DOI:
10.1039/C5RA11215J
(Paper)
RSC Adv., 2015,
5, 65975-65981
The organocatalyzed domino Michael–aldol reaction revisited. Synthesis of enantioenriched 3-hydroxycyclohexanone derivatives by reaction of enals with α,α′-diaryl-substituted acetone†
Received
12th June 2015
, Accepted 28th July 2015
First published on 28th July 2015
Abstract
The reaction of enals with α,α′-diaryl-substituted acetones (pKa > 18) catalyzed by (S)-1-(2-pyrrolidinylmethyl) pyrrolidine provides direct access to enantioenriched 2,5,6-trisubstituted-3-hydroxy cyclohexanones. The process constitutes a highly stereoselective organocatalytic tandem Michael-intramolecular aldol reaction. It has been demonstrated that the stereoselection is dependent on the reaction conditions because only syn diastereoisomers are able to cyclize, and that anti diastereoisomers participate in a retro-Michael process decreasing the enantioselection.
Introduction
Sequential organocatalytic enantioselective Michael addition-cyclization process has been thoroughly exploited in the synthesis of cyclohexanone derivatives with multiple stereocenters.1 This approach is based on an initial diastereo- and enantioselective Michael addition of reagents with prochiral methylene groups, acting as nucleophiles, to α,β-unsaturated carbonyl compounds activated as iminium ions by chiral organocatalysts. The Michael adducts then cyclize to cyclohexanone derivatives in different ways, depending on the nature of the carbonyl group, the substituents, the catalyst, and the reaction conditions.
Two different sets of starting compounds have been used for the preparation of cyclohexanone derivatives depending on the nature of the donor and Michael acceptor. In the first one, alkyl-vinyl ketones, acting as Michael acceptor, react with α-functionalized ketones with a highly acidic single methylene group leading to the Michael adducts, which readily cyclize to the final 3-hydroxy cyclohexanones. The nucleophile for the intramolecular aldol reaction is generated by enolization or enamine formation from the carbonyl of the ketone initially acting as Michael acceptor (eqn (1) in Fig. 1). In this way, 3-hydroxycyclohexanones have been prepared with very good stereoselectivity starting from β-keto esters2 or β-keto sulfones3 and phenylalanine-derived imidazolidine as organocatalysts, but in good yield and moderate stereoselection in the presence of catalytic proline.4
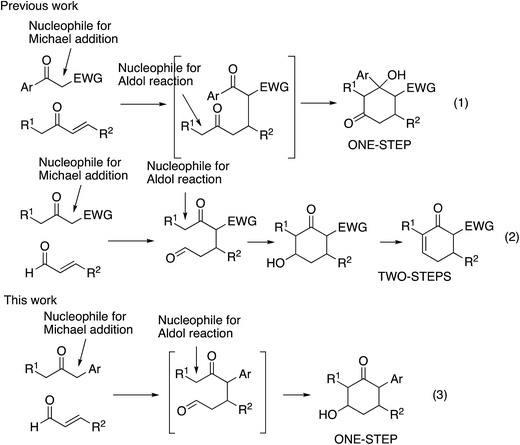 |
| Fig. 1 Representative asymmetric strategies for the synthesis of 3-hydroxy cyclohexanones. | |
Nevertheless, the most popular Michael acceptors used in those processes are α,β-unsaturated aldehydes, which react with ketoesters,5 ketosulfones,6 and phosphoryl-3-oxobutanoates7 leading to cyclohexenones (eqn (2) in Fig. 1). In those cases, the unsaturated ketone is formed in a tandem Michael–Knoevenagel process or in a two-steps protocol promoting the aldol-dehydration process from the initially formed Michael adducts. Methylene cyclohexenones have been also prepared from unsaturated aldehydes and Nazarov reagents as donors,8 or from alkyl-vinyl ketones and aldehydes and benzylamine.9
It has been established that the pKa value of a nucleophile for a direct amine catalytic activation lies between 16 and 17,10 and consequently, only ketones activated by electron withdrawing groups have been used in those transformations.11 Recently, it has been demonstrated12 that 4-nitrophenylacetone constitutes a good nucleophile in the diarylprolinol-catalyzed Michael addition to unsaturated aldehydes, but acidic or basic additives are necessary to get good yields and stereoselectivities. The cyclohexenone derivatives were obtained in a second step after cyclization–dehydration of the Michael adducts. In all those transformations, diarylprolinol ethers have been used as organocatalysts. Those proline-derivatives are able to activate the unsaturated aldehydes by iminium ion formation, but they are unable to promote the necessary activation of the nucleophilic center in the initially formed Michael adducts to promote the cyclization process when weakly acidic ketones were used.
Results and discussion
We envisaged that a bifunctional organocatalysts, with two different nitrogen atoms, would be able to activate the unsaturated aldehyde by forming an iminium ion and to deprotonate the methylene group in the Michael adduct, promoting a tandem Michael addition–intramolecular aldol reaction to 3-hydroxy cyclohexanones (eqn (3), Fig. 1).
Herein, we summarize the first tandem asymmetric Michael addition–aldol reaction of α,β-unsaturated aldehydes and ketones with pKa > 18 catalyzed by (S)-1-(2-pyrrolidinylmethyl) pyrrolidine (1),13 without additional acidic or basic additives. The reaction is highly diastereo- and enantioselective, leading to enantio-enriched 3-hydroxy cyclohexanones with four stereocenters.
Initially, we studied the effect of the solvent and temperature in the reaction of dibenzyl ketone (2a) and cinnamaldehyde (3a) catalyzed by 1, and the results are collected in Table 1. The screening showed that at rt the highest reaction rate and the best yield were obtained in methanol as a solvent (entry 1), but a very complex mixture of diastereoisomers was obtained. The reaction also proceeded in ethereal solvents, toluene, and DCM yielding mixtures of three different diastereomeric 2,5,6-triphenyl-3-hydroxy cyclohexanones (entries 2–6). No improvement in the diastereoselection was observed by lowering the temperature when the reactions were carried out in DCM as solvent (entries 7 and 8), but a mixture 7/3 of only two diastereoisomers was obtained at 0 °C in diethyl ether (entry 9). Fortunately, excellent diastereo- and enantioselectivities were observed for the reaction in Et2O at −18 °C (entry 10) although at expenses of diminishing the yield of the cyclized product. Increasing the reaction time to 240 h in that conditions did not increase the yield of the cyclization product. Lowering the temperature to −35 °C did not improve the stereoselection, but increased the reaction time to ten days (entry 11) (Scheme 1).
Table 1 Effect of the solvent and temperature on the tandem Michael-aldol reaction of 2a and 3a catalyzed by 1
Entry |
Solvent |
T (°C) |
Time (h) |
Yield 4aa,a (dr)b [ee]c |
Yields refer to isolated compounds. Determined by 1H-NMR in the reaction mixture. Determined by chiral HPLC, and refers to the major diastereoisomer. Different amounts of the Michael adducts were detected in the reaction mixtures. |
1 |
MeOH |
rt |
10 |
75% (complex mixture) |
2 |
Toluene |
rt |
36 |
50% (2 : 2 : 1) [nd] |
3 |
THF |
rt |
72 |
50% (4 : 2 : 1) [nd] |
4 |
TBME |
rt |
38 |
50% (4 : 2 : 1) [nd] |
5 |
DCM |
rt |
24 |
70% (4 : 3 : 1) [nd] |
6 |
Et2O |
rt |
38 |
60% (4 : 2 : 1) [nd] |
7 |
DCM |
0 |
72 |
67% (4 : 2 : 1) [66]d |
8 |
DCM |
−18 |
72 |
60% (4 : 2 : 1) [78]d |
9 |
Et2O |
0 |
96 |
60% (7 : 3) [78]d |
10 |
Et2O |
−18 |
144 |
53% (97 : 3) [82]d |
11 |
Et2O |
−35 |
240 |
50% (97 : 3) [82]d |
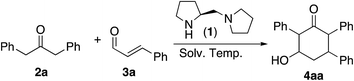 |
| Scheme 1 Organocatalyzed reaction of dibenzyl ketone with cinnamaldehyde. | |
It is important to note that only cyclization products were observed when the reaction was carried out at rt, whereas a mixture of cyclohexanone derivatives 4aa and anti-Michael adducts 5aa was detected when the reaction was performed at low temperature (Scheme 2).
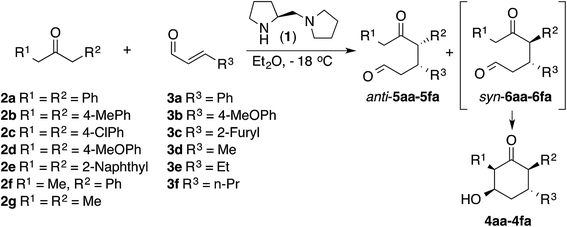 |
| Scheme 2 Domino Michael-intramolecular aldol reaction of different enals and ketones catalyzed by diamine 1. | |
Then, we explored the generality of the reaction of different β-substituted unsaturated aldehydes (3a–f) with dibenzyl ketone 2a in Et2O at −18 °C in the presence of 20 mol% of catalyst 1 without additional acidic or basic additives, and the results are summarized in Scheme 2 and Table 2 (entries 1–6). In all cases mixtures of the anti-Michael adducts 5aa–5af and 3-hydroxy-2,5,6-trisubstituted cyclohexanones 4aa–4af were obtained as single diastereoisomers, but with enantioselectivities that are dependent on the nature of the substituent at β-position in the unsaturated aldehyde. The stereochemistry of 4aa was determined by single crystal X-ray analysis,14 and generalized for all the 3-hydroxy cyclohexanone derivatives, and indicates that they result from the cyclization of syn diastereoisomers 6aa–6af, which were not detected in the reaction mixtures.
Table 2 Synthesis of 2,5,6-trisubstituted-3-hydroxy cyclohexanones from unsaturated aldehydes and ketones catalyzed by (1)
Entry |
Reagents |
Time (h) |
Products |
2 (R1, R2) |
3 (R3) |
5 (yield),a [ee]b |
4 (yield),a [ee],b dec |
Yields refer to isolated compounds. Determined by chiral HPLC. Determined by 1H-NMR of the reaction mixtures, >96 means that only a single diastereoisomer was detected by 1H-NMR. 7% of 6db was detected in the mixture. The reaction was carried out at rt. 16% of 6fa (78% ee) was isolated. |
1 |
R1 = R2 = Ph |
Ph |
144 |
5aa; (20), [46] |
4aa; (53), [83], >96 |
2 |
R1 = R2 = Ph |
4-MeOPh |
192 |
5ab; (16), [38] |
4ab; (60), [80], >96 |
3 |
R1 = R2 = Ph |
2-furyl |
192 |
5ac; (26), [26] |
4ac; (55), [66], >96 |
4 |
R1 = R2 = Ph |
Me |
336 |
5ad; (49), [44] |
4ad; (40), [52], 80 |
5 |
R1 = R2 = Ph |
Et |
408 |
5ae; (44), [40] |
4ae; (40), [68], 50 |
6 |
R1 = R2 = Ph |
n-Pr |
408 |
5af; (42), [32] |
4af; (40), [46], 40 |
7 |
R1 = R2 = 4-MePh |
4-MeOPh |
144 |
5bb; (16), [46] |
4bb; (50), [76], >96 |
8 |
R1 = R2 = 4-ClPh |
4-MeOPH |
144 |
5cb; (17), [6] |
4cb; (48), [70], >96 |
9 |
R1 = R2 = 4-MeOPh |
4-MeOPh |
240 |
5db; (78), [14]d |
|
10 |
R1 = R2 = 2-naphtyl |
4-MeOPh |
144 |
5eb; (18), [38] |
4eb; (48), [84], >96 |
11 |
R1 = Me, R2 = Ph |
Ph |
72e |
5fa; (64), [30]f |
|
12 |
R1 = R2 = Me |
Ph |
240e |
No reaction |
As a general feature, the observed enantioselectivities were much better for the formation of the syn diastereoisomers (isolated as hydroxy cyclohexanones 4) than for the anti diastereoisomers.
Furthermore, different ketones (2b–g) were tested towards cinnamaldehyde (3a) or p-methoxy cinnamaldehyde (3b) (entries 7–12 in Table 2). Notably, the behavior of these ketones is highly dependent on the substitution pattern. Thus, 1,3-di-p-tolylpropan-2-one (2b), 1,3-bis(4-chlorophenyl)propan-2-one (2c), and 1,3-di(naphtalen-2-yl)propan-2-one (2e) ketones behave in a similar way than dibenzyl ketone (2a) do, leading to the corresponding hydroxy-cyclohexanones as major products in moderate yield, with excellent diastereoselectivity and moderate to good enantioselectivity (entries 7, 8, and 10 in Table 2). On the contrary, the initial addition of 1,3-bis(4-methoxyphenyl) propan-2-one (2d) to enal 3b was highly diastereoselective, giving the anti Michael adduct 5db in good yield although poor enantioselectivity (entry 9 in Table 2) and only 7% of syn diastereoisomer (syn-6bd) was detected in the reaction mixture. The reaction was also very sensitive to the acidity of the ketone used as nucleophile. Benzyl ethyl ketone (2f) only yielded a mixture of anti-5fa (64%, 30% ee) and syn-6fa (16%, 78% ee) Michael adducts because the methylene group of the ethyl substituent is not acidic enough (pKa 24.4)15 to be deprotonated by the catalyst to promote the cyclization process (entry 11 in Table 2). Additionally, 3-pentanone (2g), with pKa 27.1,15 did not react with cinnamaldehyde after 240 h at rt (entry 12 in Table 2).
The incorporation of a nitro substituent at para position in the phenyl ring highly increases the acidity at the benzylic position11a,16,17 and, in fact, the much more acidic 1,3-bis(4-nitrophenyl) propan-2-one (2h) behaves in a quite different way, leading to the Michael–Knoevenagel adduct instead of the Michael–aldol product. It reacted with 3b yielding cyclohexenone 7hb in excellent yield and near total enantioselectivity after 17 h at −18 °C (eqn (1) in Scheme 3). After some control experiment, we were unable to detect the corresponding 3-hydroxy cyclohexanone derivative in the reaction mixture, indicating that the increase of the acidity of methylene protons allows that the initially formed Michael adduct easily epimerizes and immediately cyclizes and dehydrates to the cyclohexenone derivative 7hb. Finally, 1,3-bis(2-thienyl) propan-2-one (2i) readily reacted with 3b yielding 4ib as a mixture of diastereoisomers at C-2 and/or C-3, which spontaneously dehydrated at rt to a single diastereoisomer of cyclohexenone 7ib, but in moderate enantioselectivity (eqn (2) in Scheme 3).
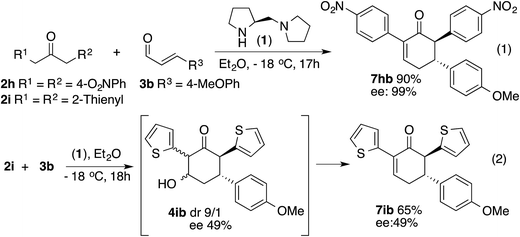 |
| Scheme 3 Michael–aldol-dehydration leading to cyclohexenones. | |
The first step of the process is well established, and accepted that the Michael addition occurs by activation of the enal by formation of an iminium ion, followed by addition of the nucleophile leading to a mixture of enantioenriched syn and anti diastereoisomers. The evolution of the Michael adducts is dependent on the pKa of the methine protons and the reaction conditions. It has been previously described that, for very acidic substrates, anti adduct totally epimerizes to the syn diastereoisomer that quickly cyclizes to hydroxy cyclohexanone derivatives by intramolecular aldol reaction2,3 or participates in an enolization process and subsequent heterocyclization.18 In our case, the epimerization is a very slow process or it does not occur at −18 °C, because a mixture of cyclized products 4, resulting form a very quickly cyclization of the syn diastereoisomers, and anti Michael adducts 5 were isolated. On the contrary, the epimerization takes place above 0 °C, isolating mixtures of cyclized products, but with lower enantioselectivity (compare entries 9 and 10 in Table 1).
We suspect that above 0 °C catalyst 1 was able to promote not only the epimerization of the anti diastereoisomer but also a retro-Michael reaction, thus eroding the ee. To shed light on the observed results we reacted dibenzylketone (2a) with p-methoxy benzaldehyde (3b), at 35 °C for 144 h, in the presence of racemic prolinol derivative 8 as catalyst (60 mol%), leading to a racemic mixture of anti-5ab and syn-6ab (1
:
3) in 20% yield. This mixture was dissolved in diethyl ether, cooled to −18 °C, and stirred for 100 h in the presence of catalyst 1. Chiral HPLC analysis of the final mixture showed that rac-syn-6ab was transformed into rac-3-hydroxy cyclohexanone 4ab, whereas anti-5ab remains in the mixture but partially deracemized in favor of (3S,4R) enantiomer (20% ee) (Scheme 4).
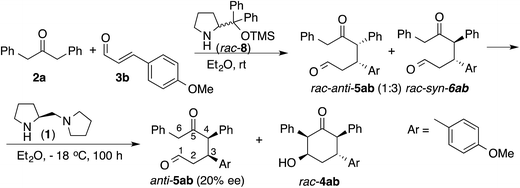 |
| Scheme 4 Intramolecular aldol or de-racemization of syn- or anti-Michael adducts. | |
This fact confirms that, under the studied conditions, only the rac-syn-diastereoisomer quickly cyclizes to the rac-hydroxy cyclohexanone, and more important, that anti-diastereoisomer deracemizes via retro-Michael reaction.19 The retro-Michael process could also explain that the catalytic process is less enantioselective for the formation of the anti- than for the syn-diastereoisomers.
With this information in mind, we propose a pathway for the reaction as summarized in Scheme 5. Bifunctional diamine 1 is able to promote the activation of the enal by formation of an iminium ion leading to a mixture of anti-5 and syn-6 diastereoisomeric Michael adducts, and the enolization of the syn-intermediate and subsequent intramolecular aldol reaction. This fact has also been observed in reactions catalyzed by prolinol derivatives (8 and related compounds) but only for ketones activated by strong electron withdrawing substituents. These diastereoisomers can equilibrate by epimerization at the stereogenic C-4 above 0 °C but not, or very slowly, at −18 °C. At that temperature, the evolution of diastereoisomers is controlled by the stereogenic center formed in the Michael reaction.2 Thus, the syn diastereoisomer 6, with all large substituent at equatorial position, suffers quickly enolization and cyclization promoted by catalyst 1 to the 3-hydroxy cyclohexanone derivative 4, whereas anti isomer does not cyclize and remains in the reaction mixture participating in a retro-Michael–Michael equilibrium responsible for the low enantioselection for this product.
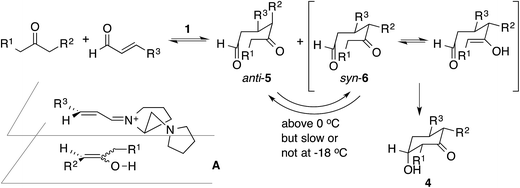 |
| Scheme 5 Proposed pathway for the studied reaction. | |
With respect to the stereochemistry in the Michael adducts, the major enantiomer of the syn-diastereoisomer could be formed by attack of the re-face of enol approaching to the re-face of the iminium ion20 (A in Scheme 5).
Conclusions
In summary, we have described a synthesis of enantioenriched 3-hydroxy-2,5,6-trisubstituted cyclohexanones although in moderate yield and moderate to good enantioselectivity. More important, the obtained results demonstrate that, contrary to previously described, ketones with pKa > 18 can be used as nucleophiles in organocatalyzed Michael addition to α,β-unsaturated aldehydes. It is necessary that the catalyst of choice be able to activate both the electrophile by formation of an iminium ion and the intermediate Michael adducts by enolization of the ketone. The behavior of the obtained diastereoisomers is dependent on the relative stereochemistry. At −18 °C, syn diastereoisomers quickly cyclize to enantioenriched 2,5,6-trisubstituted 3-hydroxy cyclohexanones, whereas anti diastereoisomers remain in the mixture and partially racemize via retro-Michael reaction.
Experimental
Chemical yields refer to pure isolated substances. TLC analysis was performed on glass-backed plates coated with silica gel 60 and an F254 indicator, and visualized by staining with phosphomolybdic acid solution. Chiral HPLC analysis was performed using different Daicel Chiralcel Columns. UV detection was monitored at 220 nm or at 254 nm. 1H NMR (400 MHz or 500 Mz) and 13C NMR (100 MHz or 126 Mz) spectra were recorded in CDCl3. Chemical shifts for protons are reported in ppm from TMS. Chemical shifts for carbons are reported in ppm from tetramethylsilane and are referenced to the carbon resonance of the solvent. Data are reported as follows: chemical shift, multiplicity (s = singlet, d = doublet, t = triplet, q = quartet, m = multiplet, br = broad), coupling constants in Hertz, and integration. Specific rotations were measured using a 5 mL cell with a 1 dm path length, and a sodium lamp, and concentration is given in g per 100 mL. Melting points were obtained with open capillary tubes and are uncorrected. Flash chromatography was carried out using silica gel (230–240 mesh).
General procedure
To a solution of aldehyde (0.30 mmol) and ketone (1.5 equivalents, 0.45 mmol) in 5 mL of diethyl ether cooled to −18 °C was added the catalyst 1 (0.06 mmol, 0.2 equiv.), and the solution stirred at that temperature for the time indicated in Table 2. Then the mixture was filtered through a pad of celite, the solvent was evaporated under vacuum and the residue was purified by flash chromatography on silica gel (hexane/ethyl acetate) to yield the desired compound.
(2R,3R,5R,6R)-3-Hydroxy-2,5,6-triphenylcyclohexanone (4aa). White solid; mp 150–153 °C (hexane). [α]25D −49.8, (c = 1, CHCl3), (83% ee). 1H NMR (500 MHz, CDCl3) δ 2.14 (s, 1H), 2.38–2.58 (m, 2H), 3.96 (dd, J = 9.0, 2.8 Hz, 1H), 3.97 (d, J = 1.9 Hz, 1H), 4.14 (d, J = 2.5 Hz, 1H), 4.41–4.61 (m, 1H), 6.78–7.55 (m, 15H); 13C NMR (126 MHz, CDCl3) δ 41.2, 46.0, 61.8, 63.7, 74.1, 126.6, 126.7, 127.5, 127.6 (2C), 127.9 (2C), 128.5 (2C), 128.6 (2C), 129.7 (2C), 130.0 (2C), 136.0, 136.6, 142.5, 205.3; HPLC (Chiralpak AD-H hexane/iPrOH = 80
:
20, 1.0 mL min−1 λ = 220 nm) tR = 18.8 min (major), tR = 11.1 min (minor); IR: 3530, 3033, 2920, 1953, 1706, 1488, 1307, 1070 cm−1; HRMS (ESI): m/z = 365.1505, calculated for C24H22NaO2+: 365.1512.
(2R,3R,5R,6R)-3-Hydroxy-5-(4-methoxyphenyl)-2,6-diphenylcyclohexanone (4ab). White solid; mp 79–82 °C (hexane); [α]25D −32.0, (c = 1, CHCl3), (80% ee); 1H NMR (400 MHz, CDCl3) δ 2.14 (s, 1H), 2.39–2.51 (m, 2H), 3.71 (s, 3H), 3.86–3.94 (m, 2H), 4.07–4.16 (m, 1H), 4.50 (m, 1H), 6.57–7.19 (m, 9H), 7.28–7.51 (m, 5H); 13C NMR (101 MHz, CDCl3) δ 29.8, 41.3, 45.2, 55.2, 61.8, 63.9, 74.0, 76.8, 77.1, 77.4, 113.8 (2C), 126.6, 127.5, 127.9, 128.5, 128.6, 129.7, 130.0 (2C), 134.7, 136.1, 136.8, 158.0, 205.4; HPLC (Chiralpak AD-H hexane/iPrOH = 80
:
20, 1.0 mL min−1 λ = 220 nm) tR = 27.2 min (major), tR = 14.2 min (minor); IR: 3493, 2920, 2853, 1710, 1509, 1245, cm−1; HRMS (ESI): m/z = 395.1608, calculated for C25H24NaO2+: 395.1618.
(2R,3R,5R,6R)-3-(Furan-2-yl)-5-hydroxy-2,6-diphenylcyclohexanone (4ac). White solid; mp 160–163 °C; [α]25D −51.5, (c = 1.3, CHCl3), (66% ee); 1H NMR (500 MHz, CDCl3) δ 2.18 (s, 1H), 2.38–2.53 (m, 1H), 2.56–2.68 (m, 1H), 4.03 (dd, J = 4.1 Hz, 1H), 4.07 (d, J = 2.4 Hz, 1H), 4.48 (d, J = 2.7 Hz, 1H), 4.48 (d, J = 2.7 Hz, 1H), 5.73 (d, J = 3.2 Hz, 1H), 6.09 (dd, J = 3.1, 1.9 Hz, 1H), 7.51–6.91 (m, 11H); 13C NMR (126 MHz, CDCl3) δ 38.2, 40.0, 61.6, 61.7, 73.4, 106.1, 109.9, 126.8, 127.5, 127.9 (2C), 128.5 (2C), 129.2 (2C), 129.9 (2C), 135.9, 136.8, 141.1, 155.3, 204.7; HPLC (Chiralpak AD-H hexane/iPrOH = 80
:
20, 1.0 mL min−1 λ = 220 nm), tR = 17.6 min (major), tR = 9.8 min (minor); IR: 3540, 3028, 2914, 2083, 1979, 1711, 1499, 1297 cm−1; HRMS (ESI): m/z = 355.1300, calculated for C22H20NaO3+: 355.1305.
(2R,3R,5R,6R)-3-Hydroxy-5-methyl-2,6-diphenylcyclohexanone (4ad). White solid; mp 55–58 °C (hexane); [α]25D +2.2, (c = 0.6, CHCl3), (52% ee); 1H NMR (500 MHz, CDCl3) δ 0.90 (d, J = 6.5 Hz, 3H), 2.02 (m, 3H), 2.31 (dd, J = 10.7, 7.0 Hz, 1H), 3.35 (d, J = 11.8 Hz, 1H), 3.96 (d, J = 2.6 Hz, 1H), 4.43 (sb, 1H), 7.18–7.51 (m, 10H); 13C NMR (126 MHz, CDCl3) δ 21.0, 34.7, 41.1, 61.6, 65.4, 74.2, 127.0, 127.5 (2C), 128.3 (2C), 128.6, 129.6 (2C), 130.1 (2C), 136.1, 137.4, 205.8; HPLC (Chiralpak AD-H hexane/iPrOH = 90
:
10, 1.0 mL min−1 λ = 220 nm) tR = 54.4 min (major), tR = 24.0 min (minor); IR: 3499, 2920, 2093, 1948, 1710, 1494, 1425, 1034 cm−1; HRMS (EI): m/z = 280.1362, calculated for C19H20O2: 280.1463.
(2R,3R,5R,6R)-3-Ethyl-5-hydroxy-2,6-diphenylcyclohexanone (4ae). Colorless oil; [α]25D +36.0, (c = 1, CHCl3), (68% ee); 1H NMR (500 MHz, CDCl3) δ 0.86 (t, 3H), 1.95–2.03 (m, 3H), 2.22 (m, 1H), 2.34–2.46 (m, 1H), 2.62–2.74 (m, 1H), 3.46 (d, J = 12.0 Hz, 1H), 3.96 (d, J = 2.3 Hz, 1H), 4.48 (bs, 1H), 6.48–7.57 (m, 10H); 13C NMR (126 MHz, CDCl3) δ 10.4, 25.4, 26.9, 40.2, 61.7, 63.5, 74.2, 126.9, 127.2, 127.3, 127.4, 128.3, 128.5, 128.6, 128.8, 129.6, 130.0, 136.0, 137.3, 206.0. HPLC (Chiralpak AD-H hexane/iPrOH = 80
:
20, 1.0 mL min−1 λ = 220 nm) tR = 14.7 min (major), tR = 11.3 min (minor); IR: 3473, 2966, 2925, 2868, 1953, 1716, 1493, 1049, 693 cm−1; HRMS (ESI): m/z = 317.1512, calculated for C20H22NaO2+: 317.1514.
(2R,3R,5R,6R)-3-Hydroxy-2,6-diphenyl-5-propylcyclohexanone (4af). Obtained as an unseparable mixture of diastereoisomers. 46% ee for the syn-diastereoisomer. Colorless oil; 1H NMR (500 MHz, CDCl3) δ 0.85 (t, 3H), 1.26–1.44 (m, 4H), 1.91–2.05 (m, 1H), 2.19 (m, 1H), 2.43 (m, 1H), 2.81 (m, 1H), 3.94 (d, J = 6.1 Hz, 1H), 4.12 (d, J = 2.8 Hz, 1H), 4.30–4.62 (m, 1H), 7.22–7.53 (m, 10H); 13C NMR (126 MHz, CDCl3) δ 14.2, 20.4, 34.7, 35.0, 35.7, 57.9, 61.1, 73.7, 127.3, 127.4, 128.3, 128.5, 128.6, 128.8, 129.6 (2C), 130.0 (2C), 136.0, 136.6, 207.2. HPLC (Chiralpak AD-H hexane/iPrOH = 80
:
20, 1.0 mL min−1 λ = 220 nm) tR = 13.2, min (major), tR = 9.2 min (minor); IR: 3454, 3033, 2960, 2929, 2856, 1957, 1879, 1707, 1489, 1453, 704 cm−1; HRMS (ESI): m/z = 331.1674, calculated for C21H24NaO2+: 331.1674.
(2R,3R,5R,6R)-3-Hydroxy-5-(4-methoxyphenyl)-2,6-dip-tolylcyclohexanone (4bb). White solid; mp 134–137 °C [α]25D +10.6, (c = 0.8, CHCl3), (76% ee); 1H NMR (500 MHz, CDCl3) δ 2.22 (s, 3H), 2.35 (m, 4H), 2.42 (m, 2H), 3.72 (s, 3H), 3.89 (m, 2H), 4.08 (d, J = 2.6 Hz, 1H), 4.45 (q, 1H), 6.37–7.59 (m, 12H); 13C NMR (126 MHz, CDCl3) δ 21.2 (2C), 29.8, 41.5, 44.9, 55.2, 61.4, 63.4, 74.1, 76.9, 113.8 (2C), 128.5, 128.7, 129.3, 129.4, 129.8 (2C), 133.1 (2C), 133.7 (2C), 135.0, 135.9, 137.1, 157.9, 205.7. HPLC (Chiralpak AD-H hexane/iPrOH = 80
:
20, 1.0 mL min−1 λ = 220 nm) tR = 21.8 min (major) tR = 12.1 min (minor); IR: 3426, 2920, 2853, 1716, 1514, 1380, 1251 cm−1; HRMS (ESI): m/z = 423.1919, calculated for C27H28NaO3+: 423.1930.
(2R,3R,5R,6R)-2,6-Bis(4-chlorophenyl)-3-hydroxy-5-(4-methoxyphenyl) cyclohexanone (4cb). White solid; mp 100–103 °C (hexane); [α]25D +12.0, (c = 0.5, CHCl3), (70% ee); 1H NMR (500 MHz, CDCl3) δ 2.20 (s, 1H), 2.29–2.58 (m, 2H), 3.72 (s, 3H), 3.81 (m, 1H), 3.87 (d, 1H), 4.06 (d, J = 2.3 Hz, 1H), 4.45 (bs, 1H), 6.24–7.52 (m, 12H); 13C NMR (126 MHz, CDCl3) δ 41.5, 45.4, 55.2, 61.1, 63.3, 73.8, 113.9, 128.2 (2C), 128.4 (2C), 128.6 (2C), 130.9 (2C), 131.5 (2C), 132.4 (2C), 133.5, 134.1, 134.4, 135.2, 158.2, 204.8. HPLC (Chiralpak AD-H hexane/iPrOH = 80
:
20, 1.0 mL min−1 λ = 220 nm) tR = 15.0 min (major), tR = 13.6 min (minor); IR: 3406, 2925, 1716, 1514, 1488, 1251, 724 cm−1; HRMS (ESI): m/z = 463.0826, calculated for C25H22Cl2NaO3+: 463.0838.
(2R,3R,5R,6R)-3-Hydroxy-5-(4-methoxyphenyl)-2,6-di(naphthalen-2-yl) cyclohexanone (4eb). White solid; mp 90–93 °C (hexane); [α]25D −28.1, (c = 1, CHCl3), (84% ee); 1H NMR (500 MHz, CDCl3) δ 2.33 (s, 1H), 2.42–2.62 (m, 2H), 3.66 (s, 3H), 4.02–4.16 (m, 1H), 4.14 (d, 1H), 4.31 (d, J = 2.2 Hz, 1H), 4.56 (bs, 1H), 6.67 (d, J = 8.7 Hz, 2H), 7.10 (d, J = 8.7 Hz, 2H), 7.18–8.15 (m, 14H); 13C NMR (126 MHz, CDCl3) δ 41.6, 45.0, 55.1, 61.8, 64.0, 74.0, 113.9 (2C), 125.4, 125.5, 126.0, 126.1, 127.4, 127.5 (2C), 127.6, 127.6, 127.8, 128.1, 128.1, 128.2, 128.4, 128.8, 128.9, 132.5, 132.7, 133.3, 133.4, 133.6, 134.5, 134.6, 158.0, 205.7; HPLC (Chiralpak AD-H hexane/iPrOH = 80
:
20, 1.0 mL min−1 λ = 220 nm) tR = 46.0 min (major), tR = 42.4 min (minor); IR: 3416, 3049, 2920, 1710, 1514, 1251, 1018, 811 cm−1; HRMS (ESI): m/z = 495.1926, calculated for C33H28NaO3+: 495.1930.
(5R,6R)-5-(4-Methoxyphenyl)-2,6-bis(4-nitrophenyl)cyclohex-2-enone (7hb). Yellow solid; mp 161–165 °C (hexane); [α]25D −44.9, (c = 1, CHCl3), (99% ee); 1H NMR (500 MHz, CDCl3) δ 2.82–3.13 (m, 2H), 3.58–3.75 (m, 1H), 3.72 (s, 3H), 4.16 (d, J = 13.3 Hz, 1H), 6.73 (d, J = 8.7 Hz, 2H), 7.01 (d, J = 8.7 Hz, 2H), 7.16 (d, J = 8.8 Hz, 2H), 7.32 (dd, J = 5.2, 3.4 Hz, 1H), 7.56 (d, J = 8.8 Hz, 2H), 8.05 (d, J = 8.7 Hz, 2H), 8.22 (d, J = 8.9 Hz, 2H); 13C NMR (126 MHz, CDCl3) δ 35.8, 47.1, 49.0, 55.2, 60.7, 114.2 (2C), 123.3 (2C), 123.4, 123.9, 128.4 (2C), 129.6, 130.4, 130.5, 132.5, 138.6, 142.3, 145.2, 146.8, 147.5, 148.7, 158.7, 195.6; HPLC (Chiralpak AD-H column hexane/iPrOH = 80
:
20, 1.0 mL min−1 λ = 254 nm) tR = 30.6 min (major), tR = 16.1 min (minor); IR: 2930, 2832, 1674, 1597, 1509, 1338, 1251, 1106 cm−1; HRMS (ESI): m/z = 467.1217, calculated for C25H20N2NaO6+: 467.1213.
(5R,6S)-5-(4-Methoxyphenyl)-2,6-di(thien-2-yl)cyclohex-2-enone (7ib). Yellow solid; mp 190–192 °C (hexane); [α]25D −3.6, (c = 0.8, CHCl3), (49% ee); 1H NMR (400 MHz, CDCl3) δ 2.91 (m, 2H), 3.63 (ddd, J = 11.3, 9.2, 5.4 Hz, 1H), 3.75 (s, 3H), 4.31 (d, J = 11.4 Hz, 1H), 6.76 (m, 4H), 7.03 (dd, J = 5.1, 3.7 Hz, 1H), 7.12 (m, 3H), 7.36 (m, 3H); 13C NMR (101 MHz, CDCl3) δ 34.9, 47.5, 55.3, 55.4, 114.0 (2C), 124.7, 125.6, 126.4, 126.6, 126.9, 126.9, 128.5, 133.0, 134.1, 136.8, 139.8 (2C), 143.0, 158.5, 195.1; HPLC (Chiralpak AD-H column hexane/iPrOH = 80
:
20, 1.0 mL min−1 λ = 254 nm) tR = 16.7 min (major), tR = 29.7 min (minor); IR: 3098, 2922.8, 2853.8, 1675, 1511, 1246, 688 cm−1; HRMS (ESI): m/z = 367.0824, calculated for C21H20O2S2+: 367.0826.
Acknowledgements
We acknowledge the financial support provided by the Spanish MINECO (Projects CTQ 2011-28487, and CTQ2014-59870 P) and Junta de Castilla y León (Project VA064U13). J. O. G.-P. thanks the Universidad de Valladolid for a predoctoral fellowship.
References
- For some recent reviews see:
(a) S. Goudedranche, W. Raimondi, X. Bugaut, T. Constantieux, D. Bonne and J. Rodriguez, Synthesis, 2013, 45, 1909 CrossRef CAS;
(b) H. Pellissier, Adv. Synth. Catal., 2012, 354, 237 CrossRef CAS PubMed;
(c) A. Moyano and R. Rios, Chem. Rev., 2011, 111, 4703 CrossRef CAS PubMed;
(d) J. L. Vicario, D. Badía and L. Carrillo, Synthesis, 2007, 2065 CrossRef CAS;
(e) S. B. Tsogoeva, Eur. J. Org. Chem., 2007, 170 Search PubMed;
(f) D. Almasi, D. A. Alonso and C. Nájera, Tetrahedron: Asymmetry, 2007, 18, 299 CrossRef CAS PubMed;
(g) D. Enders, C. Grondal and M. R. H. Hüttl, Angew. Chem., Int. Ed., 2007, 46, 1570 CrossRef CAS PubMed.
- N. Halland, P. S. Aburel and K. A. Jörgensen, Angew. Chem., Int. Ed., 2004, 43, 1272 CAS.
- J. Pulkkinen, P. S. Aburel, N. Halland and K. A. Jörgensen, Adv. Synth. Catal., 2004, 346, 1077 CrossRef CAS PubMed.
- D. Gryko, Tetrahedron: Asymmetry, 2005, 16, 1377 CrossRef CAS PubMed.
-
(a) E. Alza, S. Sayalero, S. C. Cambeiro, R. Martín-Rapún, P. O. Miranda and M. A. Pericàs, Synlett, 2011, 464 CAS;
(b) Y. Hayashi, M. Toyoshima, H. Gotoh and H. Ishikawa, Org. Lett., 2009, 11, 45 CrossRef CAS PubMed;
(c) P. Bolze, G. Dickmeiss and K. A. Jörgensen, Org. Lett., 2008, 10, 3753 CrossRef CAS PubMed;
(d) A. Carlone, M. Marigo, C. North, A. Landa and K. A. Jörgensen, Chem. Commun., 2006, 4928 RSC.
- J. Alemán, V. Marcos, L. Marzo and J. L. García-Ruano, Eur. J. Org. Chem., 2010, 4482 Search PubMed.
- L. Albrecht, B. Richter, C. Vila, H. Krawczyk and K. A. Jörgensen, Chem.–Eur. J., 2009, 15, 3093 CrossRef CAS PubMed.
-
(a) J. Peña, A. B. Antón, R. F. Moro, I. Marcos, N. M. Garrido and D. Díez, Tetrahedron, 2011, 67, 8331 CrossRef PubMed;
(b) S. Cabrera, J. Alemán, P. Bolze, S. Bertelsen and K. A. Jörgensen, Angew. Chem., Int. Ed., 2008, 47, 121 CrossRef CAS PubMed.
- H. Yang and R. G. Carter, Org. Lett., 2010, 12, 3108 CrossRef CAS PubMed.
- D. A. Alonso, S. Kitagaki, N. Utsumi and C. F. Barbas III, Angew. Chem., Int. Ed., 2008, 47, 4588 CrossRef CAS PubMed.
- For some exceptions, leading to Michael adducts, see:
(a) S. Vera, M. Marigo, E. C. Escudero-Adán and P. Melchiorre, Synlett, 2011, 489 CAS;
(b) C. Cassani, X. Tian, E. C. Escudero-Adán and P. Melchiorre, Chem. Commun., 2011, 47, 233 RSC;
(c) H. Gotoh, H. Ishikawa and Y. Hayashi, Org. Lett., 2007, 9, 5307 CrossRef CAS PubMed;
(d) C. Palomo, A. Landa, A. Mielgo, M. Oiarbide, A. Puente and S. Vera, Angew. Chem., Int. Ed., 2007, 46, 8431 CrossRef CAS PubMed.
- S. Duce, M. Jorge, I. Alonso, J. L. García Ruano and M. B. Cid, Org. Biomol.
Chem., 2011, 9, 8253 CAS.
-
(a) S. K. Hendrie and J. Leonard, Tetrahedron, 1987, 43, 3289 CrossRef CAS. For a review on the use of diamines as organocatalysts see:
(b) S. Saito and H. Yamamoto, Acc. Chem. Res., 2004, 37, 570 CrossRef CAS PubMed.
- ESI†.
- W. S. Mattews, J. E. Bares, J. E. Bartmess, F. G. Bordwell, F. J. Cornforth, G. E. Drucker, Z. Margolin, R. J. McCallum, G. J. McCollum and N. R. Vanier, J. Am. Chem. Soc., 1975, 97, 7006 CrossRef.
- M. B. Cid, S. Duce, S. Morales, E. Rodrigo and J. L. G. Ruano, Org. Lett., 2010, 12, 3586 CrossRef CAS PubMed.
-
(a) S. Duce, M. Jorge, I. Alonso, J. L. García-Ruano and M. B. Cid, Eur. J. Org. Chem., 2013, 7067 CrossRef CAS PubMed;
(b) F. G. Bordwell, J.-P. Cheng, M. J. Bausch and J. E. Bares, J. Phys. Org. Chem., 1988, 1, 209 CrossRef CAS PubMed.
-
(a) Z. Niu, X. He and Y. Shang, Tetrahedron: Asymmetry, 2014, 25, 796 CrossRef CAS PubMed;
(b) J. O. Guevara-Pulido, J. M. Andrés and R. Pedrosa, J. Org. Chem., 2014, 79, 8638 CrossRef CAS PubMed;
(c) J. O. Guevara-Pulido, J. M. Andrés and R. Pedrosa, Eur. J. Org. Chem., 2014, 8072 CrossRef CAS PubMed.
- S. P. Lathrop and T. Rovis, J. Am. Chem. Soc., 2009, 131, 13628 CrossRef CAS PubMed.
-
(a) N. Mase, R. Thayumanavan, F. Tanaka and C. F. Barbas III, Org. Lett., 2004, 6, 2527 CrossRef CAS PubMed;
(b) H. Chen, Y. Wang, S. Wei and J. Sun, Tetrahedron: Asymmetry, 2007, 18, 1308 CrossRef CAS PubMed.
Footnote |
† Electronic supplementary information (ESI) available: Copies of 1H-NMR and 13C-NMR spectra for all new compounds and copies of the HPLC chromatograms. CCDC 1029706. For ESI and crystallographic data in CIF or other electronic format see DOI: 10.1039/c5ra11215j |
|
This journal is © The Royal Society of Chemistry 2015 |
Click here to see how this site uses Cookies. View our privacy policy here.