DOI:
10.1039/C5RA11101C
(Review Article)
RSC Adv., 2015,
5, 65757-65767
A review on natural surfactants
Received
11th June 2015
, Accepted 24th July 2015
First published on 24th July 2015
Abstract
Natural surfactants or biosurfactants are amphiphilic biological compounds, usually extracellular, produced by a variety of microorganisms from various substances including waste materials. There is increasing interest on this topic because of their unique properties such as low toxicity, functionality under extreme conditions, based on renewable substances and biologically degradable nature. The diversity of these molecules supports their potential application in the field of petroleum, medicine, agriculture, food, cosmetics etc. They are also effective in curtailing the green-house effect by reducing the emission of CO2. They can be termed as ‘green’ because of their low toxicity, biodegradability and relative stability under a wide range of physicochemical environments. In spite of possessing diverse structures and better physicochemical properties than chemical surfactants, biosurfactants are not able to compete with their synthetic counterparts because of their high production & downstream costs. The commercial realization of these eco-friendly biomolecules is restricted by low productivity, expensive downstream processing and lack of appropriate understanding of the bioreactor systems for their production. But we expect that in future better reactor design and product recovery technology would be developed and overproducer microbial strain would be screened. Then production cost would be decreased and yield would be increased i.e. the production would be both ecologically & economically favored. The present review deals with an overall view on biosurfactants, their properties, advantages & disadvantages, production, characterization, application along with a recommendation for future research.
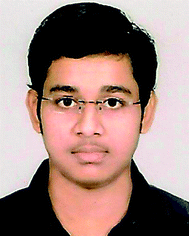 Sourav De | Sourav De was born in Arambagh, Hooghly in 1993. He completed his B.Sc. degree in Chemistry (Hons.) from St. Xavier's College, Kolkata in 2013. He is currently pursuing a M.Sc. degree in The University of Burdwan. He is working as a project trainee under my guidance. |
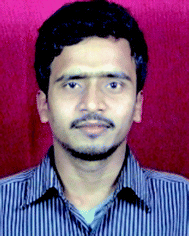 Susanta Malik | Susanta Malik was born in Kalna, Burdwan in 1988. He completed a M.Sc. degree from The University of Burdwan in 2011 and received a UGC-RGNF fellowship in 2012. He is working in my lab in the “Homogeneous Catalysis” division. |
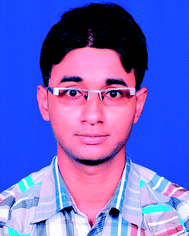 Aniruddha Ghosh | Aniruddha Ghosh was born in Raniganj, in 1988. He completed a M.Sc. degree from The University of Burdwan in 2010 and received a NET-UGC fellowship in 2010. He is working as a SRF in the lab. He is working in my lab in the “Homogeneous Catalysis” division. |
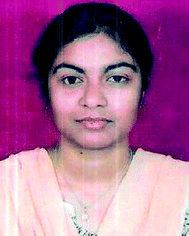 Rumpa Saha | Rumpa Saha was born in Burdwan, 1987. She completed her M.Sc. degree from the University of Burdwan, 2009 and received a CSIR NET fellowship in 2009. She was awarded her Ph.D. degree from the University of Burdwan in 2015. Dr Rumpa Saha is presently working as an Assistant Professor in the Department of Chemistry, TDB College, Raniganj, Burdwan, India. |
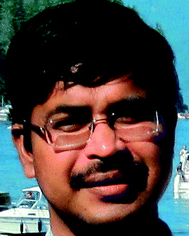 Bidyut Saha | Bidyut Saha was born in Birbhum, WB, India in 1975. He obtained his Ph.D. degree from Visva Bharati University, India in 2007. He was a visiting scientist between 2009-2010 in the Department of Chemistry, UBC, Vancouver, Canada. Dr Saha is presently working as an Associate Professor in the Department of Chemistry, The University of Burdwan, India. His area of interests is bioremediation of toxic metals, micellar catalysis and inorganic reaction mechanisms. He has published 80 papers in international journals. |
1. Introduction
Amphipathic molecules having the ability to form micelles are termed as SURFace ACTive AgeNTS or SURFACTANTS. The surface activating ability makes surfactants excellent emulsifiers, dispersing and foaming agents.1 They reduce surface tension of aqueous media (e.g. air–water), and the interfacial tension of liq–liq (e.g. oil–water or water–oil) or liq–solid (e.g. wetting phenomena) systems. They assist the solubility of polar compounds in organic solvents. Surfactants are the active ingredients found in soaps and detergents and are commonly used to separate oily stuff from a particular media. Because of these properties, surfactants find application in a variety of industrial processes. Surfactants are of synthetic or biological origin. Some of the important synthetic surfactants are linear alkyl benzenesulphonates, α-olefin sulphonates, alcohol ether sulphates, alcohol sulphates etc.2 The effectiveness of a surfactant is determined by its efficiency to reduce the surface tension. A good surfactant can lower the surface tension of water from 72 to 30 mN m−1 and interfacial tension for water against n-hexadecane from 40 to 1 mN m−1.3 More efficient surfactants have a lower critical micelle concentration (CMC). Surfactants are extensively used for industrial, food, agricultural, cosmetics and pharmaceutical application. However most of these compounds are of synthetic origin and potentially bring out environmental and toxicology problems due to their recalcitrant and persistent nature.4 Currently, the attention is on alternative environmental friendly processes for the production of different types of natural-surfactants or biosurfactants.
Biosurfactants are surfactants synthesized by living cells.5 They possess the characteristic property of lowering the surface and interfacial tension using the same mechanisms as synthetic surfactants.6 Emulsification, dispersion, solubilization, foaming, wetting, detergent capability and antimicrobial activity in some cases are the major functions of biosurfactants.7 Majority of the biosurfactants are exolipids, although they are cell-bound in some examples.5 Biosurfactants are biologically produced by yeast or bacteria or fungi from various substrates like oils, sugars, alkanes and wastes etc. For instance, Pseudomonas aeruginosa can produce rhamnolipids from substrates including C11 and C12 alkanes, succinate, pyruvate, citrate, fructose, glycerol, olive oil, glucose and manitol.8,9 In general, the CMCs of the biosurfactants differ from 1 to 200 mg L−1 and their molecular mass from 500 to 1500 Daltons.9 The first microbial surfactants on the market were sophorolipids. Biosurfactants are considered environmentally “friendly” because of their relatively nontoxic and biodegradable nature. Interest on biosurfactants have been progressively escalating due to their eco-friendly nature, diversity, possibility of large-scale production, selectivity, performance under critical circumstances and their forthcoming applications in environmental fortification. In recent years biosurfactants have been increasingly attracting the eye of the scientific and industrial community.
2. Biosurfactant properties
Surfactants are amphiphiles with both hydrophilic and hydrophobic moieties. This feature renders surfactants capable of reducing surface and interfacial tension and forming emulsions. Interest in research and application on biosurfactant is progressively increasing due to their environmental friendly nature and lower toxicity comparing with synthetic surfactant.10 Diverse functional properties such as emulsification, foaming, wetting, cleansing, surface activity, phase separation and reduction in viscosity of crude oil, makes it some of the most versatile process chemicals.1 The activities of biosurfactant depend on their concentration until the critical micelle concentration (CMC) is obtained. Above the CMC, biosurfactant monomer molecules associate to form micelles, bilayers and vesicles (Fig. 1). This property enables biosurfactants to minimize the surface and interfacial tension and enhance the solubility and bioavailability of nonpolar organic compounds.11 The CMC is usually used to measure surfactant efficiency. More efficient biosurfactants have lower CMC, i.e. less biosurfactant is needed to decrease the surface tension.1 Hydrophilic-lipophilic balance (HLB) value is a measure to indicate the type of emulsion (e.g. oil in water or water in oil). Emulsifiers with low HLB stabilize water-in-oil emulsion, whereas emulsifiers with high HLB do the opposite.12 According to some investigations, the surface activity of biosurfactants is analogous to surface activity of synthetic surfactants. Biosurfactants are able to minimize surface tension of water to 29.0 mN m−1 (at the CMC), whereas Pluronic F-68 to 42.8, SDS to 28.6.13
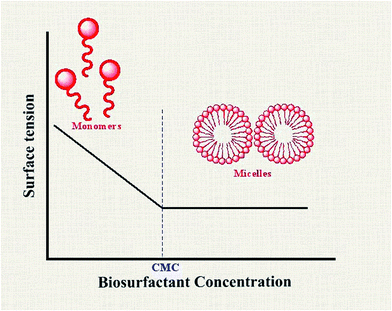 |
| Fig. 1 Relationship between surface tension and biosurfactant concentration & formation of micelles. | |
The main distinctive features of biosurfactants are:
2.1. Surface activity
Surface tension of the water is 72.75 mN m−1 (at 20 °C) and when surfactant is added, this surface tension value is lowered. A good biosurfactant can reduce surface tension of water from 72 to 35 mN m−1 and the interfacial tension of water against n-hexadecane from 40 to 1 mN m−1.3 Surfactin produced from B. subtilis can lower the surface tension of water to 25 mN m−1 and interfacial tension of water against n-hexadecane to <1 mN m−1.14 In general, biosurfactants are more efficient and effective and their CMC value is about 10–40 times lesser than that of chemically synthetic surfactants.1
2.2. Emulsification and de-emulsification
Emulsification is dispersion of one liquid into another as microscopic droplets leading to the mixing of two immiscible liquids. De-emulsification process breaks emulsions through the disruption of stable surface between the bulk phase and the internal phase. This is important in oil production processes. Stable emulsions having lifespan of months and years can be made from biosurfactants.15 Biosurfactants has the ability to stabilize (emulsify) or destabilize (de-emulsify) the emulsion. Generally high-molecular-mass biosurfactants are better emulsifiers. Sophorolipids produced from T. bombicola have been shown to reduce surface and interfacial tension, but are not good emulsifiers.16 While liposan does not reduce surface tension, but has ability to emulsify edible oils.17 This property of biosurfactants is especially useful for cosmetics and food industry.
2.3. Biodegradability
Biosurfactants, due to having biological origin, seem to be more easily biodegradable than their synthetic counterparts. The biodegradability tests of sophorolipids produced by non-pathogenic yeast Candida bombicola, carried out according to the OECD Guidelines for Testing of Chemicals (301C Modified MITI Test), showed that biodegradation of biosurfactants starts immediately after cultivation whereas synthetic surfactants showed no biodegradability after 8 days.18 Another research indicated that rhamnolipid biosurfactants are biodegradable under aerobic and anaerobic conditions, while synthetic surfactant Triton X-100 is partially biodegradable under aerobic conditions and nonbiodegradable under anaerobic conditions.19
2.4. Temperature, pH and ionic strength tolerance
Biosurfactants are stable at various temperature and pH. Lichenysin from B. licheniformis JF-2 is stable up to 50 °C, pH of 4.5–9.0 and at NaCl and Ca concentrations of 50 g L−1 and 25 g L−1 respectively.20
2.5. Chemical diversity
The chemical diversity of biosurfactants offers a wide selection of surface-active agents with properties that notably commensurate specific application.
3. Advantages and disadvantages
3.1. Advantages
Chemically synthesized surfactants are mainly petroleum based. So they are usually non-biodegradable thereby remain toxic to the environment. Again, synthetic surfactants may bio-accumulate and their production processes and by-products can be hazardous to environment. Because of increasing awareness on the need to protect this ecosystem, applications of surfactants from natural origin are possible alternatives to chemically synthesized ones.21–25
In contrast to common chemically synthesized surfactants that usually possess alkyl chains of ten or more carbon units, many biosurfactants possess surprisingly short alkyl chains. Such structures promote the aqueous solubility of these biosurfactants. In spite of their aqueous solubility, they can have remarkably low CMCs compared to synthetic surfactants of similar structure. For example, nonionic (i.e., low pH), multicomponent monorhamnolipid mixtures, where the heptyl chain congener is most prevalent, have CMC values that range from <1 mM to ∼10 mM depending on the ionic strength of solution.26 As the pH increases, CMC value increases and the rhamnolipids become deprotonated. Scientific researchers have shown that biosurfactants offer many advantages over their chemical equivalents.
3.1.1. Biodegradability. Owing to low toxicity, biological origin and simple chemical structure, these surface active compounds do not persist in the environment and are degraded easily by microorganism.
3.1.2. Biocompatibility and digestibility. Biological origin offers them inherent feature of compatibility, which grants their unabated usage in cosmetics, pharmaceuticals, agricultural fields and as functional food additives.
3.1.3. Generally low toxicity. Biosurfactant exhibits lower toxicity than the chemically-derived surfactants. For instance, in naphthalene solubilization tests, glycolipids from Rhodococcus species 413A were 50% less toxic than Tween 80.27
3.1.4. Surface and interface activity. According to Mulligan, a good surfactant can lower surface tension of water from 72 to 35 mN m−1 and the interfacial tension water/hexadecane from 40 to 1 mN m−1.3 Surfactins are able to lower the surface tension of water to 25 mN m−1 and the interfacial tension of water/hexadecane to <1 mN M−1.28
3.1.5. Availability of raw materials. Biosurfactants can be produced from relatively cheap raw materials available in abundance. The carbon sources like hydrocarbons, carbohydrates to lipids can be used individually or blended with each other.29
3.1.6. Acceptable production economics. Biosurfactants can also be produced from industrial wastes and by-products and this is an advantage for bulk production.
3.1.7. Physical factors. Many biosurfactants are active at extreme temperatures, pH and salinities.
3.1.8. Specificity. Biosurfactants being complex organic molecules having specific functional groups are often specific in their action. This property renders prime importance in detoxification of specific pollutants, development of specific cosmetic, de-emulsification of industrial emulsions, different pharmaceutical and food applications.
3.1.9. Environmental control. Biosurfactants are effective in controlling oil spills, industrial emulsions handling, detoxification & biodegradation of industrial effluents and bioremediation of contaminated soil.The worldwide production of surfactants increased to 17 million metric tons in 2000 (including soaps) with expected future growth rates of 3–4% per year globally.30
3.2. Disadvantages
Despite of having numerous advantages, biosurfactants have the following disadvantages too.31
3.2.1. Toxicity. Although the biosurfactants are thought to be environmentally friendly, some experiments showed that under certain circumstances they can impart toxicity to the environment.32 For instance, hemolytic activity test on human erythrocyte at 37 °C of lipopeptide biosurfactants (B. subtilis ATCC 6633) shows ability to rupture erythrocytes.33 Though in comparison with synthetic surfactants (CTAB, TTAB, BC and SDS), the effect is lower. Again biosurfactants do not pose harmful effects to kidney, heart, lung, liver and interfere in blood coagulation in normal clotting time. So careful and controlled use of these interesting surfactants will surely help in the enhanced cleanup of the toxic environmental pollutants and provide us a clean environment to live.
3.2.2. Expensive large scale production. Production of biosurfactants in large scale may be expensive. However this problem could be overcome by utilization of waste substrates and combating with their polluting effects at the same time which will balance the overall costs.
3.2.3. Difficulty in obtaining pure substances. There is difficulty in obtaining pure substances, which has particular importance in food, cosmetic and pharmaceutical applications. Multiple consecutive steps required in downstream processing of diluted broths may be the cause behind it.
3.2.4. Very low productivity. Over producing strains of microbes are very rare and those found generally display very low productivity.
3.2.5. Strong foam formation. Due to strong foam formation, an improvement in the production yield is hampered. Therefore diluted media have to be applied and only immobilized systems can provide an increased productivity.
4. Biosurfactant is the “Green” surfactant
Biosurfactants display a lot of advantages over chemically synthesized surfactants. They are less toxic, highly effective, and easily biodegradable. So biosurfactants are considered to be “green” alternatives to surfactants of synthetic origin.34
5. Biosurfactant classification
Chemically synthesized surfactants are usually classified according to the nature of their polar groups (cationic, anionic, zwitterionic and non-ionic). Biosurfactants are generally categorized mainly by their chemical structure and microbial origin. Structurally, they are amphiphilic containing a hydrophilic moiety (comprising an acid, alcohol, peptide cations, or anions, mono-, di- or polysaccharides) and a hydrophobic moiety (made up of unsaturated or saturated hydrocarbon chains or fatty acids). The hydrophilic part of biosurfactants is responsible for their rate of solubility in water. The lipophilic part is responsible for capillary activity. These two parts are joined by ester linkage (including lactones) with organic and inorganic acids or amide linkage (single and peptide) or glycosidic linkage (sugar–sugar and sugar-hydroxy fatty acids). Rosenberg and Ron classified biosurfactants in two categories according to molecular mass.35
(i) Low-molecular weight surface active agents: having efficiently lower surface and interfacial tension. Major classes include glycolipids, lipopeptides and phospholipids.
(ii) High-molecular weight surface active agents: effective as emulsion-stabilizing agents. Major classes include polymeric and particulate surfactants.
The major types of biosurfactants and their producer are presented in Table 1 (adapted from ref. 1).
Table 1 Classification of biosurfactant and important types of microbial origin
Biosurfactant |
Microorganism |
Group |
Sub-group |
Class |
Low-molecular weight surface active agents |
Glycolipids |
Rhamnolipids |
P. aeruginosa, Pseudomonas sp. |
Sophorolipids |
T. bombicola, T. apicola |
Trehalolipids |
R. erythropolis, Mycobacterium sp. |
Cellobiolipids |
U. zeae, U. maydis |
Lipopeptides & lipoproteins |
Surfactin |
B. subtilis |
Viscosin |
P. fluorescens |
Peptide-lipid |
B. licheniformis |
Fatty acids, neutral lipids and phospholipids |
Fatty acids |
C. lepus |
Neutral lipids |
N. erythropolis |
Phospholipids |
T. thiooxidans |
High-molecular weight surface active agents |
Polymeric surfactants |
Emulsan |
A. calcoaceticus |
Biodispersan |
A. calcoaceticus |
Alasan |
A. radioresistens |
Liposan |
C. lipolytica |
Particulate surfactants |
Vesicles and fimbriae |
A. calcoaceticus |
Whole cells |
Variety of bacteria |
6. Biosurfactant production
Many microorganisms, such as bacteria, yeasts, and fungi are able to produce surface-active agents during their growth. For instance,
• Bacteria producing biosurfactants: rhamnolipids from Pseudomonas aeruginosa and surfactin from Bacillus Subtilis.5
• Yeasts producing biosurfactants: sophorolipids from Torulopsis bombicola and peptidolipid from Candida petrophilum.5
• Fungi producing biosurfactants: sophorolipids from Candida batistae and Candida bombocola.36
In addition to production by growing cells, resting cell systems are also able to produce biosurfactants. Fungi yield better amount of biosurfactant when compared to bacteria. Presence of rigid cell wall in them may be the reason behind.36
Biosurfactants are biologically produced from various substrates. Its downstream processing costs account up to 60% of the total production cost.1 This makes commercial production of biosurfactants quite expensive. So now a days, production from inexpensive and waste substrates is underlined to overcome expensive production cost. A lot of hazardous and non-hazardous wastes are generated every year throughout the world. Their proper management and utilization is needed badly. So, residues from tropical agronomic crops, residues of the fruit processing industries, waste from oil processing mills, residues from the coffee processing industry etc. have been reported as substrates for biosurfactant production.37–41 These are explained in details below.
6.1. Oil wastes
Oil wastes from vegetable oil refineries and the food industry are being used as appropriate substrates for biosurfactant production. Sunflower seed oil and oleic acid can be used for the production of rhamnolipids by Thermus thermophilus HB8.42 Incorporation of these cheaper oils and oil wastes as source in the industrial production media might reduce the overall costs of biosurfactant production.
6.2. Dairy whey
From 1 kg of paneer (cheese) about 6 liters of whey is produced. This whey from dairy industries is also a cheap and durable substrate for biosurfactant fermentation. Using dairy wastes as substrates, the production of high concentrations of sophorolipids were achieved using two-stage cultivation process for the yeast Cryptococcus curvatus ATCC 20509.43
6.3. Starchy waste
Starchy waste materials are also inexpensive raw materials and suitable for the production of biosurfactants. Potato processing industry is a major source of starchy waste. These wastes are rich source of carbon (in the form of sugars and starch), nitrogen and sulfur (from protein), inorganic minerals, trace elements and vitamins. Use of potato effluents as substrates for surfactin production was reported by Thompson.44
6.4. Molasses
Molasses are the co-product of sugar industry generated during sugar manufacturing from either sugar beet or sugarcane and are rich source of available carbon. Patel and Desai treated molasses and corn-steep liquor as the primary source of carbon and nitrogen for the production of rhamnolipid biosurfactant using P. aeruginosa GS3.45
6.5. Animal fat
A large quantity of animal fat and tallow can be obtained from meat processing industries and can be used for biosurfactant production. Deshpande and Daniels treated animal fat for the production of sophorolipids using the yeast, C. bombicola.46
6.6. Soap stock
Soap stock is a by-product of oil-seed processing industry. It can also be used for the production of biosurfactant. P. aeruginosa LB1, isolated from soil contaminated by petroleum, could produce surface-active rhamnolipids (RLLBI) by batch fermentation in a mineral salts medium with soap stock as the only carbon source.47
6.7. Production by biotransformation
In the last few years, considerable attention has been paid toward the production of biosurfactants by biotransformation. The main objective is to use microbial fermentation to get different hydrophobic and hydrophilic moieties of biosurfactants. They could then be joined by enzymatic treatment to generate commercial biosurfactants. These enzyme systems are highly specific and so the reactions can easily be carried out at room temperature and pressure. As for example, soybean lecithin can be converted to a new biosurfactant by phospholipase D obtained from Streptococcus chromofuscus.1
7. Factors affecting biosurfactant production
The production of biosurfactant not only depends on the producer strain but also on the culture conditions. So a lot of parameters affect not only the amount of biosurfactant but also the type of product produced.48
7.1. Environmental factors
To have a large quantity of biosurfactants, it is always essential to optimize the bioprocess as the product may be influenced by changes in pH, temperature, aeration and agitation speed.
7.1.1. pH. The effect of pH on the production of biosurfactant was studied by Zinjarde and Pant.49 They reported that product was best when the pH was 8.0 which is the natural pH of sea water.
7.1.2. Temperature. Various microbial processes for the production of biosurfactant are dependent on temperature and get affected by a little change. Most of the biosurfactant productions reported till now have been performed in the temperature range of 25 to 30 °C.1
7.1.3. Aeration and agitation. Both aeration and agitation facilitate the transfer of oxygen from the gas phase to the aqueous phase. So these are significant factors that influence the production of biosurfactants. It was observed by Adamczak and Bednarski that the best production value of the surfactant (45.5 g L−1) was obtained when the air flow rate was 1 vvm and the dissolved oxygen concentration was maintained at 50% of saturation.50
7.2. Nutritional factors
The type, quality and quantity of biosurfactant produced are affected and influenced by several nutritional factors.
7.2.1. Carbon sources. Carbon sources exhibit a significant role in the growth as well as production of biosurfactants by the various microorganisms. Glycerol, glucose, mannitol, sodium acetate and ethanol are some of the water-soluble carbon sources whereas n-alkanes, olive oil are water-immiscible substrates.1
7.2.2. Nitrogen sources. Nitrogen is necessary for microbial growth as protein & enzyme syntheses depend on it. So nitrogen is important in the biosurfactant production medium. Several nitrogen compounds such as yeast extract, ammonium nitrate, ammonium sulphate, urea peptone, sodium nitrate, meat and malt extracts have been used for the production of biosurfactants. It has been found that yeast extract is the mostly used nitrogen source for biosurfactant production.
7.2.3. C/N and C/P ratio. Increased productivity of glycolipid (rhamnolipid) is seen at high carbon and nitrogen (C/N) ratio and carbon and inorganic phosphorous (C/P) ratio.51–53
7.2.4. Metal ion concentration. Metal ions can form important cofactors of many enzymes. So, metal ion concentrations play a very important role in the production of some biosurfactants. The overproduction of surfactin biosurfactant occurs in presence of Fe2+ in mineral salt medium.54
7.2.5. Incubation time. Incubation time also have a significant effect on the biosurfactant production. Different microbes are able to produce biosurfactants at different intervals of time. Using animal fat, the maximum biosurfactant production by Candida bombicola was observed after the 68 h of incubation.36
7.3. Cultivation strategies
Cultivation strategies for biosurfactants include shake flask, batch, fed-batch, continuous and integrated microbial/enzymatic processes.55 Most of the studies on this topic involve the optimization of culture conditions in shake flask. Often bioreactor is applied in continuous or fed-batch fermentation in the production of biosurfactant. One of the main disadvantages for the production in bioreactor is intense foam formation caused by the surfactant when the solution is aerated and agitated. Specifically with P. aeruginosa, high foam formation is further enhanced by the presence of extracellular proteins, which results in increased production cost.56
7.4. Downstream processing
Reduction of downstream costs is an important factor towards the establishment of an economic process. It is proposed for some industrial applications that downstream costs are liable for approximately 60% of the total production costs.1 Conventional methods for recovery of biosurfactants are acid precipitation, solvent extraction, crystallization, ammonium sulfate precipitation and centrifugation.1 Recent methods for biosurfactants recovery are foam fractionation, ultrafiltration and adsorption–desorption on polystyrene resins and ion exchange chromatography.
7.5. Strain improvement strategies
Metabolic and cellular engineering approaches have been successfully applied to improve strain performance in several cases. So the integration of engineering and biology is profoundly needed in order to obtain high yields and low costs on biosurfactant production.
8. Biosurfactant fermentation and recovery
Operating conditions of the fermentor can affect biosurfactant production. So control of cultural conditions i.e. pH, temperature, agitation, aeration, dilution rate, the concentration of metal ions, the nature of the carbon and nitrogen sources are necessary. As for example, the production of rhamnolipid by P. aeruginosa is optimal at pH 6.7 and 37 °C. At higher pH (>7.5), production gets ceased. So in order to obtain biosurfactants at large quantities, it is essential to optimize the fermentation conditions.
Recovery of biosurfactant depends mainly on its ionic charge (chromatography), solubility (water/organic solvents) and location (intracellular, extracellular, cell bound).1 The most common biosurfactant recovery techniques are listed in Table 2 (adapted from ref. 1).
Table 2 Different recovery methods and biosurfactants recovered by each method
Recovery method |
Biosurfactants recovered |
Solvent extraction |
Sophorolipids, liposan, trehalolipids |
Centrifugation |
Glycolipids |
Adsorption |
Lipopeptides, rhamnolipids, glycolipids |
Crystallization |
Glycolipids, cellobiolipids |
Tangential flow filtration |
Mixed biosurfactants |
Acid precipitation |
Surfactin |
Precipitation by acetone |
Glycolipids, bioemulsifier |
Ultrafiltration |
Surfactin, glycolipids |
Foam separation and precipitation |
Surfactin |
Diafiltration and precipitation |
Glycolipids |
9. Biosynthesis and regulation
Biosurfactants are amphipathic i.e. hydrophobic and hydrophilic moieties are present within the molecule. This means we have to look on at least two different synthetic paths: one leading to the synthesis of hydrophilic and one to the hydrophobic component. The hydrophobic moieties are synthesized by simple pathways of lipid metabolism. The hydrophilic counterparts exhibit a greater degree of structural complexity. So a wide variety of biosynthetic methods are involved in their synthesis.5 According to Syldatk and Wagner, four principle possibilities of biosynthesis of such amphiphilic molecules1,5 are
• Both the hydrophilic and hydrophobic moieties are synthesized de novo by two separate pathways.
• The hydrophilic moiety is synthesized de novo whereas the synthesis of the hydrophobic moiety is induced by substrate.
• The hydrophobic moiety is synthesized de novo whereas the synthesis of the hydrophilic moiety is substrate dependent.
• The synthesis of both the hydrophobic and hydrophilic moieties depends on the substrate used.
10. Purification and structure elucidation
The most significant step next to the production of biosurfactants is their purification from the fermentation media so as to make them available to various industrial uses. Several methods such as ion exchange, adsorption–desorption, solvent extractions, acid precipitation, filtration, centrifugation are known for their purification. Various solvents such as hexane, chloroform, ethyl acetate, pentane, methanol etc. are used for the purification of biosurfactants.36
For the complete characterization of biosurfactants, several chromatographic and spectroscopic techniques are used either individually or in combination. They are TLC, HPLC, FTIR, GC-MS, NMR, LC-MS and FAB-MS.36 Some of these analytical methods used for different biosurfactants are shown in Table 3.
Table 3 Biosurfactants and analytical methods used for their characterization
Biosurfactant |
Analytical method |
Reference |
Rhamnolipids |
FTIR, HPLC, TLC |
57,58 |
Sophorolipids |
FTIR, HPLC |
59 |
Trehalolipids |
HPLC |
60 |
Surfactin |
HPLC |
61 |
Phospholipids |
GC/MS |
62 |
11. Estimation of biosurfactant activity
The activities of a biosurfactant can be determined by measuring its ability to change surface & interfacial tensions and hydrophilic-lipophilic balance (HLB).1 When a surfactant is added to a liquid system in a significant amount, a reduction of surface tension is observed up to a critical value. Above this surfactant monomers associate readily to form micelles, bilayers and vesicle (Fig. 1). This value is known as the critical micelle concentration (CMC).63–65 Reduction of surface & interfacial tension and critical micelle concentration (CMC) values can be measured by tensiometric methods like the Du-Noüy-Ring or Wilhelmi plate approach.55 The HLB value offers prediction of surfactant property. When a new surfactant is got, it is compared with surfactant of known HLB value to predict its property.5 The HLB value varies between 0 and 20. According to Griffin, the HLB can be calculated as following.
MWHP denotes the molecular weight of the hydrophilic part and MWSA denotes the molecular weight of the whole surface-active agent.
The HLB value allows us to predict the surfactant properties of a molecule as shown in Table 4.55
Table 4 HLB value and representative predicted property of a biosurfactant
Hydrophilic-lipophilic balance value |
Predicted property |
0 to 3 |
Anti-foaming agent |
4 to 6 |
Water/oil emulsifier |
7 to 9 |
Wetting agent |
8 to 18 |
Oil/water emulsifier |
13 to 15 |
Typical detergent |
10 to 18 |
Solubilizer |
12. Cost analysis
Design and economic evaluation of a process is important before constructing and operating a plant and this requires combined knowledge from both scientific and engineering disciplines.66 The overall cost analysis includes the estimation of capital and operating expenditure.
Based on process economic model developed by Superpro Designer process simulation software, the annual operating cost for the production of 90.7 million kg sophorolipid from glucose and high oleic sunflower oil was US$268 million with a unit production cost of US$2.95 kg−1. However, this for the same amount of sophorolipid from glucose and oleic acid was US$230 million, which resulted in a lesser unit production cost of US$2.54 kg−1.67 Even though sophorolipid was more expensive than its chemical counterparts, its wider application potential will make them more attractive in the surfactant market.
13. Biosurfactant application
Biosurfactants owing to their unique functional properties have numerous applications in several fields.
13.1. Microbial enhanced oil recovery (MEOR)
MEOR is a powerful technique to recover oil remaining in reservoirs with low permeability or crude oil with high viscosity. The remaining oil in the reservoir is often located in locations that are hard to access and the oil remains trapped in the pores by capillary pressure.68 Biosurfactants minimize the interfacial tension between oil/water and oil/rock. This diminishes the capillary forces restricting oil from moving. Biosurfactants can also bind tightly to the oil–water interface thereby forming emulsion. This stabilizes the desorbed oil in water and removes oil along with injected water.69 Bacillus subtilis at 45 °C has good sand-pack oil recovering ability.70,71
13.2. Soil washing technology and bioremediation of crude oil-contaminated environments
Highly hydrophobic contaminants have ability to bind very tightly with soil, thereby inaccessible to biodegradation. Surfactants potentially have the ability to promote desorption of the contaminants from soil. Biosurfactant like rhamnolipids were effective in removing polycyclic aromatic hydrocarbons (PAHs) and pentachlorophenol from soil with removal efficiency of 60–80%.72,73 Crude oils have very low water solubility, high adsorption onto soil matrix. Oil-contaminated soil is especially difficult for bioremediation as oil excess forms droplets or films on soil particles. It is a powerful obstacle against microbial degradation. Biosurfactants are produced to reduce the tension at the hydrocarbon–water interface thereby pseudosolubilizing the hydrocarbons and thus increasing mobility, bioavailability and consequent biodegradation.74 That's why biosurfactants are very useful for applications in the oil industry and this is reflected in the market, where the large majority of biosurfactants produced are for petroleum-related applications.75 The BS29 bioemulsions produced from Gordonia sp. are promising washing agents for the bioremediation of hydrocarbon-contaminated soils.76
13.3. Metals remediation
Contamination of soil with heavy metals is very hazardous for human and other living organisms in this ecosystem. Due to their exceptionally toxic nature, presence of even low concentrations of heavy metals in the soils has been found to have serious danger. Several biosurfactant has the ability of metal remediation. Rhamnolipids are known to have ability to remove heavy metals.77
13.4. Biomedical field
The biological surfactants are extensively useful in the biomedical grounds. Several biosurfactants have strong antibacterial, antifungal and antivirus activity. These surfactants act as anti-adhesive agents to pathogens and so they are useful for treating many diseases. So they can be used as therapeutic and probiotic agent.70 Rhamnolipid produced by Pseudomonas aeruginosa, mannosylerythritol lipids from Candida Antarctica, lipopeptides produced by B. subtilis and B. licheniformis have been shown to have antimicrobial activities.78 Iturin, a lipopeptide produced by B. subtilis showed anti-fungal activity against the morphology and membrane structure of yeast cells.54 A rhamnolipid mixture obtained from P. aeruginosa AT10 is found to have inhibitory activity against the bacteria Escherichia coli, Serratia arcescens, Micrococcus luteus, Alcaligenes faecalis, Mycobacterium phlei and Staphylococcus epidermidis and excellent antifungal properties against Aspergillus niger, Enicillium crysogenum, Chaetonium globosum, Aureobasidium pullulans and the phytopathogenic Rhizoctonia solani and Botrytis cinerea.79 Sophorolipids from C. bombicola has a virucidal activity against the human semen.28 Other advantages and applications of bio-surfactant in medicine are gene delivery, immunomodulation, wound healing, insecticidal, antitumoral activities etc.
13.5. Agricultural field
Use of chemicals and pesticides in agricultural field for arthropod control often produce undesirable effects. But biosurfactant also shows antimicrobial and insecticidal activity. Moreover, no adverse effects on the environments or human beings are anticipated from them. Lipopeptide biosurfactants produced by several bacteria show insecticidal activity against fruit fly Drosophila melanogaster and hence they can be used as biopesticide.3 In agricultural field, biosurfactants can also be used to obtain good wettability and to achieve even distribution of fertilizer in the soil.
13.6. Laundry industry
Almost all surfactants that are being used as commercial laundry detergents are chemically synthesized and exert toxic effects to fresh water living organisms. But biosurfactants are eco-friendly. They also show good emulsion formation capability with vegetable oils. Moreover, biosurfactants like CLP (Cyclic Lipopeptide) are stable over a wide pH range (7.0 to 12.0) and no loss of their surface-active property occurs when they are heated at high temperature.80 Their excellent compatibility and stability favors their inclusion in the formulation of laundry detergents.
13.7. Food processing industry
Biosurfactants are also being used in food industries generally as food additives (emulsifiers). For instance, fatty acid esters containing glycerol, lectin and its derivatives, sorbitan or ethylene glycol and ethoxylated derivatives of monoglycerides along with recently synthesized oligopeptide.81 These emulsifiers improve the taste, flavor and quality of food-products with minimal health hazards. Their potential applications in food industry includes fat stabilization in food oils, defoaming in sugar production, increased solubility in instant drinks and soups, starch complexation in instant potatoes and protective coatings for vegetables, fruits etc.
13.8. Cosmetic industry
Biosurfactant have found a niche in the health care and cosmetic industry due to their skin friendly properties. Sophorolipids can be esterified or blended with ethylene oxide and can be used as skin moisturizers. Kao Corporation (www.kao.com/jp/) in Tokyo, Japan uses them in the personal care sector. Biosurfactants are used as foaming agents, emulsifiers, solubilizers, wetting agents, cleansers, antimicrobial agents, mediators of enzyme action, in insect repellents, acne pads, antacids, bath products, anti-dandruff products, contact lens solutions, baby products, mascara, lipsticks, toothpaste etc.82
13.9. Reduction of CO2 emissions
Atmospheric gases such as CO2, water vapors and methane are able to absorb long wave radiation (infra-red) emitted from the earth's surface. This aids in heating the earth's surface (greenhouse effect). Studies have shown that biosurfactants a have a role to play in the reduction of CO2 emission. About 1.5 million tons of CO2 emissions were avoided in 1998 by the production of oleochemical surfactants.83
13.10. Other
Others potential applications include ceramics, pulp & paper and metal processing, fixing dyes etc.
14. Limitation for the commercial use
Because of complexity and high production cost, commercial use of biosurfactants on a large scale is limited. Till now, rhamnolipids and surfactin are commercially available. Rhamnolipids are produced by a very few number of companies such as AGAE Technologies (http://www.agaetech.com), Jeneil Biosurfactant Company (JBR products) and Rhamnolipid, Inc. (http://www.rhamnolipidholdings.com). However, even in these companies, readily available products do not always have for sale. One of the reasons of the above fact may be that rhamnolipids are the only biosurfactant that has been approved by US Environmental Protection Agency for use in pharmaceuticals, cosmetics and food products.84
15. Recommendations for future research
Comparing to synthetic surfactants, biosurfactants possess many industrially attractive properties and advantages. Yet, they have not been commercialized extensively due to high investment costs. The major operating costs in their production are fermentation and recovery. To overcome this, use of pure carbon sources (such as oleic acid) can be done. But use of these pure carbon sources is extremely expensive. Use of low-cost raw materials can be a possible solution for this obstacle.39 Different substances such as vegetable oils, animal fat, distillery and dairy wastes, soya molasses, starchy wastes etc. can be used as raw materials. Rhamnolipid production from olive oil mill effluent by Pseudomonas spp. is a remarkable development in this field.1 Recent improvement in the production technology of biosurfactants has already enabled a 10 to 20-fold increment in productivity, but further significant improvements are required.
Since downstream processing and purification costs are important, advancements are required in production of biosurfactant under non-sterile environments. If it is feasible, it could significantly curtail the high production costs. If the remediation can be executed in situ, production could also be performed in situ. This would be technical & cost effective and less labor & transport would be required. So the process would be both ecologically and economically favorable.
Little is known about in situ production of biosurfactant by microbes. Most of the productions were done under laboratory conditions. So development is needed in this section.
16. Conclusion
In spite of many laboratory based success in biosurfactants production, its production at industrial scale remains a challenging issue. The commercial production of any product depends on its market demand, ease of availability of raw material and production cost. Low productivity, expensive downstream processing and dearth of appropriate understanding of the bioreactor systems are the major barrier in the production of biosurfactant. We expect that in future super-active microbial strains would be developed using genetic modification for their production at industrial level. Invention of modern methods and discovery of more reliable sources are also expected in future. So yields would then be increased and production costs would be decreased and new biosurfactants will continue to be discovered and chemistry of these molecules would be better understood. So the time is not far when the biosurfactants will begin to compete favorably with their synthetic equivalents in the surfactant industry. Rapidly growing number of publications and patents on the search term “biosurfactant” (Fig. 2) clearly demonstrate rapidly increasing body of research and interest on this topic.
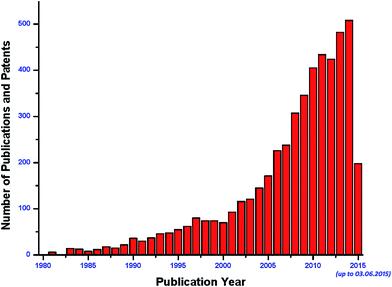 |
| Fig. 2 The number of publications and patents obtained from a year wise search for the term “biosurfactant” on SciFinder search directory (on 03.06.2015). | |
Acknowledgements
S.D. acknowledges invaluable discussions with Alpana Nayek De, Parul RKS High School, Arambagh, India and Sankar Prosad De, Baurah Panchanan High School, Burdwan, India on biosurfactant origin and structure. We thank Parichita Saha, IIT, Madras, India for providing useful data related to the number of patents and publications.
References
- J. D. Desai and I. M. Banat, Microbiol. Mol. Biol. Rev., 1997, 61, 47–64 CAS.
- P. L. Layman, Chem. Eng. News, 1985, 63, 23–48 Search PubMed.
- C. N. Mulligan, Environ. Pollut., 2005, 133, 183–198 CrossRef CAS PubMed.
- R. S. Makkar and K. J. Rockne, Environ. Toxicol. Chem., 2003, 22, 2280–2292 CrossRef CAS PubMed.
- C. Syldatk and F. Wagner, Production of biosurfactants, in Biosurfactants and biotechnology, ed. N. Kosaric, W. L. Cairns and N. C. C. Gray, Marcel Dekker, New York, 1987, vol. 25, pp. 89–120 Search PubMed.
- A. Singh, J. D. Van Hamme and O. P. Ward, Biotechnol. Adv., 2007, 25, 99–121 CrossRef CAS PubMed.
- S. Mukherjee, P. Das and R. Sen, Trends Biotechnol., 2006, 27, 509–515 CrossRef PubMed.
- M. Robert, M. E. Mercadé, M. P. Bosch, J. L. Parra, M. J. Espiny, M. A. Manresa and J. Guinea, Biotechnol. Lett., 1989, 11, 871–874 CrossRef CAS.
- C. N. Mulligan and B. F. Gibbs, Proc. Indian Natl. Sci. Acad., 2004, B70, 31–55 Search PubMed.
- I. M. Shete, G. Wadhawa, I. M. Banat and B. A. Chopade, J. Sci. Ind. Res., 2006, 65, 91–115 Search PubMed.
- L. M. Whang, P. W. G. Liu, C. C. Ma and S. S. Cheng, J. Hazard. Mater., 2008, 151, 155–163 CrossRef CAS PubMed.
- W. Griffin, J. Soc. Cosmet. Chem., 1954, 5, 249–256 Search PubMed.
- O. Pornsunthorntaweea, P. Wongpanita, S. Chavadeja, M. Abeb and R. Rujiravanita, Bioresour. Technol., 2008, 99, 1589–1595 CrossRef PubMed.
- K. Hisatsuka, T. Nakahara, N. Sano and K. Yamada, Agric. Biol. Chem., 1971, 35, 686–692 CrossRef.
- J. Velikonja and N. Kosaric, Biosurfactants in food applications, in Biosurfactants: Production, Properties and Applications, New York, 1993 Search PubMed.
- D. G. Cooper and D. A. Cavalero, J. Biotechnol., 2003, 103, 31–41 CrossRef.
- M. C. Cirigliano and G. M. Carman, Appl. Environ. Microbiol., 1985, 50, 846–850 CAS.
- Y. Hirata, M. Ryu, Y. Oda, K. Igarashi, A. Nagatsuka, T. Furuta and M. Sugiura, J. Biosci. Bioeng., 2009, 108, 142–146 CrossRef CAS PubMed.
- P. K. Mohan, G. Nakhla and E. K. Yanful, Water Res., 2006, 40, 533–540 CrossRef CAS PubMed.
- M. J. McInerney, M. Javaheri and D. P. Nagle Jr, J. Ind. Microbiol., 1990, 5, 95–101 CrossRef CAS.
- M. Benincasa, Curr. Microbiol., 2007, 54, 445–449 CrossRef CAS PubMed.
- G. H. Sayed, F. M. Ghuiba, M. I. Abdou, E. A. A. Badr, S. M. Tawfik and N. A. M. Negm, Colloids Surf., A, 2012, 393, 96–104 CrossRef CAS PubMed.
- G. H. Sayed, F. M. Ghuiba, M. I. Abdou, E. A. A. Badr, S. M. Tawfik and N. A. M. Negm, J. Surfactants Deterg., 2012, 15, 735–743 CrossRef CAS.
- S. M. Tawfik, J. Ind. Eng. Chem., 2015, 28, 171–183 CrossRef CAS PubMed.
- S. M. Tawfik, A. A. Abd-Elaal, I. Aiad, Res. Chem. Intermed. DOI:10.1007/s11164-015-2076-4.
- A. Lebron-Paler, Solution and interfacial characterization of rhamnolipid biosurfactant from Pseudomonas aeruginosa ATCC 9027, PhD Dissertation, University of Arizona, 2008 Search PubMed.
- S. H. Kanga, J. S. Bonner, C. A. Page, M. A. Mills and R. L. Autenrieth, Environ. Sci. Technol., 1997, 31, 556–561 CrossRef CAS.
- M. Krishnaswamy, G. Subbuchettiar, T. K. Ravi and S. Panchaksharam, Curr. Sci., 2008, 94, 736–747 Search PubMed.
- N. Kosaric, Food Technol. Biotechnol., 2001, 39, 295–304 CAS.
- P. K. S. M. Rahman and E. Gakpe, Biotechnology, 2008, 7, 360–370 CrossRef CAS.
- N. Kosaric, Pure Appl. Chem., 1992, 64, 1731–1737 CrossRef CAS.
- V. S. Millioli, E. C. Servulo, L. G. Sobral and D. D. Carvalho, Global NEST J., 2009, 11, 181–188 Search PubMed.
- G. Dehghan-Noudeh, M. Housaindokht and B. S. F. Bazzaz, J. Microbiol., 2005, 43, 272–276 Search PubMed.
- S. K. Ritter, Chem. Eng. News, 2004, 82, 25–30 CAS.
- E. Rosenberg and E. Z. Ron, Appl. Microbiol. Biotechnol., 1999, 52, 154–162 CrossRef CAS.
- G. Bhardwaj, S. S. Cameotra and H. K. Chopra, J. Pet. Environ. Biotechnol., 2013, 4, 1–6 Search PubMed.
- C. J. B. Lima de, E. J. Ribeiro, E. F. C Sérvulo, M. M. Resende and V. L. Cardoso, Appl. Biochem. Biotechnol., 2009, 152, 156–158 CrossRef PubMed.
- D. Onbasli and B. Aslim, J. Environ. Biol., 2009, 30, 161–163 CAS.
- R. S. Makkar and S. S. Cameotra, Appl. Microbiol. Biotechnol., 2002, 58, 428–434 CrossRef CAS PubMed.
- N. Krieger, C. N. Doumit and A. M. David, Adv. Exp. Med. Biol., 2010, 672, 203–210 CrossRef CAS.
- A. Pandey, C. R. Soccol and D. Mitchell, Process Biochem., 2000, 35, 1153–1169 CrossRef CAS.
- A. A. Pantazaki, M. I. Dimopoulou, O. M. Simou and A. A. Pritsa, Appl. Microbiol. Biotechnol., 2010, 88, 939–951 CrossRef CAS PubMed.
- H. J. Daniel, M. Reuss and C. Syldatk, Biotechnol. Lett., 1998, 20, 1153–1156 CrossRef CAS.
- D. N. Thompson, S. L. Fox and G. A. Bala, Appl. Biochem. Biotechnol., 2000, 84–86, 917–930 CrossRef CAS.
- R. M. Patel and A. J. Desai, Lett. Appl. Microbiol., 1997, 25, 91–94 CAS.
- M. Deshpande and L. Daniels, Bioresour. Technol., 1995, 54, 143–150 CrossRef CAS.
- M. Benincasa, J. Food Eng., 2002, 54, 283–288 CrossRef.
- A. Salihu, I. Abdulkadir and M. N. Almustapha, Biotechnol. Mol. Biol. Rev., 2009, 3, 111–117 Search PubMed.
- S. S. Zinjarde and A. Pant, J. Basic Microbiol., 2002, 42, 67–73 CrossRef.
- M. Adamczak and W. Bednarski, Biotechnol. Lett., 2000, 22, 313–316 CrossRef CAS.
- A. S. Santos, A. P. W. Sampaio, G. S. Vasquez, L. M. Santa Anna, N. Pereira Jr and D. M. G. Freire, Appl. Biochem. Biotechnol., 2002, 98–100, 1025–1035 CrossRef CAS.
- L. H. Guerra-santos, O. Käppeli and A. Fiechter, Appl. Microbiol. Biotechnol., 1986, 24, 443–448 CrossRef CAS.
- C. N. Mulligan and B. F. Gibbs, Appl. Environ. Microbiol., 1989, 55, 3016–3019 CAS.
- L. Thimon, F. Peypoux and G. Michel, Biotechnol. Lett., 1992, 14, 713–718 CrossRef CAS.
- M. M. Müller, J. H. Kügler, M. Henkel, M. Gerlitzki, B. Hörmann, M. Pöhnlein, C. Syldatk and R. Hausmann, J. Biotechnol., 2012, 162, 366–380 CrossRef PubMed.
- F. D. A. Kronemberger, L. M. M. Santa Anna, A. C. L. B. Fernandes, R. R. D. Menezes, C. P. Borges and D. M. G. Freire, Appl. Biochem. Biotechnol., 2008, 147, 33–45 CrossRef CAS PubMed.
- M. Wild, A. D. Caro, A. L. Hernández, R. M. Miller and G. Soberon-Chavez, FEMS Microbiol. Lett., 1997, 153, 279–285 CrossRef CAS PubMed.
- J. Wu and L. K. Ju, J. Biotechnol., 1998, 59, 193–202 CrossRef CAS.
- Y. Hu and L. K. Ju, J. Biotechnol., 2001, 87, 263–272 CrossRef CAS.
- S. Maghsoudi, M. Vossoughi, A. Kheirolomoom, E. Tanaka and S. Katoh, Biochem. Eng. J., 2001, 8, 151–156 CrossRef CAS.
- D. A. Davis, H. C. Lynch and J. Varley, Enzyme Microb. Technol., 2001, 28, 346–354 CrossRef CAS.
- D. Koma, F. Hasumi, E. Yamamoto, T. Ohta, S. Y. Chung and M. Kubo, J. Biosci. Bioeng., 2001, 91, 94–96 CrossRef CAS.
- A. Ghosh, R. Saha, K. Mukherjee, S. K. Ghosh, P. Sar, S. Malik and B. Saha, Res. Chem. Intermed., 2015, 41, 3057–3078 CrossRef CAS.
- A. Ghosh, R. Saha and B. Saha, J. Ind. Eng. Chem., 2014, 20, 345–355 CrossRef CAS PubMed.
- K. Mukherjee, R. Saha, A. Ghosh, S. K. Ghosh and B. Saha, Spectrochim. Acta, Part A, 2013, 101, 294–305 CrossRef CAS PubMed.
- D. Petrides, Bioprocess design and economics, in Bioseparations Science and Engineering, ed. R. G. Harrison, Oxford University Press, New York, 2003 Search PubMed.
- R. D. Ashby, A. J. McAloon, D. K. Y. Solaiman, W. C. Yee and M. Reed, J. Surfactants Deterg., 2013, 16, 683–691 CrossRef CAS.
- R. Sen, Prog. Energy Combust. Sci., 2008, 34, 714–724 CrossRef CAS PubMed.
- H. Suthar, K. Hingurao, A. Desai and A. Nerurkar, J. Microbiol. Methods, 2008, 75, 225–230 CrossRef CAS PubMed.
- I. M. Banat, Bioresour. Technol., 1995, 51, 1–12 CrossRef CAS.
- R. S. Makkar and S. S. Cameotra, J. Ind. Microbiol. Biotechnol., 1997, 18, 37–42 CrossRef CAS PubMed.
- M. Garcia-junco, C. Gomez-lahoz, J. L. Niqui-arroyo and J. J. Ortega-calvo, Environ. Sci. Technol., 2003, 37, 2988–2996 CrossRef CAS.
- C. N. Mulligan and F. Eftekhari, Eng. Geol., 2003, 70, 269–279 CrossRef.
- R. Rosen and E. Z. Ron, Mass Spectrom. Rev., 2002, 21, 244–265 CrossRef CAS PubMed.
- G. Bognolo, Colloids Surf., A, 1999, 152, 41–52 CrossRef CAS.
- A. Franzetti, G. Bestetti, P. Caredda, P. La Colla and E. Tamburini, FEMS Microbiol. Ecol., 2008, 63, 238–248 CrossRef CAS PubMed.
- S. Wang and C. N. Mulligan, Water, Air, Soil Pollut., 2004, 157, 315–330 CrossRef CAS.
- L. Rodrigues, I. M. Banat, J. Teixeira and R. Oliveira, J. Antimicrob. Chemother., 2006, 57, 609–618 CrossRef CAS PubMed.
- A. Abalos, A. Pinazo, M. R. Infante, M. Casals, F. Garcýa and A. Manresa, Langmuir, 2001, 17, 1367–1371 CrossRef CAS.
- A. K. Mukherjee, Lett. Appl. Microbiol., 2007, 45, 330–335 CrossRef CAS PubMed.
- G. Bloomberg, Lebensmitteltechnologie, 1991, 24, 130–131 Search PubMed.
- E. Gharaei-Fathabad, Am. J. Drug Discovery Dev., 2010, 1, 58–69 Search PubMed.
- M. Patel, J. Ind. Ecol., 2003, 7, 47–62 CrossRef CAS.
- M. Nitschke and S. Costa, Trends Food Sci. Technol., 2007, 1, 252–259 CrossRef PubMed.
|
This journal is © The Royal Society of Chemistry 2015 |
Click here to see how this site uses Cookies. View our privacy policy here.