |
386 |
4.4 |
4a |
639 |
0.71 |
406 |
2842 |
0.11 |
474 |
2.7 |
364 |
6.3 |
295 |
8.4 |
4b |
483 |
0.41 |
406 |
1616 |
0.05 |
381 |
1.2 |
287 |
2.4 |
The absorption spectra of ferrocenyl substituted pyrenes 2 and 3a–3c, show characteristic vibronic pattern of pyrene, on the other hand the characteristic vibronic pattern is disappeared in the absorption spectra of 4a and 4b, indicating strong interaction of the pyrene with TCBD. The ferrocenyl substituted pyrenes 4a and 4b exhibit strong charge transfer bands at 474 nm and 483 nm respectively corresponding to the charge transfer from pyrene to TCBD moiety. The red shifted CT band in 4b than 4a indicates stronger D–A interaction between pyrene and TCBD in 4b. The strong absorption band at 639 nm in 4a corresponds to the charge transfer from ferrocene to TCBD,10 indicating stronger D–A interaction between ferrocene and TCBD in 4a. The small shoulder at lower energy region to the absorption at 483 nm in 4b may be assigned to the weak charge transfer from ferrocene to the TCBD indicating weaker D–A interaction between ferrocene and TCBD due to the meta linkage.
The emission spectra of ferrocenyl substituted pyrenes 2, 3a–3c, 4a and 4b in dichloromethane are displayed in Fig. 2. The characteristic emission pattern indicates the origin of emission from pyrene moiety. The emission wavelengths of ferrocenyl substituted pyrenes were found to be constant, but the quantum yield values were dependent on the nature of substituents. The quantum yield values follow the order 4a > 2 > 3b > 3a > 3c > 4b. The fluorescence intensity increases upon addition of TCBD, in 4a but decreases in 4b, which can be explained on the basis of the frontier molecular orbital (FMO) plots. The FMO plots reveal that the HOMO in 4a is localized on pyrene, whereas in 4b the HOMO is localized on ferrocene unit, which suggests that the fluorescence in 4b is quenched due to fast non radiative deactivation of excited state due to photoinduced charge transfer from donor ferrocene to the acceptor TCBD unit (Fig. 5).11
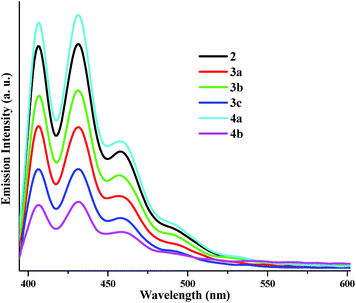 |
| Fig. 2 Emission spectra of ferrocenyl substituted pyrenes 2, 3a–3c, 4a and 4b in dichloromethane at the concentration of 0.1 optical densities. | |
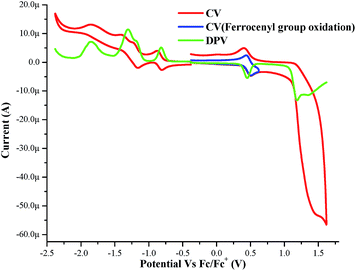 |
| Fig. 3 Overlaid CV and DPV plots of ferrocenyl substituted pyrene 4a. | |
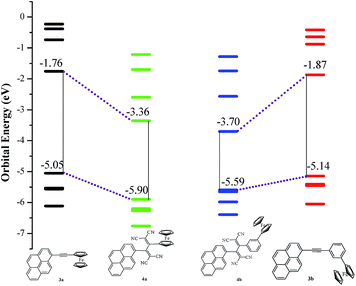 |
| Fig. 4 Comparison of computational energy levels of ferrocenyl substituted pyrenes 3a, 3b and 4a, 4b. | |
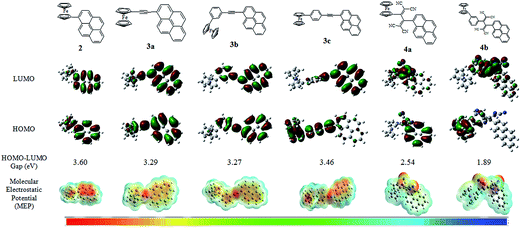 |
| Fig. 5 Frontier molecular orbitals and MEP diagrams of ferrocenyl substituted pyrenes 2, 3a–3c, 4a and 4b at the B3LYP/6-31G** level for C, H and N; and at the Lanl2DZ level for Fe. | |
Electrochemical properties
The electrochemical properties of ferrocenyl substituted pyrenes 2, 3a–3c, 4a and 4b were investigated using cyclic and differential pulse voltammetric (CV and DPV) techniques in dichloromethane solvent using tetrabutylammoniumhexafluorophosphate (Bu4NPF6) as supporting electrolyte. The CV and DPV plots are shown in Fig. 3 and S2–S6 (ESI†); and the corresponding data are listed in Table 2.
Table 2 The electrochemical properties of the ferrocenyl substituted pyrenes 2, 3a–3c, 4a and 4ba
|
E4 Oxidb |
E3 Oxidb |
E2 Oxidb |
E1 Oxid (Fc) |
E1 Red |
E2 Redb |
E3 Redb |
E4 Redb |
The electrochemical analysis was performed in a 0.1 M solution of Bu4NPF6 in dichloromethane at 100 mV s−1 scan rate, versus Saturated Calomel Electrode (SCE) and referenced against Fc/Fc+, the potentials listed are half wave potentials. Irreversible/quasi reversible wave. |
2 |
1.48 |
1.2 |
0.99 |
0.05 |
−1.32 |
−1.85 |
|
|
3a |
— |
1.22 |
0.94 |
0.07 |
−1.31 |
−1.87 |
|
|
3b |
1.19 |
1.08 |
0.79 |
0.03 |
−1.31 |
−1.83 |
|
|
3c |
1.21 |
1.04 |
0.8 |
0.02 |
−1.34 |
−1.88 |
|
|
4a |
|
1.35 |
1.18 |
0.45 |
−0.82 |
−1.18 |
−1.31 |
−1.84 |
4b |
|
|
1.15 |
0.08 |
−0.61 |
−1.12 |
−1.34 |
−1.82 |
From the electrochemical data following observations are apparent: (a) the first oxidation (ferrocenyl oxidation) of 2 and 3a–3c appears at ∼0.05 V. (b) The introduction of electron deficient TCBD unit increases the ferrocenyl oxidation of 4a and 4b to 0.45 and 0.07 V respectively, indicating strong interaction of ferrocene with TCBD in 4a and weak interaction in 4b. The meta linkage between ferrocene and TCBD hinders the electronic communication between ferrocene and TCBD in 4b preventing the delocalization of ferrocenyl electrons to the TCBD. (c) The second oxidation potential of ferrocenyl substituted pyrenes 4a and 4b is higher than that of 2 and 3a–3c due to the introduction of electron deficient TCBD moiety. (d) The introduction of TCBD in 4a and 4b makes their reduction easier than 2 and 3a–3c. (e) The 4a and 4b exhibit two reduction waves corresponding to the reduction of two dicyanovinyl moieties. (f) The delocalization of ferrocenyl electrons to the TCBD makes it relatively electron rich in 4a, which resulted the higher reduction potential of 4a than 4b. The meta linkage in 4b hinders the delocalization electron density from ferrocene to the TCBD.
The computational calculations support the electrochemical studies. The HOMO of ferrocenyl substituted pyrene 4a is localized on pyrene, whereas in 4b it is localized on ferrocene (Fig. 5). The meta linkage in 4b hinders the delocalization of ferrocenyl electrons to electron deficient TCBD which results the localization of HOMO on ferrocene. In case of 4a, the delocalization of ferrocenyl electrons into the TCBD makes ferrocene electron deficient, leading to the localization of HOMO on relatively electron rich pyrene.
Computational calculations
Computational calculations were performed using density functional theory at B3LYP/6-31G** level for C, H and N; and at Lanl2DZ level for Fe. The comparison of energy levels of ferrocenyl substituted pyrenes 3a, and 3b with 4a, and 4b are shown in Fig. 4. The HOMO energy levels are stabilized in 4a and 4b than 3a and 3b. The better stabilization of HOMO in 4a than 4b supports the harder oxidation of 4a than 4b. Similarly the more stabilized LUMO in 4b indicates the easier first reduction of 4b than 4a.
The frontier molecular orbital (FMO) plots and molecular electrostatic potential (MEP) diagram of ferrocenyl substituted pyrenes 2, 3a–3c, 4a and 4b are displayed in Fig. 5. The LUMO of ferrocenyl substituted pyrenes 2 and 3a–3c are located on the pyrene unit and spacer, whereas the LUMO of 4a and 4b is located on TCNE part, indicating strong acceptor nature of TCNE. In 4a the HOMO is localized on pyrene unit, whereas in 4b on ferrocene unit. The HOMO–LUMO distribution pattern indicates strong donor–acceptor interactions in 4a and 4b.
In the MEP diagrams the red color indicates higher electron density whereas the blue color indicates poor electron density. The MEP diagram reveals that the electron density of ferrocenyl substituted pyrenes 2 and 3a–3c is distributed on the entire molecule, whereas in 4a and 4b it is accumulated on TCBD moiety (red color) due to its electron withdrawing nature. The comparison of MEP diagrams of 4a and 4b reveals that in 4b the electron density is accumulated on TCBD and ferrocenyl part also, whereas in case of 4a the electron density is only on TCBD moiety. This supports the strong electronic communication of ferrocene with TCBD in 4a and weak electronic communication in 4b.
Crystal structure
The single crystal of ferrocenyl substituted pyrene 3b was obtained by slow diffusion of hexane into the dichloromethane solution. The crystal data and structure refinement parameters are shown in Table S1 (ESI†). The crystal structure shows slight variations from the energy optimized structure (Fig. 6). The C
C bond is slightly longer in the energy optimized structure than the crystal structure, and the C–C single bonds adjacent to C
C are slightly shorter in energy optimized structure than crystal structure. The cyclopentadienyl ring of ferrocene is staggered in crystal structure with torsional angle of 24.53° whereas in energy optimized structure it is eclipsed (torsional angle = 0°). The slight variations in the crystal structure and energy optimized structure are may be due to the close packing of the crystal in the solid state.
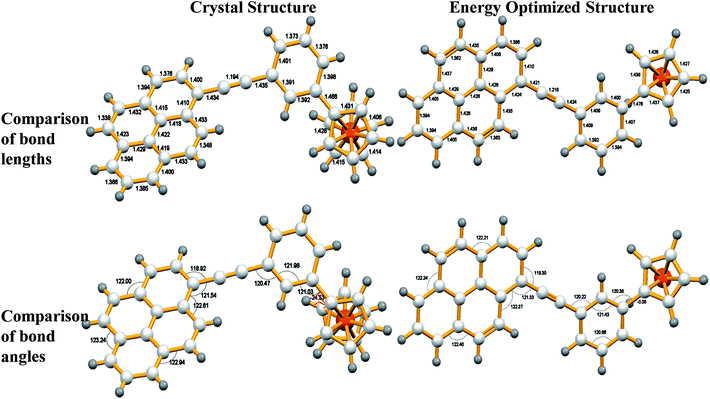 |
| Fig. 6 Comparison of the crystal structure and energy optimized structure of ferrocenyl substituted pyrene 3b. | |
The crystal packing diagram of 3b is displayed in Fig. 7, and the distances of intermolecular interactions are shown in Table 3. The C–H⋯π interactions between the H(30) and H(32) with the π-electron cloud of the cyclopentadienyl ring of ferrocene forms two dimensional chain along b-axis, showing alternate bands of ferrocenyl moiety and pyrene moiety. The extensive π⋯π stacking interactions between the two pyrene rings explores the packing into complex 3D structural motif.
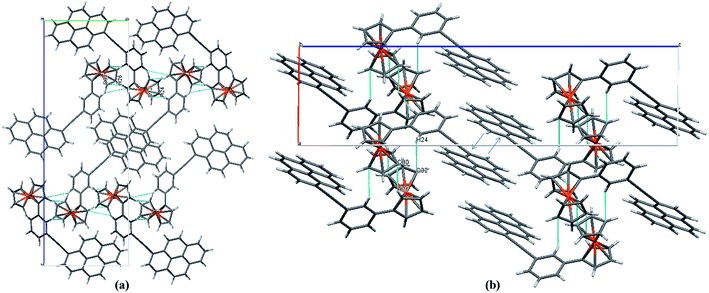 |
| Fig. 7 Crystal packing of ferrocenyl substituted pyrene 3b showing hydrogen bonded 2D network along a-axis (a) and π–π staking interactions along b-axis (b). | |
Table 3 Distances of intermolecular interactions in the crystal structure of 3b
Interaction |
Distance (Å) |
C(26)–H(26)⋯π C(30) |
2.869 |
C(24)–H(24)⋯π C(32) |
3.262 |
C(22)–H(22)⋯π C(26) |
3.572 |
Conclusion
The ferrocenyl substituted pyrenes 2, 3a–3c, 4a and 4b were designed and synthesized using Suzuki and Sonogashira cross-coupling, and [2+2] cycloaddition–retroelectrocyclization reactions. The electronic absorption spectra of 2 and 3a–3c show successive red shift with enhanced conjugation. The ferrocenyl substituted pyrene 4a exhibits two strong charge transfer bands (from pyrene to TCBD and ferrocene to TCBD), on the other hand 4b exhibits one strong charge transfer band (from pyrene to TCBD) and one weak charge transfer band (from ferrocene to TCBD). The spacer group between pyrene and ferrocene does not affect the emission wavelengths, whereas the quantum yield values are the function of spacers. The TCBD derivatives 4a and 4b show difficult oxidation and easier reduction. The ferrocenyl oxidation potential of 4a is much higher than 4b, due to better electronic communication. The photonic applications of these molecules are presently ongoing in our laboratory.
Experimental section
General methods
Chemicals were used as received unless otherwise indicated. All oxygen or moisture sensitive reactions were performed under nitrogen/argon atmosphere using standard schlenk method. 1H NMR (400 MHz), and 13C NMR (100 MHz) spectra were recorded on the Bruker Avance (III) 400 MHz instrument, by using CDCl3 as solvent. 1H NMR chemical shifts are reported in parts per million (ppm) relative to the solvent residual peak (CDCl3, 7.26 ppm). Multiplicities are given as: s (singlet), d (doublet), t (triplet), q (quartet), dd (doublet of doublets), m (multiplet), and the coupling constants, J, are given in Hz. 13C NMR chemical shifts are reported relative to the solvent residual peak (CDCl3, 77.36 ppm). UV-visible absorption spectra of all compounds were recorded on a Cary-100 Bio UV-visible Spectrophotometer. Fluorescence spectra of all the compounds were recorded on a Horiba Jobin Yvon Fluoromax 4P spectrophotometer. Cyclic Voltammograms (CVs) and Differential Pulse Voltammograms (DPVs) were recorded on a CHI620D electrochemical analyzer using Glassy carbon as working electrode, Pt wire as the counter electrode, and Saturated Calomel Electrode (SCE) as the reference electrode. The potentials were referenced against Fc/Fc+ as per IUPAC guidelines.12 HRMS was recorded on Bruker-Daltonics, micrOTOF-Q II mass spectrometer.
Synthesis and characterization
Procedure for Suzuki coupling reaction.
Synthetic procedure for 1-ferrocenylpyrene 2. 1-Bromopyrene (200 mg; 0.7117 mmol) and ferrocenyl boronic acid (196 mg; 0.8540 mmol) were dissolved in dry THF (22 mL) and 5 mL 1 M K2CO3 solution was added, the solution was degassed with argon and, the catalyst Pd(PPh3)4 (8.22 mg; 0.007117 mmol) was added. The reaction mixture was stirred at 80 °C for 24 h. After completion of reaction the solvent was removed under reduced pressure, and the residue was purified by column chromatography on (230–400 mesh size) silica with hexane
:
CH2Cl2 (4
:
1 ratio) and recrystallized from chloroform
:
hexane mixture to yield the desired product 2 as violet powder (181 mg, 0.47 mmol, yield: 68%).
Ferrocenyl substituted pyrene 2. Crystalline solid. Yield: 70% (192 mg); 1H NMR (CDCl3, 400 MHz, ppm): δ 8.75 (d, 2H, J = 8 Hz), 8.41 (d, 2H, J = 8 Hz), 8.13–8.17 (m, 3H), 8.05 (t, 3H, J = 4 Hz), 7.98 (t, 1H, J = 8 Hz), 4.84 (t, 2H, J = 2 Hz), 4.49 (t, 2H, J = 2 Hz), 4.22 (s, 5H); 13C NMR (CDCl3, 100 MHz, ppm): 134.4, 132.0, 131.0, 130.2, 129.1, 129.0, 127.8, 127.3, 127.1, 126.3, 125.8, 125.3, 125.2, 124.9, 124.7, 87.6, 71.3, 70.1, 68.9; HRMS (ESI) m/z = calculated for C26H18Fe = 386.0753, measured 386.0788.
Generalized procedure for Sonogashira coupling reaction. 1-Bromopyrene (200 mg; 0.7117 mmol) 1 and corresponding ferrocenyl alkyne (0.845 mmol) were dissolved in THF
:
triethylamine (10
:
1, v/v; 15 mL). The reaction mixture was purged with argon, and Pd(PPh3)4 (8.24 mg, 5 mol%), and CuI (5 mg, 5 mol%) were added, followed by stirring at 70 °C for 24 hours. Upon completion of reaction, the mixture was evaporated to dryness, and the crude product was dissolved in CH2Cl2, chromatographed on silica (1
:
1; hexanes
:
CHCl3), and recrystallized from chloroform
:
hexane
:
ethanol (7
:
2
:
1) mixture to give 3a–3c (yield 61–68%) as purple crystalline solid.
Ferrocenyl substituted pyrene 3a. Crystalline solid. Yield: 68% (200 mg); 1H NMR (δ): 8.63 (d, 2H, J = 8 Hz), 8.31–8.24 (m, 2H), 8.19–8.16 (m, 2H), 8.08–8.12 (d × 2, 2H, J = 8 Hz), 8.01–8.06 (m, 2H), 4.68 (t, 2H, J = 4 Hz), 4.33–4.35 (m, 7H); 13C NMR (CDCl3, 100 MHz, ppm): 132.1, 131.7, 131.5, 131.1, 129.8, 128.5, 128.2, 127.7, 126.6, 126.0, 125.78, 125.76, 124.95, 124.90, 124.8, 119.0, 94.7, 85.2, 72.0, 70.4, 69.4, 65.8; HRMS (ESI) m/z = calculated for C28H18Fe = 410.0753, measured 410.0761.
Ferrocenyl substituted pyrene 3b. Crystalline solid. Yield: 70% (243 mg); 1H NMR (δ): 8.72 (d, 1H, J = 8 Hz), 8.21–8.27 (m, 4H), 8.10–8.16 (m, 2H), 8.02–8.07 (m, 2H), 7.83 (t, 1H, J = 1.5 Hz), 7.57–7.60 (dt, 1H, J = 1.32 Hz and 1.24 Hz), 7.52–7.55 (dt, 1H, J = 1 and 1.76 Hz), 7.37 (t, 1H, J = 8.4 Hz), 4.74 (t, 1H, J = 2.04 and 1.76 Hz), 4.37 (t, 1H, J = 2 and 1.76 Hz), 4.11 (s, 5H); 13C NMR (CDCl3, 100 MHz, ppm): 140.2, 132.3, 131.6, 131.4, 130.0, 129.6, 129.3, 128.9, 128.7, 128.5, 127.6, 126.60, 126.57, 126.0, 125.9, 124.89, 124.86, 124.7, 123.8, 118.2, 95.7, 88.8, 84.7, 70.0, 70.0, 69.5, 66.9; HRMS (ESI) m/z = calculated for C34H22Fe = 486.1066, measured 486.1070.
Ferrocenyl substituted pyrene 3c. Crystalline solid. Yield: 61% (212 mg); 1H NMR (δ): 8.77 (d, 1H, J = 9 Hz), 8.20–8.25 (m, 4H), 8.02–8.15 (m, 4H), 7.65 (d, 2H, J = 8.2 Hz), 7.53 (d, 2H, J = 8.0 Hz), 4.72 (s, 2H), 4.39 (s, 2H), 4.08 (s, 5H); HRMS (ESI) m/z = calculated for C34H22Fe = 486.1066, measured 486.1101.
Procedure for the synthesis of ferrocenyl substituted pyrene 4a by [2+2] cycloaddition–retroelectrocyclization reaction. TCNE (31 mg, 0.24 mmol) was added to a solution of 3a (100 mg, 0.24 mmol) in dichloromethane (20 mL) under argon atmosphere. The reaction mixture was stirred for 6 h at room temperature. After completion of reaction the solvent was removed under reduced pressure, and the product was purified by column chromatography with dichloromethane as eluent to yield 4a as dark colored solid. Yield: 80% (105 mg).
Ferrocenyl substituted pyrene 4a. Crystalline solid. Yield: 80% (105 mg); 1H NMR (δ): 8.49 (d, 1H, J = 9.28 Hz), 8.36–8.41 (m, 2H), 8.22–8.30 (m, 2H), 8.11–8.18 (m, 2H), 7.94 (d, 1H, J = 8.28 Hz), 5.23 (s, 1H), 4.89 (s, 2H), 4.77 (s, 2H), 4.33 (s, 5H); 13C NMR (CDCl3, 100 MHz, ppm): 135.3, 131.6, 131.5, 130.7, 128.2, 127.9, 127.7, 127.4, 126.1, 125.8, 125.1, 124.6, 123.4, 115.8, 114.5, 112.2, 111.9, 103.7, 95.7, 92.4, 90.7, 86.5, 76.0, 75.8, 75.5, 73.1, 73.0, 72.8, 72.6, 72.1; HRMS (ESI) m/z = calculated for C34H18N4Fe = 477.0513, measured 477.0474.
Procedure for the synthesis of ferrocenyl substituted pyrene 4b by [2+2] cycloaddition–retroelectrocyclization reaction. TCNE (26 mg, 0.21 mmol) was added to a solution of 3b (100 mg, 0.21 mmol) in 1,2-dichloroethane and acetonitrile (1
:
1) solvent mixture (20 mL) under argon atmosphere. The reaction mixture was refluxed for 72 hours at 140 °C temperature. After completion of reaction the solvents were removed under reduced pressure, and the product was purified by column chromatography with dichloromethane as eluent to yield 4b as dark colored solid. Yield: 85% (107 mg).
Ferrocenyl substituted pyrene 4b. Crystalline solid. Yield: 85% (107 mg); 1H NMR (δ): 8.35–8.42 (m, 3H), 8.29 (d, 1H, J = 8.76 Hz), 8.24 (d, 1H, J = 8.28 Hz), 8.11–8.17 (m, 3H), 7.92 (d, 1H, J = 8.04 Hz), 7.7 (bs, 1H), 7.61–7.63 (m, 1H), 7.3–7.4 (m, 2H), 5.54 (t, 2H, J = 1.76 Hz), 4.32 (t, 2H, J = 1.76 Hz), 3.92 (s, 5H); HRMS (ESI) m/z = calculated for C40H22N4Fe = 614.1189, measured 614.1160.
Acknowledgements
RM thanks CSIR, and DST, New Delhi for financial support. BD and TJ thank CSIR and UGC, New Delhi respectively for their fellowships. We are thankful to Sophisticated Instrumentation Centre (SIC), IIT Indore.
References
-
(a) C. Joachim, J. K. Gimzewski and A. Aviram, Nature, 2000, 408, 541–548 CrossRef CAS PubMed;
(b) A. R. Pease, J. O. Jeppesen, J. F. Stoddart, Y. Luo, C. P. Collier and J. R. Heath, Acc. Chem. Res., 2001, 34, 433–444 CrossRef CAS PubMed;
(c) R. F. Service, Science, 2001, 293, 782–785 CrossRef CAS PubMed;
(d) M. Ruben, J. M. Lehn and P. Muller, Chem. Soc. Rev., 2006, 35, 1056–1067 RSC;
(e) G. S. He, L. S. Tan, Q. Zheng and P. N. Prasad, Chem. Rev., 2008, 108, 1245–1330 CrossRef CAS PubMed;
(f) M. Liangwab and J. Chen, Chem. Soc. Rev., 2013, 42, 3453–3488 RSC;
(g) K. Szaciłowski, Chem. Rev., 2008, 108, 3481–3548 CrossRef PubMed;
(h) J. C. May, J. H. Lim, I. Biaggio, N. N. P. Moonen, T. Michinobu and F. Diederich, Opt. Lett., 2005, 30, 3057–3059 CrossRef CAS.
-
(a) Q. T. Zhang and J. M. Tour, J. Am. Chem. Soc., 1998, 120, 5355–5362 CrossRef CAS;
(b) J. L. Wang, Q. Xiao and J. Pei, Org. Lett., 2010, 12, 4164–4167 CrossRef CAS PubMed;
(c) T. Tanaka and A. Osuka, Chem. Soc. Rev., 2015, 44, 943–969 RSC;
(d) H. L. Dong, H. F. Zhu, Q. Meng, X. Gong and W. P. Hu, Chem. Soc. Rev., 2012, 41, 1754–1808 RSC;
(e) B. Dhokale, T. Jadhav, S. M. Mobin and R. Misra, Chem. Commun., 2014, 50, 9119–9121 RSC;
(f) R. Misra, T. Jadhav, B. Dhokale, P. Gautam, R. Sharma, R. Maragani and S. M. Mobin, Dalton Trans., 2014, 13076–13086 RSC;
(g) R. Misra, B. Dhokale, T. Jadhav and S. M. Mobin, Dalton Trans., 2014, 4854 RSC.
-
(a) Y. O. Lee, T. Pradhan, S. Yoo, T. H. Kim, J. Kim and J. S. Kim, J. Org. Chem., 2012, 77, 11007 CrossRef CAS PubMed;
(b) T. M. Figueira-Duarte and K. Mullen, Chem. Rev., 2011, 111, 7260–7314 CrossRef CAS PubMed.
-
(a) S. W. Yang, A. Elangovan, K. C. Hwang and T. I. Ho, J. Phys. Chem. B, 2005, 109, 16628–16635 CrossRef CAS PubMed;
(b) C. Lambert, J. Ehbets, D. Rausch and M. Steeger, J. Org. Chem., 2012, 77, 6147–6154 CrossRef CAS PubMed.
-
(a) P. L. Pauson, Q. Chem. Soc. Rev., 1955, 9, 391–414 RSC;
(b) H. Nishihara, Adv. Inorg. Chem., 2002, 53, 41–86 CrossRef CAS;
(c) D. R. Van Staveren and N. M. Nolte, Chem. Rev., 2004, 104, 5931–5985 CrossRef CAS PubMed;
(d) R. C. J. Atkinson, V. C. Gibson and N. J. Long, Chem. Soc. Rev., 2004, 33, 313–328 RSC;
(e) R. Misra, R. Maragani, T. Jadhav and S. M. Mobin, New J. Chem., 2014, 38, 1446–1457 RSC;
(f) A. Vecchi, P. Galloni, B. Floris, S. V. Dudkin and V. N. Nemykin, Coord. Chem. Rev., 2015, 291, 95–171 CrossRef CAS PubMed;
(g) C. J. Ziegler, K. Chanawanno, A. Hasheminsasab, Y. V. Zatsikha, E. Maligaspe and V. N. Nemykin, Inorg. Chem., 2014, 53, 4751–4755 CrossRef CAS PubMed;
(h) X. Yin, Y. Li, Y. Zhu, X. Jing, Y. Li and D. Zhu, Dalton Trans., 2010, 9929–9935 RSC;
(i) Y. Li, T. Liu, H. Liu, M. Z. Tian and Y. Li, Acc. Chem. Res., 2014, 47, 1186–1198 CrossRef CAS PubMed.
-
(a) B. Dhokale, P. Gautam, S. M. Mobin and R. Misra, Dalton Trans., 2013, 1512–1518 RSC;
(b) P. Gautam, B. Dhokale, S. M. Mobin and R. Misra, RSC Adv., 2012, 2, 12105–12107 RSC;
(c) R. Misra, B. Dhokale, T. Jadhav and S. M. Mobin, Dalton Trans., 2014, 4854 RSC;
(d) R. Misra, B. Dhokale, T. Jadhav and S. M. Mobin, Organometallics, 2014, 33, 1867–1877 CrossRef CAS;
(e) R. Misra, B. Dhokale, T. Jadhav and S. M. Mobin, New J. Chem., 2014, 38, 3579–3585 RSC;
(f) R. Misra and P. Gautam, Org. Biomol. Chem., 2014, 12, 5448–5457 RSC;
(g) R. Misra, R. Maragani, P. Gautam and S. M. Mobin, Tetrahedron Lett., 2014, 55, 7102–7105 CrossRef CAS PubMed;
(h) R. Misra, P. Gautam and R. Maragani, Tetrahedron Lett., 2014, 56, 1664–1666 CrossRef PubMed;
(i) B. Dhokale, T. Jadhav, S. M. Mobin and R. Misra, Dalton Trans., 2015 10.1039/c5dt00565e.
-
(a) T. M. Figueira-Duarte and K. Mullen, Chem. Rev., 2011, 111, 7260–7314 CrossRef CAS PubMed;
(b) S. I. Kirin, H. B. Kraatz and N. M. Nolte, Chem. Soc. Rev., 2006, 35, 348–354 RSC.
-
(a) L. Cuffe, R. D. A. Hudson, J. F. Gallagher, S. Jennings, C. J. McAdam, R. B. T. Connelly, A. R. Manning, B. H. Robinson and J. Simpson, Organometallics, 2005, 24, 2051 CrossRef CAS;
(b) M. D. Gonzalez, F. Oton, R. A. Orenes, A. Espinosa, A. Tárraga and P. Molina, Organometallics, 2014, 33, 2837–2852 CrossRef CAS;
(c) R. Martinez, A. I. Ratera, A. Tarraga, P. Molina and J. Veciana, Chem. Commun., 2006, 3809–3811 RSC;
(d) M. Kaur, P. Kaur, V. Dhuna, S. Singh and K. Singh, Dalton Trans., 2014, 5707–5712 RSC;
(e) T. Romero, A. Caballero, A. Tarraga and P. Molina, Org. Lett., 2009, 11, 3466–3469 CrossRef CAS PubMed.
-
(a) M. Kivala, C. Boudon, J.-P. Gisselbrecht, P. Seiler, M. Gross and F. Diederich, Angew. Chem., 2007, 119, 6473–6477 CrossRef PubMed ; M. Kivala, C. Boudon, J.-P. Gisselbrecht, P. Seiler, M. Gross and F. Diederich, Angew. Chem., Int. Ed., 2007, 46, 6357–6360 Search PubMed;
(b) M. Kivala, T. Stanoeva, T. Michinobu, B. Frank, G. Gescheidt and F. Diederich, Chem.–Eur. J., 2008, 14, 7638–7647 CrossRef CAS PubMed;
(c) B. B. Frank, B. C. Blanco, S. Jakob, F. Ferroni, S. Pieraccini, A. Ferrarini, C. Boudon, J.-P. Gisselbrecht, P. Seiler, G. P. Spada and F. Diederich, Chem.–Eur. J., 2009, 15, 9005–9016 CrossRef CAS PubMed;
(d) M. Yamada, P. Rivera-Fuentes, W. B. Schweizer and F. Diederich, Angew. Chem., Int. Ed., 2010, 49, 3532–3535 CrossRef CAS PubMed;
(e) T. Shoji, A. Maruyama, C. Yaku, N. Kamata, S. Ito, T. Okujima and K. Toyota, Chem.–Eur. J., 2015, 21, 402–409 CrossRef CAS PubMed;
(f) T. Michinobu, Chem. Soc. Rev., 2011, 40, 2306–2316 RSC;
(g) T. Shoji, S. Ito, K. Toyota, M. Yasunami and N. Morita, Chem.–Eur. J., 2008, 14, 8398–8408 CrossRef CAS PubMed;
(h) M. Morimoto, K. Murata and T. Michinobu, Chem. Commun., 2011, 47, 9819–9821 RSC;
(i) T. M. Pappenfus, D. K. Schneiderman, J. Casado, J. T. L. Navarrete, M. C. R. Delgado, G. Zotti, B. Vercelli, M. D. Lovander, L. M. Hinkle, J. N. Bohnsack and K. R. Mann, Chem. Mater., 2011, 23, 823–831 CrossRef CAS;
(j) Y. Hua, J. He, C. Zhang, C. Qin, L. Han, J. Zhao, T. Chen, W. Y. Wong, W. K. Wong and X. Zhu, J. Mater. Chem. A, 2015, 3, 3103–3112 RSC;
(k) W. Y. Wong, Dalton Trans., 2007, 4495–4510 RSC.
-
(a) T. Mochida and S. Yamazaki, J. Chem. Soc., Dalton Trans., 2002, 3559–3564 RSC;
(b) T. Shoji, S. Ito, T. Okujima and N. Morita, Chem.–Eur. J., 2013, 19, 5721–5730 CrossRef CAS PubMed.
-
(a) B. Dhokale, P. Gautam and R. Misra, Tetrahedron Lett., 2012, 53, 2352–2354 CrossRef CAS PubMed;
(b) T. Jadhav, R. Maragani, R. Misra, V. Sreeramulu, D. N. Rao and S. M. Mobin, Dalton Trans., 2013, 4340–4342 RSC;
(c) R. Sharma, R. Maragani, S. M. Mobin and R. Misra, RSC Adv., 2013, 3, 5785 RSC;
(d) R. Misra, T. Jadhav and S. M. Mobin, Dalton Trans., 2013, 16614–16620 RSC;
(e) R. Maragani and R. Misra, Tetrahedron Lett., 2013, 54, 5399–5402 CrossRef CAS PubMed;
(f) R. Misra, T. Jadhav and S. M. Mobin, Dalton Trans., 2014, 2013–2022 RSC;
(g) R. Misra, R. Sharma and S. M. Mobin, Dalton Trans., 2014, 6891–6896 RSC;
(h) V. A. Nadtochenko, N. N. Denisov, V. Y. Gak, N. V. Abramova and N. M. Loim, Russ. Chem. Bull., 1999, 48, 1900 CrossRef CAS;
(i) S. Barlow and S. R. Marder, Chem. Commun., 2000, 1555–1562 RSC.
- G. Gritzner and G. Kuta, Pure Appl. Chem., 1984, 56, 461–466 CrossRef.
Footnote |
† Electronic supplementary information (ESI) available: General experimental methods, electrochemical data, theoretical calculations, crystal structure data and copies of 1H, 13C NMR and HRMS spectra of new compounds. CCDC 1054932 contains crystal structure of 3b. For ESI and crystallographic data in CIF or other electronic format see DOI: 10.1039/c5ra11433k |
|
This journal is © The Royal Society of Chemistry 2015 |
Click here to see how this site uses Cookies. View our privacy policy here.