DOI:
10.1039/C5RA11224A
(Paper)
RSC Adv., 2015,
5, 65768-65774
Long-term reliability of silicon wafer-based traditional backsheet modules and double glass modules†
Received
17th June 2015
, Accepted 27th July 2015
First published on 27th July 2015
Abstract
An extensive program of series extended sequential long-term reliability stress including thermal cycling (TC) 600, damp heat (DH) 3000, 600 hours potential induced degradation (PID) and humidity freeze (HF) 50 were performed on silicon wafer-based traditional backsheet modules and double glass photovoltaic (PV) modules. The relative module maximum power (Pmax) degradations of traditional backsheet modules are 3.87%, 7.34%, 13.3%, 33.73% and those of double glass modules are 2.78%, 3.12%, 2.27%, 2.72%, respectively. From all the above results, HF50 has a greater impact on Pmax degradation of traditional backsheet modules, and a strong correlation is thereby found between the Water Vapor Transmission Rate (WVTR) of the backsheet and the Pmax degradation. Traditional backsheet modules have higher WVTR and greater Pmax degradation, while double glass modules are impermeable and have much lower Pmax degradation. The key factor for excellent performance of Si wafer-based double glass PV modules is replacing the polymer backsheet by a glass panel with impermeability to water vapor, which enables double glass modules to offer much higher reliability and longer durability.
1. Introduction
As a promising renewable energy source, PV energy is gaining great support from governments worldwide. With the newer techniques of manufacturing crystalline Si wafer-based PV modules and efficient power converter design, it is possible to make the PV project cost-effective.1 Si wafer-based double glass photovoltaic modules have exceptionally low degradation, demonstrating their higher reliability and longer durability.2 The durability and reliability of Si wafer-based PV modules are extremely important for lowering the leverage cost of electricity generated by PV. If the lifetime of PV modules can be extended to 40 years instead of 20 years, the cost of modules in a PV power plant can be reduced by a factor of two.
Previously, we have reported the basic design concept of double glass PV modules and performed standard test according to IEC61215 on 28th European Photovoltaic Solar Energy Conference in 2013.3 Compared to the traditional backsheet modules, double glass modules exhibited excellent performance under different stress conditions such as damp heat (DH), thermal cycling (TC), humidity-freeze (HF), mechanical loading, fire class A test and potential induced degradation (PID). Although double-glass PV modules have existed by several groups over the past few years, to the best of our knowledge, few systematical studies about the long-term reliability and the Pmax degradation of double glass modules have been reported. In this work, we performed strict tests in climate chambers to simulate the outdoor field operations, to investigate the long-term reliability of double glass and traditional backsheet PV modules, and try to find out the reason why double glass modules have higher reliability and longer durability. Our results show that great progress has been made in solving differences for two different modules, and we provide new research directions.
2. Experimental
The structure of double glass modules and standard conventional back sheet modules is displayed in Fig. 1. The major difference is the traditional backsheet materials of conventional solar modules is replaced with toughened 2.5 mm-thick glass. The preparing process of the samples comprises the following steps: solar cells are welded and striped followed by a lay up process where we place front glass, solar cells string, EVA, backsheet (or back side glass) and lamilation at temperature 80 to 150 °C for 15 min is operated, then the backsheet modules are framed with Al bars while the double glass modules are frameless, finally the modules are characterized by I–V and EL measurements.
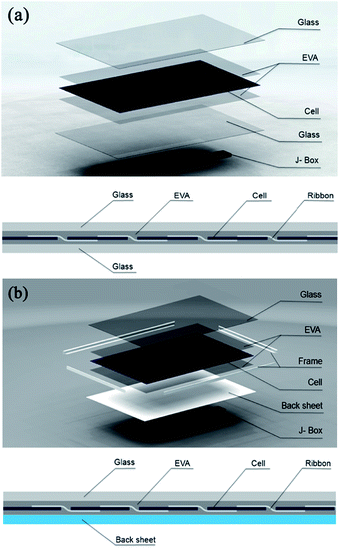 |
| Fig. 1 Structure of double glass (a) and traditional module (b). | |
To systematically investigate the degradation behavior of double glass modules compared with traditional backsheet modules, we carried out an extensive program with series sequential long-term reliability accelerated tests and characterizations in the State Key Lab of PV Science and Technology (PVST) in Trina solar. The current–voltage (I–V) characteristics of all PV modules were measured under standard test conditions (STC, under 1000 W m−2 irradiance density and 25 °C module temperature) before and after different accelerated environments stress tests. Visual inspections were also carried out according to IEC 61215.4 12 pieces of each commercially available traditional backsheet PV modules and double glass PV modules were selected to perform the fair comparison. Each module has 60 pieces 156 × 156 mm2 multi-crystalline solar cells connected with ribbons. To minimize the testing errors, all accelerated stress tests were carried out under the same condition, with the same operators, the same chamber and the same IV tester. Both the traditional backsheet modules and the double glass modules were tested under extended climate chamber tests which have 3 times above longer testing times comparing to the standard requirements as described in IEC61215 standard,4 i.e. 600 thermal cycles (−40 °C to +85 °C), 3000 hours of damp heat (85 °C, 85% relative humidity), 50 humidity-freeze cycles (+85C °C to −40 °C, 85% R.H.) and 600 hours system voltage durability test.
3. Results and discussion
3.1 Thermal cycling
As reported by Mr Nochang Park et al.5 the module power decreases as a result of thermal cycling (TC) stress, due to the formation of cracks between the solder and metal. During the thermal cycling conditions, the PV modules experience a continuous thermal load because of the mismatch between the coefficient of thermal expansion of solder and silicon wafer, which leads to fatigue cracks at the solder interconnection,6 and causes higher series resistance (Rs) and lower fill factor (FF). Compared with traditional backsheet modules, double glass PV modules are more durable, presenting less power degradation due to thermal cycling stress (see Table S1†). As indicated in Fig. 2(a), double glass modules degrade <2.8% over 600 cycles; whereas the backsheet modules degrade over 3.8%. In addition, the Isc and FF have the similar degradation trend in Fig. 2(b) and (c). This suggests that TC stress has a stronger influence on the Pmax degradation of traditional backsheet modules than that of double glass modules.
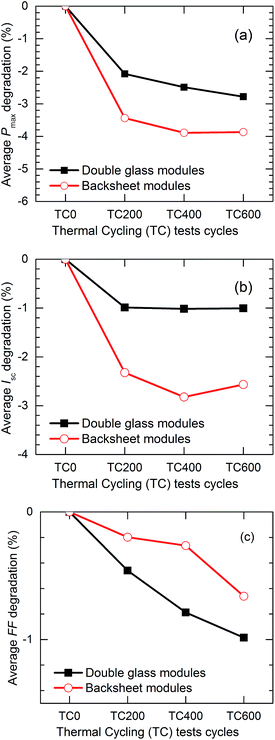 |
| Fig. 2 (a) Pmax degradation, (b) Isc reduction and (c) FF reduction of traditional modules and double glass modules before and after TC stress. | |
3.2 Damp heat
Damp heat (DH) stress is to evaluate the influence of moisture ingress on the PV modules performance. The Pmax degradation results from the loss in FF due to high Rs.7 And the cause of the increase in the series is because the sliver fingers and solder joints could be oxidized or corroded by high thermal and moisture stress.8–10 As seen in Table S2,† the Pmax of traditional backsheet PV modules keep going down as aging continued. In contrast, double glass modules remain relatively constant under the same level of exposures. As displayed in Fig. 3(a), under 3000 hours of damp heat exposure, a significant reduction up to 7.34% in power output is observed in traditional backsheet modules, while double glass module samples perform well within the IEC specification, which is less than 5% power degradation. As shown in Fig. 3(b) and (c), the continuing degradation in Pmax for traditional modules is associated with a drop in Isc and FF. The loss of Isc and FF has significant influence on the degradation of Pmax. It is also can be seen from Fig. 3(d) that after DH3000 stress, the yellowing index of EVA in traditional module is 14.08, which is much worse than that of double glass modules 4.05. This is because more water moisture ingress into traditional backsheet modules accelerated the aging of EVA.13
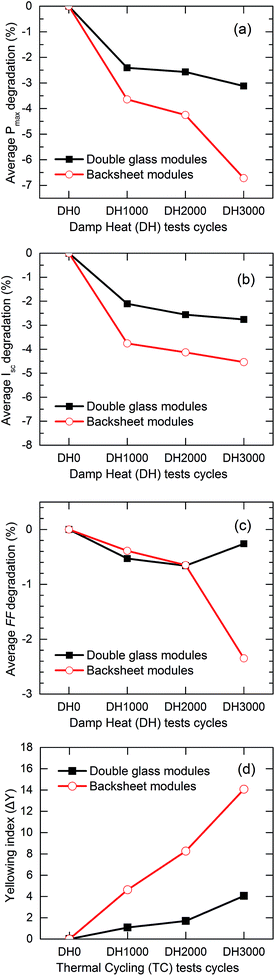 |
| Fig. 3 (a) Pmax degradation, (b) Isc reduction, (c) FF reduction and (d) yellowing index of traditional modules and double glass modules before and after DH stress. (Yellowing index: the yellow index changes before and after thermal aging or light aging, usually represented by ΔY; yellow index: characterization of the degree of a polymer material deviation from the white). | |
3.3 Humidity-freeze stress
Compared to traditional backsheet modules, the almost-zero water moisture penetration into the double glass modules is responsible for the excellent performance under humidity freeze reliability test which was carried out up to 50 cycles (Table S3†). The result indicates that the Pmax degradation of traditional backsheet modules reaches up to 33.73% while that of double glass modules is only 2.72% after humidity-freeze 50 cycles stress test in Fig. 4(a). As presented in Fig. 4(c), the significant change of FF for traditional backsheet modules exhibits a strong correlation with the degradation of Pmax. It is highly suspected that the moisture penetrate the backsheet under humidity-freeze condition, and the acetate moiety of the EVA polymer reacts and forms acetic acid with the presence of water moisture, which can accelerate the corrosion of electrical interconnects such as busbar, collector ribbon, and will further lead to higher Rs and lower FF.14 Additionally, a further study was performed with different WVTR backsheet modules and a strong correlation has been found between WVTR of backsheet and the Pmax degradation under HF10. Modules with lower WVTR have less Pmax degradation, as displayed in Fig. 4(d).
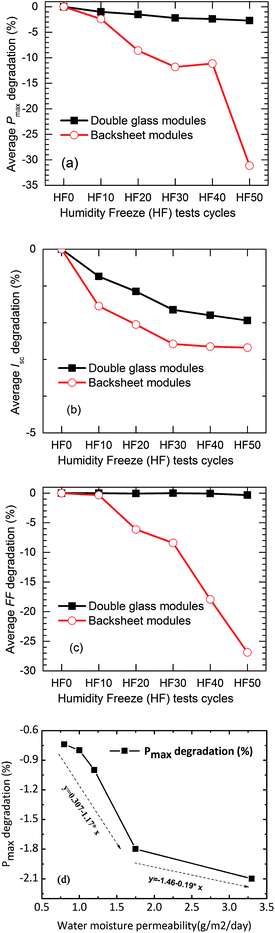 |
| Fig. 4 (a) Pmax degradation, (b) Isc reduction and (c) FF reduction of traditional modules and double glass modules after HF stress, and (d) Pmax degradation of traditional modules with different WVTR backsheet after HF10. | |
3.4 Long-term potential induced degradation (PID)
Higher leakage currents which can be caused by the increase of water moisture through solar panel would make the encapsulation material more conductive11 and it is one of the factors involved in PID. Two traditional modules and two double glass modules were subjected to PID test (−1000 V, 85 °C, 85% R.H. with copper foil on both sides) for 600 hours, which was 6 times above IEC standard (see Table S4†). As to traditional modules with anti-PID material and anti-PID cells which were particularly fabricated with a special anti-PID technology, the average degradation is about 13.3% after 600 hours' test and the observed average Pmax degradation of double glass module is only 2.27% due to superior moisture barrier property, as shown in Fig. 5(a). From Fig. 5(b) and (c), neither much Isc loss for both module types nor big drop in FF on traditional backsheet modules was found, which is because higher WVTR of backsheet allows water diffusion into PV modules leading corrosion of interconnectors, higher sheet resistance and lower FF.
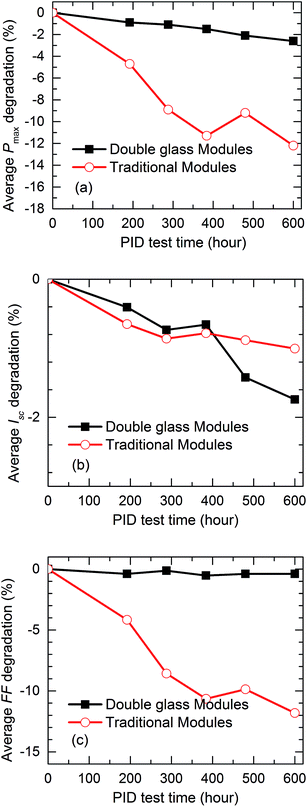 |
| Fig. 5 (a) Pmax degradation, (b) Isc reduction and (c) FF reduction of traditional modules and double glass modules after PID stress. (1000 V, 85 °C, 85% R.H.). | |
3.5 Long-term multiple stress sequential
To understand the limitations of double glass modules, a series of combined sequential stress tests including DH2000, TC600, HF50 were performed on double glass modules and traditional modules (Table S5†). The Pmax degradation of double glass modules and traditional modules are 4.94% and 77.66% respectively, revealing double glass modules exhibit less degradation as illustrated in Fig. 6(a). As indicated in Fig. 6(b) and (c), the great Pmax degradation of traditional modules results from the big loss in FF due to high Rs, and this is because water vapor ingressing into traditional modules causes interconnects corrosion after long-term multiple stress. As the major encapsulation material of traditional PV modules, backsheet plays an important role of insulation, protection, and water proof for solar cells. However, the WVTR of polymeric backsheet is 1∼3 g m−2 day and the whole backsheet is water ingress route and the distance from atmosphere to all solar cells is 0.8–1 mm (thickness of backsheet plus EVA), while the backside glass panel of double glass module is impermeable, the only water transmission route of double glass modules is though the EVA around the edges and the distance from atmosphere to nearest solar cells is 15 mm, the traditional modules also have exactly same route through EVA between front glass and backsheet as well. Water vapor is known as a very reactive species which assists hydrolysis of polymeric material and corrosion of metallic components. After the long-term multiple stress sequential, with the presence of water moisture, the acetate moiety of the EVA polymer reacts and forms acetic acid,12–14 which can cause badly corrosion of electrical interconnects such as silver fingers and collector ribbons15 as showed in Fig. 7(b) and increase series resistance and decrease FF presented in Fig. 6(c). As M. Koehl and X. Han et al. also reported that an increased electrical resistance is associated with corrosion effects of the electrical interconnects.16,17
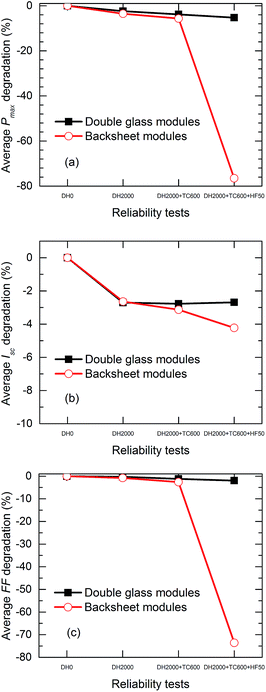 |
| Fig. 6 (a) Pmax degradation, (b) Isc reduction and (c) FF reduction of double glass modules module and traditional modules after long-term multiple sequential stress test. | |
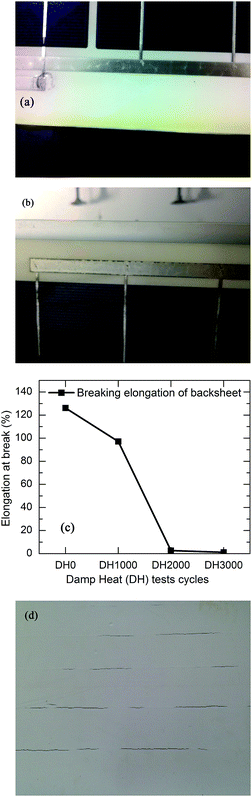 |
| Fig. 7 Collector ribbon appearance of (a) double glass module and (b) traditional module after long-term multiple sequential stress test; (c) the elongation after break and (d) crack of backsheet after DH stress test. | |
The visual inspection check shows that the collector ribbon of traditional backsheet module was oxidized seriously and turned black, while that of double glass module appeared only a little bit yellowing, see Fig. 7(a) and (b). And it can be considered that there was relatively little corrosion occurring in double glass modules after the long-term multiple stress. Fig. 7(c) shows that elongation at break of backsheet remained only 2.7% and 1.2% after DH 2000 and DH 3000 stress test, which would make the backsheet brittle, cracked or even broken easily, as shown in Fig. 7(d), and further result in the oxygen and water moisture transporting into modules and accelerating the aging of EVA and corrosion of solar cells.
High WVTR is responsible for allowing water to enter the PV module which causes corrosion of the inner components. Encapsulants with high WVTR are the reason for corrosion of the solder bonds and copper interconnects.18 Higher WVTR of polymeric backsheet causes the moisture and oxygen to enter traditional modules and accelerate the aging of EVA and produce acetic acid. With the presence of moisture and acetic acid, the sliver finger, ribbon connector and backside electrode are corroded19–21 and led to higher Rs, lower FF, worse yellowing, and more Pmax degradation of traditional backsheet modules. From the above experiment data and analyses, it can be concluded that the key factor of super less Pmax degradation and discoloration of double glass modules after extended long-term reliability test is impermeability of backside glass panel, which protects EVA and solar cells, overcomes and remedies all the quality and reliability issues of traditional PV modules.
4. Conclusions
Different extended stress conditions such as TC 600, DH 3000, PID 600, HF 50 were performed, and the Pmax degradation of traditional backsheet modules is 3.87%, 7.34%, 13.3%, 33.73% while that of double glass modules is 2.78%, 3.12%, 2.27%, 2.72% accordingly. Compared to the traditional backsheet modules, double glass modules have much less Pmax degradation under different stress conditions. From all stresses, HF 50 has much more impact on Pmax degradation of traditional backsheet modules than others and a strong correlation was found between WVTR of backsheet and the Pmax degradation under HF 10 stress. Thereby the penetration of moisture into the module is correlated with decreased performance for a PV module. As the most important factor of double glass PV modules, the impermeability for water vapor of backside glass panel can bring less yellowing and discoloration, less corrosion and oxidation of ribbon connector and backside electrode, and less crack of solar cells, which causes module power less degradation after extended long-term reliability test.
Acknowledgements
The authors would like to thank all the team members for the helps in modules samples fabrication and reliability tests in manufacturing department and test laboratory of Trina Solar. This work is supported by the China National “863” Project under the Project Number of 2015AA050302 and China Natural Science Foundation of Jiangsu Province under the Project Number of BK20140273 and the National Natural Science Foundation of China (61474045).
Notes and references
- S. Yuvarajan, D. Yu and S. Xu, A novel power converter for photovoltaic applications, J. Power Sources, 2004, 135, 327–331 CrossRef CAS PubMed.
- J. Y. Ye, T. Reindl, A. G. Aberle and T. M. Walsh, Performance Degradation of Various PV Module Technologies in Tropical Singapore, IEEE J. Photovolt., 2014, 4, 1288–1294 CrossRef.
- Y. B. Zhang, J. M. Xu, Y. H. Shu, P. Quan, Y. Wang, J. Mao, Y. Y. Gao, C. G. Fu, Z. Q. Feng, P. J. Verlinden, P. X. Yang and J. H. Chu, High-reliability and long-durability wafer-based double-glass module crystalline silicon solar cells with fire-safety class a certification, 28th EU PVSEC, vol. 4, 2013, pp. 31233–3126 Search PubMed.
- IEC 61215, “Crystalline Silicon Terrestrial Photovoltaic Modules-Design Qualification and Type Approval” edition 2 ( 2005).
- N. Park, J. Jeong and C. Han, Estimation of the degradation rate of multi-crystalline silicon photovoltaic module under thermal cycling stress, Microelectron. Reliab., 2014, 54, 1562–1566 CrossRef CAS PubMed.
- R. Subrahmanyan, J. R. Wilcox and C. Y. Li, A Damage Integral Approach to Thermal Fatigue of Solder Joints, IEEE Trans. Acoust., Speech, Signal Process., 1989, 12, 240–252 Search PubMed.
- E. L. Meyer and E. E. van Dyk, Assessing the Reliability and Degradation of Photovoltaic Module Performance Parameters, IEEE Trans. Reliab., 2004, 53, 83–92 CrossRef.
- W. Oh, S. Kim, S. Bae, N. Park, Y. Kang, H. Lee and D. Kim, The degradation of multi-crystalline silicon solar cells after damp heat tests, Microelectron. Reliab., 2014, 54, 2176–2179 CrossRef CAS PubMed.
- N. G. Dhere and N. R. Raravikar, Adhesional shear strength and surface analysis of a PV module deployed in harsh coastal climate, Sol. Energy Mater. Sol. Cells, 2001, 67, 363–367 CrossRef CAS.
- B. Ketola and A. Norris, Degradation Mechanism Investigation of Extended Damp Heat Aged PV Modules, 26th EU PVSEC, Hamburg, 2012, pp. 3523–3528 Search PubMed.
- J. Berghold, O. Frank, H. Hoehne, S. Pingel, B. Richardson and M. Winkler, Potential Induced Degradation of solar cells and panels, 25th EU PVSEC, Valencia, Spain, 2010, pp. 6–10 Search PubMed.
- G. Oreski and G. M. Wallner, Aging mechanisms of polymeric films for PV encapsulation, Sol. Energy, 2005, 79, 612–617 CrossRef CAS PubMed.
- P. Hülsmann and K.-A. Weiss, Simulation of water ingress into PV-modules: IEC-testing, Sol. Energy, 2015, 115, 347–353 CrossRef PubMed.
- M. D. Kempe, G. J. Jorgensen, K. M. Terwilliger, T. J. McMahon, C. E. Kennedy and T. T. Borek, Acetic acid production and glass transition concerns with ethylene-vinyl acetate used in photovoltaic devices, Sol. Energy Mater. Sol. Cells, 2007, 91, 315–329 CrossRef CAS PubMed.
- A. Ndiaya, et al., Degradation of silicon photovoltaic modules: a literature review, Sol. Energy, 2013, 96, 140–151 CrossRef PubMed.
- M. Koehl and P. Bentz, Origin of damp-heat induced cell degradation, Sol. Energy Mater. Sol. Cells, 2013, 116, 49–54 CrossRef PubMed.
- X. Han, Y. Wang, L. Zhu, H. Xiang and H. Zhang, Mechanism study of the electrical performance change of silicon concentrator solar cells immersed in de-ionized water, Energy Convers. Manage., 2012, 53, 1–10 CrossRef CAS PubMed.
- O. Hasan and A. F. M. Arif, Performance and life prediction model for photovoltaic modules Effect of encapsulant constitutive behavior, Sol. Energy Mater. Sol. Cells, 2014, 122, 75–87 CrossRef CAS PubMed.
- T. H. Kim, N. C. Park and D. H. Kim, The effect of moisture on the degradation mechanism of multicrystalline silicon on photovoltaic module proportional to root of the contact resistance, Microelectron. Reliab., 2013, 53, 1823–1827 CrossRef CAS PubMed.
- J. Tao, K. Zhang, C. Zhang, L. Chen, H. Cao, J. Liu, J. Jiang, L. Sun, P. Yanga and J. Chu, A sputtered CdS buffer layer for co-electrodeposited Cu2ZnSnS4 solar cells with 6.6% efficiency, Chem. Commun., 2015, 51, 10337–10340 RSC.
- J. Tao, J. Liu, J. He, K. Zhang, J. Jiang, L. Sun, P. Yanga and J. Chu, Synthesis and characterization of Cu2ZnSnS4 thin films by the sulfurization of co-electrodeposited Cu–Zn–Sn–S precursor layers for solar cell applications, RSC Adv., 2014, 4, 23977–23984 RSC.
Footnote |
† Electronic supplementary information (ESI) available. See DOI: 10.1039/c5ra11224a |
|
This journal is © The Royal Society of Chemistry 2015 |
Click here to see how this site uses Cookies. View our privacy policy here.