DOI:
10.1039/C5RA10479C
(Paper)
RSC Adv., 2015,
5, 73566-73574
Photoresponsive and wetting performances of sheet-like nanostructures of tungsten trioxide thin films grown on wood surfaces
Received
3rd June 2015
, Accepted 24th August 2015
First published on 24th August 2015
Abstract
Tungsten trioxide films with sheet-like nanostructures were grown on wood surfaces through a simple low-temperature hydrothermal method using ethanol as an inducer. The morphologies, phase structures, compositions, optical properties and wetting behaviors of the WO3-treated wood surfaces were characterized. The ethanol content in the precursor solution played an important role in the morphologies and phase structures. It was observed that the WO3 film based on the wood substrate was composed of 2D nanosheets with an edge length of 580–957 nm and a thickness of about 80 nm and was highly crystallized with a phase-pureness. The UV-vis analysis shows that the sample prepared with the 20% ethanol content responded to ultraviolet radiation of wavelength 270–350 nm very intensively. When the sample was irradiated under UV light (365 nm), there appeared an obvious color difference value (ΔC). The water contact angle (WCA) measurements demonstrated that the WO3-treated wood surfaces possessed superhydrophilic behavior and then converted to the superhydrophobic property through the octadecyltrichlorosilane (OTS) treatment. The AFM analysis indicated that a change in surface roughness of the film was responsible for photochromic and hydrophobic performances. The obtained wood surfaces exhibited photoresponsive and self-cleaning functions, which have potential applications such as information storage, smart sensors, and anti-fake materials.
1. Introduction
With the advantages of being lightweight, aesthetic and with renewable properties, wood is widely used in buildings, decorations and elsewhere in our daily lives.1–4 However, the application of wood is limited by its drawback of low dimensional stability that was caused by the numerous hydrophilic groups in cell wall polymers.5–7 Many research studies have explored the surface modification of wood. Interest has recently arisen in the development of the technology of coating wood surfaces with inorganic or organic films, driven by their excellent characteristics of responsive functions and environmental purification.8–13
Photochromic materials can change their colors reversibly in response to light and some successful applications such as optical storage, memory devices, and display and communications systems have been reported.14–16 Compared with the organic photochromic materials,17,18 inorganic photochromic materials exhibited a broad developing prospect due to the excellent thermostability, good fatigue resistance, and controllable size and shape.19,20 Recently, the nanosized tungsten trioxide with different structures and morphologies presents excellent performances in energy-saving smart windows, high contrast displays and gas sensors.21–28 Thus, various methods have been developed to fabricate tungsten trioxide films, such as sol–gel,29 magnetron sputtering,30 chemical vapor deposition,31 hydrothermal32 and anodic oxidation.33 Among these, the hydrothermal deposition is one of the most competitive methods because it can accurately control the film microstructures. Talebian et al. found the content of isopropyl alcohol in the precursor solution may effectively control both the nucleation and the growth orientation of the ZnO crystallite on the substrate.34 This suggested that the using of different inducers in the precursor solution may be a good choice to obtain other oxide film materials. Therefore, using ethanol as the inducer, a one-step hydrothermal approach was first developed to grow tungsten trioxide films on wood surfaces. To our best knowledge, there is no report regarding the photoresponsive and wetting performances of wood surfaces deposited by nanostructured WO3.
In this study, WO3 nanosheets were grown on wood surfaces through a simple low-temperature hydrothermal method with the precursor solution using ethanol as the inducer. The as-prepared wood products can be applied in fields of information storage, smart sensors, and anti-fake materials. The effects of ethanol content on the morphologies, crystallites, chemical components and optical properties of the films were examined. The photoresponsive and wetting properties of wood surfaces were investigated.
2. Material and methods
2.1. Materials
Sodium tungstate dihydrate (99.5%) and sulfuric acid (95.0–98.0%) used in this study were supplied by Tianjin Rgent Chemical Reagent Co., Ltd. The ethanol (99.7%) and acetone (99.5%) were purchased from Tianjian Kaitong Chemical Reagent Co., Ltd. The octadecyltrichlorosilane (OTS) (95.0%) used as surface hydrophobic modification was purchased from Shanghai Aladdin Chemical Co., Ltd. All of the chemicals were used as received without further purification. The birch veneers with a size of 25 mm × 25 mm × 0.6 mm were obtained from a plywood mill in Harbin, China. The veneer specimens were ultrasonically treated by distilled water, acetone and ethanol for 20 min, respectively, and then dried in a vacuum oven at 65 °C for 24 h.
2.2. Preparation of WO3 film coated on wood surface
The synthetic route of WO3 film growth on wood surface was as follows. Firstly, the 0.97 g of Na2WO4·2H2O was dissolved into 50 ml of the precursor solution (the solvent was distilled water and the content of ethanol was adjusted at 0%, 2%, 10%, 20%, 30% and 40%, respectively) at room temperature, followed by being acidified to 1.0 of pH value using the H2SO4 solution. The mixed solution was conveyed into a stainless steel autoclave. After the wood specimens were placed into the reaction solution, the autoclave was sealed and maintained at 110 °C for 24 h, and then cooled down to room temperature. Finally, the white films were formed on the wood surfaces and then treated by the ethanol and distilled water for several times and dried in an oven at 60 °C. The chemical reactions taking place during the film deposition can be described as: H2WO4 → WO3 + H2O.
2.3. Establishment of superhydrophobic wood surface
The surface modification of WO3 film coated on wood was performed using a self-assembly of OTS monolayer. First, the as-prepared samples were submerged into a 25 ml of 2.0% OTS/chloroform solution at room temperature for 1.5 h, followed by rinsing with chloroform for three times. Then the above samples were dried in an oven at 45 °C for 3 h. Finally, the wood surface with superhydrophobic property was obtained. The repellency of wood surface toward water droplet including apparent contact angle, advancing contact angle (ACA) and receding contact angle (RCA) was measured using a contact angle (CA) analyzer (JC2000C).
2.4. Characterization
The morphologies and chemical compositions of the WO3-treated wood surfaces were determined by the scanning electron microscopy (SEM, Quanta 200) and X-ray energy dispersive spectrometer (EDS). Crystalline structures were identified by the X-ray diffraction technique (XRD, Rigaku D/max2200 diffractometer) using a Cu Kα radiation generator operated at 1200 W (40 kV × 30 mA). The Fourier transform infrared spectroscopy (FTIR, Nicolet, Thermo Fisher Scientific) was also utilized for characterizing the products. XPS was used for chemical state analysis of the product. XPS signals were recorded with a K-Alpha XPS Analyzer (ThermoFisher Scientific Company) using an Al Kα source. Optical properties of the resulting wood samples were measured by UV-vis diffuse reflectance spectroscopy (TU-1901) with BaSO4 as the baseline correction. Atomic force microscopy (AFM) images were obtained through a Multimode Nanoscope IIIa controller (Veeco Inc., USA) with a silicon tip operated in a tapping mode to characterize the surface morphology and roughness.
2.5. Photochromic property study
The photochromic properties of the WO3-treated wood samples were determined using a color difference meter (Minolta Lab Color test cm2300d, Japan) with illuminant D65 and 10° observer. The color difference (ΔC) of wood samples were characterized after being exposed to a UV lamp device (a sealed box, containing 2 parallel UV tubular lamp in it, each UV lamp possessed a wavelength of 365 nm, 3 W and the samples were 15 cm distance from the lamps). The Commission International de'Eclairage Lab uniform color space for the color sheet system was used to calculate the color difference. L*, a* and b* are the three-dimensional rectangular coordinates, which are worked out from the tristimulus values. L* refers to the lightness index while a* and b* refer to the chromaticity indexes. The color difference was calculated according to the following equations:
where ΔC shows the color difference of the corresponding WO3-treated woods before and after being irradiated.
3. Results and discussion
3.1. Microstructures of wood surfaces treated by WO3
Fig. 1 presents the SEM images of the control wood and the obtained films coated on wood substrates to reveal the effect of ethanol content on the growth process of the WO3 film. In Fig. 1a, the pristine wood surface appears to be smooth and free of any other materials. In Fig. 1b, the film prepared with pure distilled water exhibits much smaller nanosheets aggregating on the wood surfaces. However, when the ethanol content was increased to 20%, the nanostructures varied to large and dispersed 2D nanosheets with an edge length of 580–957 nm and a thickness of about 80 nm, as shown in Fig. 1e. When the ethanol content was further increased to 40%, the sizes of the nanosheets were larger (Fig. 1g). The reason was that the energy barrier of the particle to agglomerate is primarily determined by dielectric constant and surface potential. There was the reference showing the values of the dielectric constants of the ethanol–water solutions as a function of ethanol content (Table 1).35 The greater the ethanol–water ratio, the lower the dielectric constant of the solvent, reduced the polarity of particles and electrostatic attraction among particles. Therefore, higher ethanol content made the greater crystal growth rate than the nucleation rate, resulting in size increase of particle.36 Fig. 1h shows the EDS spectra of the film coated on wood surface. The presence of a significant W peak at around 1.8 keV in the spectrum of the sample predicated that WO3 particles were grown on the wood surface through the hydrothermal process.
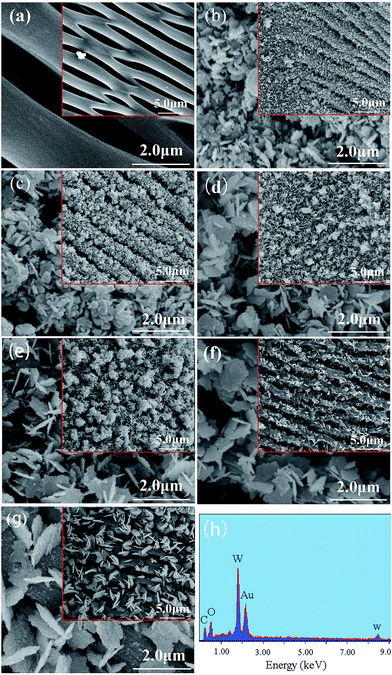 |
| Fig. 1 SEM images of (a) the control wood, and the films prepared with different ethanol content: (b) 0%; (c) 2%; (d) 10%; (e) 20%; (f) 30%; (g) 40%, and EDS spectra of (h) the obtained film coated on wood surface. | |
Table 1 Values of the dielectric constants of the ethanol–water solutions as a function of ethanol content
Ethanol content |
0% |
20% |
40% |
Dielectric constant |
78.4 |
67.0 |
55.0 |
3.2. Phase and composition analyses
Fig. 2a displays the XRD patterns of the pristine wood, the pure WO3 film prepared with the 20% ethanol content, and the WO3-treated woods prepared with the ethanol contents of 0%, 20% and 40%, respectively. The diffraction peaks at 15.4° and 22.3° are attributed to the (101) and (002) diffraction planes of cellulose in the wood. At the 0% or 20% of ethanol content, other diffraction peaks are assigned to the (100), (001), (110), (101), (200), (111), (201), (211), (220), (221), (400) and (401) diffraction planes of hexagonal WO3 structures (JCDS card no. 75-2187). Moreover, at the 20% of ethanol content, the peak intensity of (200) was increased, indicating the higher crystallinity. However, when the ethanol content was increased to 40%, the peaks intensities were reduced but the number of peaks was increased. The reason can be explained as that polymorphism prevailed at the 40% of ethanol content. In addition, under the same condition (20% ethanol content), peaks intensities of the WO3 film loaded on wood surfaces were reduced compared with pure WO3 film. This is probably ascribed to the rough structures of wood surfaces. Fig. 2b shows FT-IR spectra of the pristine wood and the obtained film on wood surface. Compared with the pristine wood, a remarkable peak at 813 cm−1 appeared on the as-prepared film. The evidence for the structural determination of WO3 crystal is the peak position of the stretching vibration of O–W–O located at about 820 cm−1.37 In general, the IR band of O–W–O in crystalline WO3 is seen at 600–900 cm−1.38 Hence, the band at 813 cm−1 is ascribed to the O–W–O stretching and bending modes of the bridging oxygen atoms, suggesting that WO3 had successfully grown on wood surfaces. The result was in accordance with that obtained from XRD.
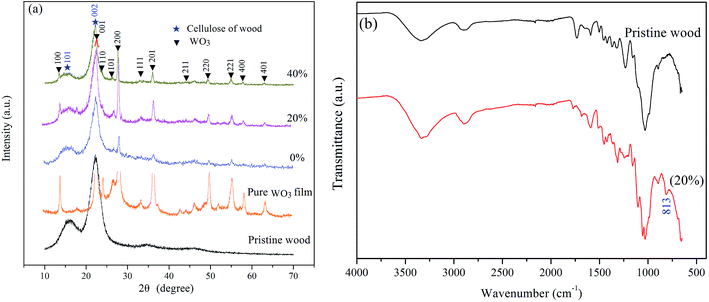 |
| Fig. 2 XRD patterns and FT-IR spectra of pristine wood, pure WO3 film, and the obtained WO3-treated woods. | |
3.3. XPS analysis
For the sake of clarification of the surface chemical composition and electronic structure, XPS analysis was applied to characterize the WO3-treated wood prepared with the 20% ethanol content. The peak positions in all of the XPS spectra are calibrated with C 1s at 284.6 eV. From the wide-scan XPS in Fig. 3a, it can be seen that all indexed peaks were ascribed to W, O, and C elements. In order to further investigate binding state, narrow-scan Gaussian–Lorentz fitting curves for W and O elements were carried out. In Fig. 3b, the peak observed at 35.95 eV and 38.11 eV binding energies corresponded to the W6+ state in the WO3 thin film. The energy separation between the main peaks of W 4f7/2 and W 4f5/2 was 2.16 eV which agreed with the tungsten in the W6+ valence state and also suggested the nominal stoichiometry of the WO3 thin film.39,40 The O 1s spectrum illustrated in Fig. 3c could be deconvoluted as two peaks. The major one observed at 530.68 eV was ascribed to O 1s in the W–O bond configuration, while the other one centered at 532.94 eV was probably assigned to the chemisorbed oxygen of the surface hydroxyl.41 Based on the above discussions, it was confirmed that the WO3 film had been well coated on wood surface.
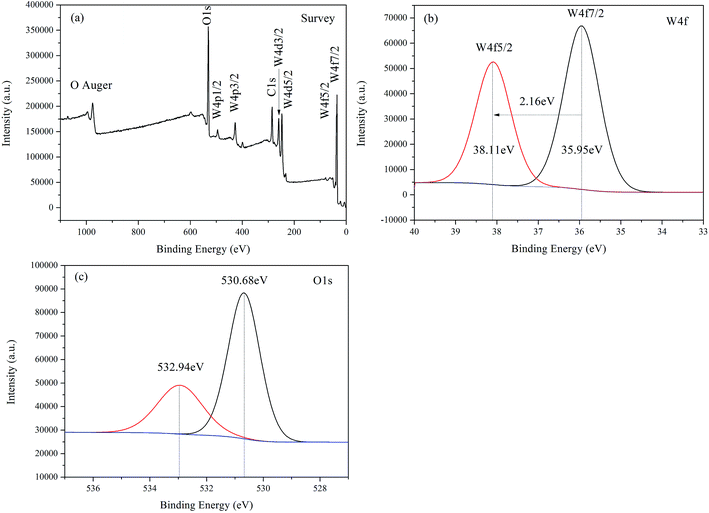 |
| Fig. 3 XPS survey spectra (a) of the WO3-treated wood, the XPS curves for W 4f (b) and O 1s (c). | |
3.4. Optical and photochromic properties
Fig. 4a displays the UV-vis absorption spectra of the WO3-treated woods prepared with the 0%, 20% and 40% of ethanol contents, and the inset shows the evaluation of the optical band gap of the WO3 film prepared with the 20% of ethanol content. As it was obvious, all the samples responded to ultraviolet radiation of wavelength in 270–350 nm very intensively, with threshold values at around 420 nm, according with the intrinsic absorption edge of the pristine WO3.42 However, the sample prepared with the 20% of ethanol content shows a remarkable red shift compared with the two synthesized at the 0% and 40%, suggesting a larger light absorption range. Furthermore, the estimated band gap of the sample prepared with the 20% of ethanol content was around 3.17 eV, consistent with the band gap of WO3.43 After the sample was exposed to a UV light (365 nm), the peak of the sample was changed to 273.36 nm.
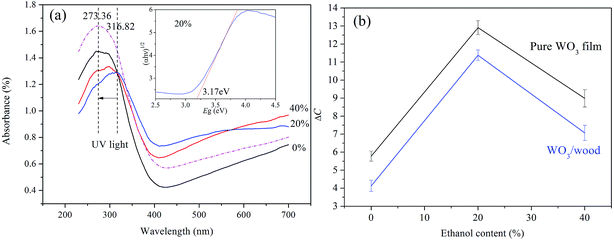 |
| Fig. 4 UV-vis absorption spectra of WO3-treated woods, the inset for the evaluation of the optical band gap using the Tauc plot, and the color differences (ΔC) of WO3-treated woods and pure WO3 films exposed under UV lamp (365 nm). | |
Fig. 4b shows the color difference values (ΔC) of WO3-treated wood samples and pure WO3 films irradiated by UV light. It is seen that the photoresponsive behavior of the sample or film prepared with the 20% of ethanol content was greater than that prepared with the 0% and 40%. In addition, under the same preparation condition, the photoresponsive behavior of the pure WO3 film was slightly higher than the WO3-treated wood sample. This probably depended on the rough surface of the original wood sample.
3.5. Photochromic mechanism
The photochromic mechanism of the WO3 film can be elucidated by the model of double insertion-extraction of ions and electrons, as shown in Fig. 5. When the WO3 film was irradiated with UV light, electrons (e−) and holes (h+) formed. Resulting from water absorbed on the surface or interior, the necessary protons (H+) for the coloration can be produced from the reaction of the absorbed water with the holes. Subsequently the photogenerated electrons injected into the conductive band (CB) of WO3 reacted with H+ and WO3, and thus hydrogen tungsten bronze (HxWVxWVI1−xO3) was produced. Accordingly, the WO3 film turned blue due to the intervalence charge transfer from the valence band of W5+ to the conduction band of W6+.44 Therefore, the capacity of the WO3 film capturing photons determined the amount of photoinduced electrons–hole pairs, which directly influenced the photoresponsive behavior of the WO3 film. Based on the SEM and XRD analyses of samples, it was known that the sample prepared with the 20% of ethanol content exhibited the larger crystals with hexagonal structures. Moreover, the UV-vis analysis also shows that the above sample possessed a larger light absorption range. However, at the 40% of ethanol content, the photochromic activity of the obtained sample was reduced. This is due to the presence of different bandgaps and symmetry elements corresponding to different co-existing crystal structures from XRD observations.
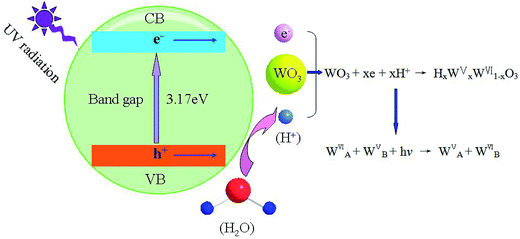 |
| Fig. 5 Schematic representation of the photochromic mechanism of WO3-treated woods exposed under UV lamp (365 nm). | |
3.6. WCAs measurement
Fig. 6 depicts the WCAs of the pristine wood surface, WO3-treated wood surfaces and WO3-treated wood surfaces modified by the octadecyltrichlorosilane (OTS). The pristine wood surface exhibits the 70.3° of WCA and the value increased to 127.4° through the OTS treatment. However, the WO3-treated wood surface shows about 0° of WCA. However, it was more notable that WO3-treated wood surface possessed the superhydrophobic behavior after the OTS treatment. In order to fully understand the superhydrophobic properties of samples, advancing contact angle (ACA) and receding contact angle (RCA) was also presented. Moreover, a quartz glass substrate was used to find out whether wood had any specific role to play in hydrophilic and hydrophobic aspects. The results show that the quartz glass surface also had the superhydrophilic behavior after coating WO3 film and changed to superhydrophobic performance after coating OTS (Table 2). The schematic illustration of the synthetic process of the superhydrophobic wood are explained in Fig. 7. Through a hydrothermal process, the WO3 particles were grown on the pristine wood surface. Subsequently, the hydroxyl groups resulting from the OTS hydrolysis were responsible for bonding the surface hydroxyl groups of the WO3 and eventually the covalent bonds of the Si–O–W were generated. The sheet-like WO3 nanostructures and the long-chain hydrophobic alkyls probably determined the large surface roughness, which created the superhydrophobic property.
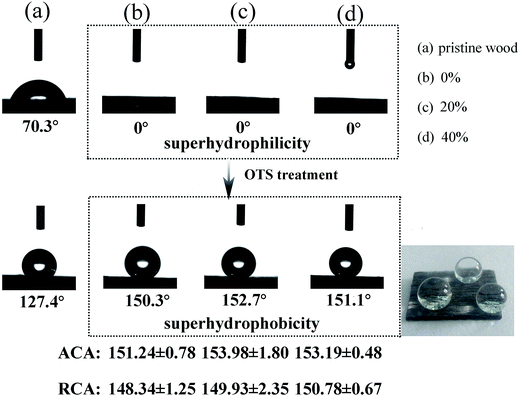 |
| Fig. 6 WCAs of pristine wood surface, WO3-treated wood surfaces and WO3-treated wood surfaces modified by OTS. | |
Table 2 Contact angles of quartz glass substrate, quartz glass-WO3, and quartz glass-WO3-OTS
Samples |
Quartz glass |
Quartz glass-WO3 |
Quartz glass-WO3-OTS |
WCAs |
32° |
0° |
150.1° |
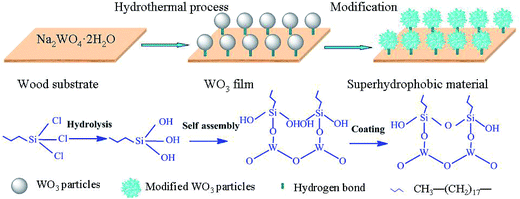 |
| Fig. 7 Schematic illustration of the synthetic process of the superhydrophobic wood treated by WO3 and OTS. | |
3.7. Photochromic and superhydrophobic analyses
In order to explore the relations between surface roughness and photochromic and superhydrophobic performances, the AFM images of samples are presented in Fig. 8. Compared with the untreated wood surface (Fig. 8a), the WO3-treated wood revealed a fine microstructure and more complex surface texture which agreed with a rough topography (Fig. 8b). After being irradiated under UV light, the sample became rougher (Fig. 8c). The wood surface treated by WO3 and OTS consisted of high “mountains” and deep “valleys” (Fig. 8d). The difference of the surface roughness can be reflected in the root mean square (RMS) roughness values.45–47 The RMS roughness values of four surfaces were 45.71 nm (the untreated wood), 51.84 nm (the WO3-treated wood) 68.15 nm (the WO3-treated wood under UV light irradiation), and 81.31 nm (the wood treated by WO3 and OTS), respectively. Therefore, it can be concluded that the modified surface topology was responsible for the film with photoresponsive and hydrophobic features, as a result of a change in surface roughness.
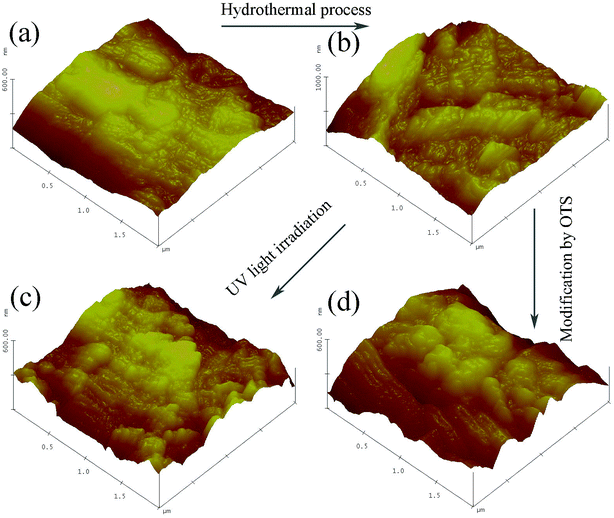 |
| Fig. 8 AFM images of (a) the pristine wood, (b) the WO3-treated wood, (c) the WO3-treated wood under UV light irradiation, and (d) the wood treated by WO3 and OTS. | |
4. Conclusions
The sheet-like WO3 nanostructures were grown on wood surfaces through a simple low-temperature hydrothermal method using ethanol as the inducer. It was found by the SEM and XRD analyses that the ethanol had great contribution to the morphologies and phase structures of the WO3 films. The WO3 films made up of 2D nanosheets with a higher crystallinity were prepared with the precursor solution with the 20% ethanol content. The wood surfaces based on WO3 films exhibited good sensitivity to excitation light sources, and the one prepared with the 20% of ethanol content performed even better. The WCA measurements demonstrated that the wettability of WO3 film deposited on wood surface altered the property from superhydrophilicity to superhydrophobicity through the simple OTS treatment. The photoresponsive and hydrophobic performances were attributed to the larger RMS roughness value through the AFM analyses. Due to the photoresponsive and superhydrophobic functions, these modified wood products have promising applications in fields of information storage, smart sensors, and anti-fake materials.
Acknowledgements
This work was financially supported by The National Natural Science Foundation of China (31470584), Fundamental Research Funds for the Central Universities (2572015AB06) and Research Funds for Bionic Photoresponse of Wood and Intelligent Property (2572014CBY01).
References
- P. Börjesson and L. Gustavsson, Greenhouse gas balances in building construction: wood versus concrete from life-cycle and forest land-use perspectives, Energy Policy, 2000, 28, 575–588 CrossRef
. - M. Worgull, M. Schneider, M. Röhrig, M. Röhrig, T. Meier, M. Heilig, A. Kolew, K. Feit, H. Hölscher and J. Leuthold, Hot embossing and thermoforming of biodegradable three-dimensional wood structures, RSC Adv., 2013, 3, 20060–20064 RSC
. - M. Qu, P. Pelkonen, L. Tahvanainen, J. Arevalo and D. Gritten, Experts' assessment of the development of wood framed houses in China, J. Cleaner Prod., 2012, 31, 100–105 CrossRef PubMed
. - L. Wang, A. Toppinen and H. Juslin, Use of wood in green building: a study of expert perspectives from the UK, J. Cleaner Prod., 2014, 65, 350–361 CrossRef PubMed
. - M. A. Ermeydan, E. Cabane, N. Gierlinger, J. Koetz and I. Burgert, Improvement of wood material properties via in situ polymerization of styrene into tosylated cell walls, RSC Adv., 2014, 4, 12981–12988 RSC
. - P. D. Evans, A. F. A. Wallis and N. L. Owen, Weathering of chemically modified wood surfaces, Wood Sci. Technol., 2000, 34, 151–165 CrossRef CAS
. - Y. Meng, Y. Xia, T. M. Young, Z. Cai and S. Wang, Viscoelasticity of wood cell walls with different moisture content as measured by nanoindentation, RSC Adv., 2015, 5, 47538–47547 RSC
. - S. Bourbigot and S. Duquesne, Fire retardant polymers: recent developments and opportunities, J. Mater. Chem., 2007, 17, 2283–2300 RSC
. - S. Trey, R. T. Olsson, V. Ström, L. Berglund and M. Johansson, Controlled deposition of magnetic particles within the 3-D template of wood: making use of the natural hierarchical structure of wood, RSC Adv., 2014, 4, 35678–35685 RSC
. - C. T. Hsieh, B. S. Chang and J. Y. Lin, Improvement of water and oil repellency on wood substrates by using fluorinated silica nanocoating, Appl. Surf. Sci., 2011, 257, 7997–8002 CrossRef CAS PubMed
. - B. Hui, J. Li and L. Wang, Electromagnetic shielding wood-based composite from electroless plating corrosion-resistant Ni–Cu–P coatings on Fraxinus mandshurica veneer, Wood Sci. Technol., 2014, 48, 961–979 CrossRef CAS
. - M. N. Kavalenka, A. Hopf, M. Schneider, M. Worgull and H. Hölscher, Wood-based microhaired superhydrophobic and underwater superoleophobic surfaces for oil/water separation, RSC Adv., 2014, 4, 31079–31083 RSC
. - T. Keplinger, E. Cabane, M. Chanana, P. Hass, V. Merk, N. Gierlinger and I. Burgert, A versatile strategy for grafting polymers to wood cell walls, Acta Biomater., 2015, 11, 256–263 CrossRef CAS PubMed
. - S. Pu, T. Yang, B. Yao, Y. Wang, M. Lei and J. Xu, Photochromic diarylethene for polarization holographic optical recording, Mater. Lett., 2007, 61, 855–859 CrossRef CAS PubMed
. - S. Kawata and Y. Kawata, Three-dimensional optical data storage using photochromic materials, Chem. Rev., 2000, 100, 1777–1788 CrossRef CAS PubMed
. - J. C. Crano, T. Flood, D. Knowles, A. Kumar and B. van Gemert, Photochromic compounds: chemistry and application in ophthalmic lenses, Pure Appl. Chem., 1996, 68, 1395–1398 CrossRef CAS
. - R. Gao, D. Cao, Y. Guan and D. Yan, Flexible self-supporting nanofiber thin films showing reversible photochromic fluorescence, ACS Appl. Mater. Interfaces, 2015, 7, 9904–9910 CAS
. - R. Gao, Y. Zhao, X. Yang and D. Yan, Stimuli-responsive fluorescence based on the solid-state bis[2-(2-benzothiazoly)phenolato]zinc(II) complex and its fiber thin film, RSC Adv., 2015, 5, 56470–56477 RSC
. - Y. Ohko, T. Tatsuma, T. Fujii, K. Naoi, C. Niwa, Y. Kubota and A. Fujishima, Multicolour photochromism of TiO2 films loaded with silver nanoparticles, Nat. Mater., 2003, 2, 29–31 CrossRef CAS PubMed
. - Z. Jiao, J. Wang, L. Ke, X. W. Sun and H. V. Demir, Morphology-tailored synthesis of tungsten trioxide (hydrate) thin films and their photocatalytic properties, ACS Appl. Mater. Interfaces, 2011, 3, 229–236 CAS
. - G. R. Bamwenda and H. Arakawa, The visible light induced photocatalytic activity of tungsten trioxide powders, Appl. Catal., A, 2001, 210, 181–191 CrossRef CAS
. - L. Liu, S. Guo, X. Zhang, M. Sun and Y. Zhao, Designable fabrication of hierarchical WO3·H2O hollow microspheres for enhanced visible light photocatalysis, RSC Adv., 2015, 5, 16376–16385 RSC
. - D. Chen, X. Hou, H. Wen, Y. Wang, H. Wang, X. Li, R. Zhang, H. Lu, H. Xu, S. Guan, J. Sun and L. Gao, The enhanced alcohol-sensing response of ultrathin WO3 nanoplates, Nanotechnology, 2010, 21, 035501 CrossRef PubMed
. - J. C. Chou and J. L. Chiang, Ion sensitive field effect transistor with amorphous tungsten trioxide gate for pH sensing, Sens. Actuators, B, 2000, 62, 81–87 CrossRef CAS
. - Y. Liu, H. He, J. Li, W. Li, Y. Yang, Y. Li and Q. Chen, ZnO nanoparticles-functionalized WO3 nanoplates with enhanced photoelectrochemical properties, RSC Adv., 2015, 5, 46928–46934 RSC
. - W. Mickelson, A. Sussman and A. Zettl, Low-power, fast, selective nanoparticle-based hydrogen sulfide gas sensor, Appl. Phys. Lett., 2012, 100, 173110 CrossRef PubMed
. - C. Santato, M. Ulmann and J. Augustynski, Photoelectrochemical properties of nanostructured tungsten trioxide films, J. Phys. Chem. B, 2001, 105, 936–940 CrossRef CAS
. - G. Xi, J. Ye, Q. Ma, N. Su, H. Bai and C. Wang, In situ growth of metal particles on 3D urchin-like WO3 nanostructures, J. Am. Chem. Soc., 2012, 134, 6508–6511 CrossRef CAS PubMed
. - M. Breedon, P. Spizzirri, M. Taylor, J. du Plessis, D. McCulloch, J. Zhu, L. Yu, Z. Hu, C. Rix, W. Wlodarski and K. Kalantar-zadeh, Synthesis of nanostructured tungsten oxide thin films: a simple, controllable, inexpensive, aqueous sol–gel method, Cryst. Growth Des., 2009, 10, 430–439 Search PubMed
. - A. Subrahmanyam and A. Karuppasamy, Optical and electrochromic properties of oxygen sputtered tungsten oxide (WO3) thin films, Sol. Energy Mater. Sol. Cells, 2007, 91, 266–274 CrossRef CAS
. - Z. L. Li, F. Liu, N. S. Xu, J. Chen and S. Z. Deng, A study of control growth of three-dimensional nanowire networks of tungsten oxides: from aligned nanowires through hybrid nanostructures to 3D networks, J. Cryst. Growth, 2010, 312, 520–526 CrossRef CAS PubMed
. - D. Ding, Y. Shen, Y. Ouyang and Z. Li, Hydrothermal deposition and photochromic performances of three kinds of hierarchical structure arrays of WO3 thin films, Thin Solid Films, 2012, 520, 7164–7168 CrossRef CAS PubMed
. - S. S. Kalanur, Y. J. Hwang, S. Y. Chae and O. S. Joo, Facile growth of aligned WO3 nanorods on FTO substrate for enhanced photoanodic water oxidation activity, J. Mater. Chem. A, 2013, 1, 3479–3488 CAS
. - N. Talebian, M. R. Nilforoushan and N. Maleki, Ultraviolet to visible-light range photocatalytic activity of ZnO films prepared using sol–gel method: the influence of solvent, Thin Solid Films, 2013, 527, 50–58 CrossRef CAS PubMed
. - M. A. Esteso, O. M. Gonzalez-Diaz, F. F. Hernandez-Luis and L. Fernandez-Merida, Activity coefficients for NaCl in ethanol–water mixtures at 25 °C, J. Solution Chem., 1989, 18, 277–288 CrossRef CAS
. - Y. Shen, D. Ding and Y. Deng, Fabrication and characterization of WO3 flocky microspheres induced by ethanol, Powder Technol., 2011, 211, 114–119 CrossRef CAS
. - M. F. Daniel, B. Desbat, J. C. Lassegues, B. Gerand and M. Figlarz, Infrared and Raman study of WO3 tungsten trioxides and WO3, xH2O tungsten trioxide tydrates, J. Solid State Chem., 1987, 67, 235–247 CrossRef CAS
. - C. Guery, C. Choquet, F. Dujeancourt, J. M. Tarascon and J. C. Lassegues, Infrared and X-ray studies of hydrogen intercalation in different tungsten trioxides and tungsten trioxide hydrates, J. Solid State Electrochem., 1997, 1, 199–207 CrossRef CAS
. - Y. F. Lu and H. Qiu, Laser coloration and bleaching of amorphous WO3 thin film, J. Appl. Phys., 2000, 88, 1082–1087 CrossRef CAS PubMed
. - A. J. More, R. S. Patil, D. S. Dalavi, S. S. Mali, C. K. Hong, M. G. Gang, J. H. Kim and P. S. Patil, Electrodeposition of nano-granular tungsten oxide thin films for smart window application, Mater. Lett., 2014, 134, 298–301 CrossRef CAS PubMed
. - B. Chai, J. Li, Q. Xu and K. Dai, Facile synthesis of reduced graphene oxide/WO3 nanoplates composites with enhanced photocatalytic activity, Mater. Lett., 2014, 120, 177–181 CrossRef CAS PubMed
. - K. J. Patel, C. J. Panchal, V. A. Kheraj and M. S. Desai, Growth, structural, electrical and optical properties of the thermally evaporated tungsten trioxide (WO3) thin films, Mater. Chem. Phys., 2009, 114, 475–478 CrossRef CAS PubMed
. - J. Su, X. Feng, J. D. Sloppy, L. Guo and C. A. Grimes, Vertically aligned WO3 nanowire arrays grown directly on transparent conducting oxide coated glass: synthesis and photoelectrochemical properties, Nano Lett., 2010, 11, 203–208 CrossRef PubMed
. - A. I. Gavrilyuk, Photochromism in WO3 thin films, Electrochim. Acta, 1999, 44, 3027–3037 CrossRef CAS
. - K. Boussu, B. van der Bruggen, A. Volodin, J. Snauwaert, C. van Haesendonck and C. Vandecasteele, Roughness and hydrophobicity studies of nanofiltration membranes using different modes of AFM, J. Colloid Interface Sci., 2005, 286, 632–638 CrossRef CAS PubMed
. - G. Krishnamoorthy, S. Sadulla, P. K. Sehgal and A. B. Mandal, Greener approach to leather tanning process: D-lysine aldehyde as novel tanning agent for chrome-free tanning, J. Cleaner Prod., 2013, 42, 277–286 CrossRef CAS PubMed
. - W. Shen, C. Zhang, Q. Li, W. Zhang, L. Cao and J. Ye, Preparation of titanium dioxide nano particle modified photocatalytic self-cleaning concrete, J. Cleaner Prod., 2015, 87, 762–765 CrossRef CAS PubMed
.
|
This journal is © The Royal Society of Chemistry 2015 |