DOI:
10.1039/C5RA10461K
(Paper)
RSC Adv., 2015,
5, 59240-59250
Regioselective synthesis of novel 2,3,4,4a-tetrahydro-1H-carbazoles and their cholinesterase inhibitory activities†
Received
2nd June 2015
, Accepted 25th June 2015
First published on 25th June 2015
Abstract
The present study describes the regioselective synthesis of novel 2,3,4,4a-tetrahydro-1H-carbazoles (syn-2(a–q) and anti-3(a–b)) from L-menthone via Fischer indole synthesis. The reaction between L-menthone and different substituted phenyl hydrazines, carried out in acetic acid as catalyst and solvent, yielded a mixture of corresponding 2,3,4,4a-tetrahydro-1H-carbazole diastereomers, instead of the expected indoles. These were characterized by different spectroscopic and single-crystal X-ray diffraction techniques. The isolated single diastereoisomers were further evaluated for inhibitory activities against various enzymes such as cholinesterases, xanthine oxidase, α-chymotrypsin, urease, phosphodiesterase and carbonic anhydrase-II activity, as well as against DPPH radical scavenging and anti-cancer activity against PC-3 cell line. The 2,3,4,4a-tetrahydro-1H-carbazoles show promising inhibitory activities only against acetyl- and butyrylcholinesterase. Moreover, kinetic analyses were carried out for the study of mechanism of inhibition of the active compounds.
1. Introduction
Alzheimer's disease (AD) is a neurodegenerative disorder, characterized by progressive dementia and other cognitive deficits. It is estimated as being the sixth leading cause of death in the US, that affects one in eight older individuals.1 Though hallmarks associated with the pathophysiology of AD are β-amyloid rich senile plaques and neurofibrillary tangles, several other factors such as oxidative stress and low levels of acetylcholine have also been found to play an important role in the progression of the disease.2 The low level of acetylcholine is attributed to the reduced activity of choline acyltransferase or increased activity of cholinesterase enzymes (AChE and BChE). Therefore, inhibition of cholinesterases has emerged as a promising strategy for AD therapy through enhancing cholinergic function.3
In normal healthy brain, hydrolytic action of AChE is higher than BChE, while in AD patient ratio of AChE to BChE is dramatically decreased. Therefore, BChE is dominantly involved in hydrolytic activity and cholinergic dysfunction in AD brain. In addition, increased level of BChE is also involved in the cleavage of amyloid precursor protein into β-amyloid, which accumulates to form β-amyloid plaques.4,5 Thus, selective BChE inhibitors are involved in augmenting cholinergic function and preventing the plaques formation, therefore controlling the pathogenesis of disease. Currently many drugs are available for the management of AD, such as tacrine and donepezil,6 but they exhibit poor selectivity, bioavailability and adverse side effects on peripheral nervous system3 and liver.7,8 Thus, the need for novel anti-Alzheimer's agents driven us to evaluate novel indole-type derivatives as cholinesterase inhibitors. Indole derivatives have inspired chemists due to their natural origin and diverse pharmacological properties,9–11 such as neuroprotective,12 glucokinase,13 anti-oxidant,14 anti-cancer15 etc. Besides these activities, the indole scaffold is also considered as a promising target for cholinesterase inhibition.16,17 Furthermore, alkyl substitution at the pyrrole part of indole often improves activity, such as in β-carboline, tetrahydrocarbazoles, and serotonin derivatives.18
Due to great structural diversity of biologically active indoles, various methods have been developed for their synthesis.19–21 However, all such methods make use of expensive catalysts and long reaction times. Herein, we report the synthesis of 2,3,4,4a-tetrahydro-1H-carbazole (3[H]-indole) derivatives from L-menthone and substituted phenyl hydrazine via transition-metal free Fischer indolization reaction and evaluate them for cholinesterase inhibition.
2. Results and discussion
2.1. Chemistry
Fischer indolization is a widely employed method for the synthesis of indoles, but in case of unsymmetrical ketones, the method plausibly results in two regioisomers, namely indole 1 and 2,3,4,4a-tetrahydro-1H-carbazole (3[H]-indole) 2/3.22 In the current study, the reaction between L-menthone and substituted phenyl hydrazine results only in diastereomeric 2,3,4,4a-tetrahydro-1H-carbazoles (syn-2(a–q) or anti-3(a–b)) in the presence of acetic acid as catalyst and solvent (Scheme 1). The diastereoselective ratio between syn- and anti-2,3,4,4a-tetrahydro-1H-carbazoles (2/3) was found to be 1
:
1–1.8
:
1 as determined by 1H NMR spectra. Silica gel column chromatography allowed isolation of the syn-2(a–q) derivatives in 30–56% yield. Of the anti-isomers, only anti-3a (39%) and 3b (35%) compounds could be obtained in pure form. All purified compounds were evaluated for various bioactivities.
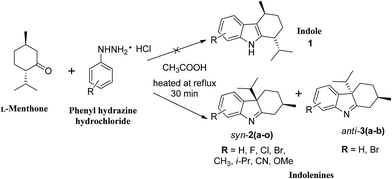 |
| Scheme 1 Regioselective synthesis of 2,3,4,4a-tetrahydro-1H-carbazoles syn-2(a–q) and anti-3(a–b). | |
A plausible mechanism shows the formation of the hydrazone 4, which undergoes tautomerism, followed by [3,3]-sigmatropic rearrangement of the enehydrazine tautomer 6. Cyclization followed by the loss of ammonia provides the 2,3,4,4a-tetrahydro-1H-carbazole skeleton, as presented in Scheme 2. In this mechanism, enehydrazine 6 is the key intermediate that determines fate of the reaction. As weak acid or low concentration of acids promotes preferentially the formation of more substituted enehydrazine (thermodynamic product), cyclization occurs at the more substituted carbon and resulting product will be 2,3,4,4a-tetrahydro-1H-carbazoles.22 In addition, indolization of L-menthone resulted in an isomeric mixture of 2,3,4,4a-tetrahydro-1H-carbazoles, in ratios given in Table 3. This happens due to epimerization of stereogenic center bearing i-Pr group. The separation of diastereomers by column chromatography yielded syn-2,3,4,4a-tetrahydro-1H-carbazole as the faster eluting product, and the anti-substituted 2,3,4,4a-tetrahydro-1H-carbazole as the slower eluting product. The structure of the faster-eluting 2,3,4,4a-tetrahydro-1H-carbazole was deduced by 13C-NMR and single-crystal X-ray diffraction studies.
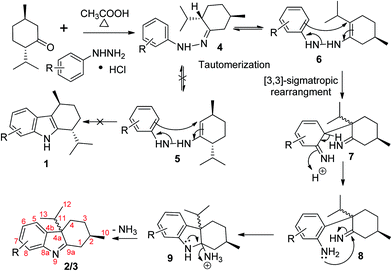 |
| Scheme 2 Plausible mechanism of 2,3,4,4a-tetrahydro-1H-carbazole. | |
The structure of compound 2j (R = 4Br) is composed of a planar 2,3,4,4a-tetrahydro-1H-carbazole ring (N-9/C-8a−C-4a/C-9a), fused with a cyclohexane ring (C-4a/C-9a−C-4) that exists in a chair form. The isopropyl (C-11−C-13) and methyl substituents (C-10), attached to C-4a and C-2, were found to occupy axial and equatorial orientations, respectively (Fig. 1). It was difficult to purify the slower moving 2,3,4,4a-tetrahydro-1H-carbazole (anti-product) through column chromatography. Thus, only two of the anti-products were fully characterized. Structural assignments were made by extensive use of 2D COSY, HSQC, HMBC and NOESY experiments.
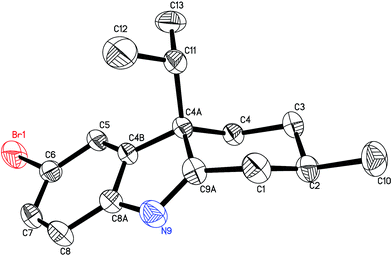 |
| Fig. 1 The ORTEP plot of compound 2j drawn at 30% probability level. | |
2.2. Spectral data of syn-2,3,4,4a-tetrahydro-1H-carbazole 2j
2,3,4,4a-Tetrahydro-1H-carbazole 2j was obtained as yellow crystals that showed the [M+] at m/z 305.0763 in high resolution (HR) EI-MS spectrum. IR showed characteristic peak for the C
N bond at 1582 cm−1. The 13C-NMR exhibited three CH3, three CH2, five CH groups and five quaternary carbon atoms, indicating an 2,3,4,4a-tetrahydro-1H-carbazole scaffold (Table 1). The methine (CH) C-11, resonating at δ 28.6 ppm, showed correlations with a septet (δ 2.28 ppm) in HSQC experiment and with the methyl protons at δ 0.28 ppm (H-12) and 1.20 ppm (H-13) in HMBC experiment, permitting assignment of an i-Pr unit. The unusually high field chemical shift of the H-12 methyl is probably due to shielding by C
N bond. Assignment of geminal protons was done using HSQC, COSY and HMBC experiments. From HSQC experiment, it was observed that two set of geminal protons, resonating at δ 2.17 ppm and 2.79 ppm and at δ 1.63 ppm and 1.47 ppm corresponds to carbons appeared at δ 37.8 ppm (C-1) and 28.9 ppm (C-3), respectively. Assignment for geminal protons at C-1 was confirmed by three bond HMBC correlation with quaternary carbon characteristic of an 2,3,4,4a-tetrahydro-1H-carbazole ring (C-9a), and isolated methine (C-2) resonating at δ 190.7 and δ 36.6, respectively. A methylene (H-4eq), resonating as a doublet of triplets at δ 2.49 showed COSY correlations with H-3ax (δ 1.50) and H-3eq (δ 1.62), which allows assignment of H-3 and H-4. The large coupling constant between H-2ax and H-3ax (J = 13.2 Hz) suggested a chair conformation in which methyl group has an equatorial orientation, which was also supported by X-ray data. The stereochemistry was assigned on the basis of NOESY experiment. Thus, NOE correlation between methyl (H-12) of isopropyl unit, isolated methyl (H-10) and one of the methylene protons, H-1 (δ 2.17), indicated that these protons are syn to each other. C-12 methyl protons (δ 1.19) showed interactions with H-4 at δ 2.49 indicating that it has an equatorial disposition. One of the methylene resonated at δ 1.50, showed cross peaks with C-12 methyl (δ 1.19) which confirmed that this H-3 is axially oriented.
Table 1 NMR spectral data of compound 2j
C# |
δC |
δH |
Mult. |
HBMC |
COSY |
NOESY |
Axial proton. Equatorial proton. |
1a |
37.8 |
2.18 (t) |
CH2 |
C-2, C-6, C-9a, C-10 |
H-1b |
— |
1b |
— |
2.80 (ddd) |
CH2 |
C-4a, C-9a |
— |
H-1a |
2 |
36.6 |
1.68–1.59 (m) |
CH |
— |
— |
H-1b |
3a |
28.9 |
1.51 (qd) |
CH2 |
— |
H-4b |
— |
3b |
— |
1.68–1.59 (m) |
CH2 |
— |
H-4b |
H-4b |
4a |
33.4 |
1.01 (td) |
CH2 |
C-8a, C-10 |
H-3a, H-3b, H-4b |
H-3a, H-4b, H-4b |
4b |
- |
2.50 (dt) |
CH2 |
C-2, C-9a |
— |
— |
4a |
61.3 |
— |
C |
— |
— |
— |
4b |
130.3 |
— |
C |
— |
— |
— |
5 |
126.3 |
7.43–7.39 (m) |
CH |
C-4a, C-5, C-6, C-7, C-8a, C-8 |
— |
— |
6 |
154.4 |
— |
C |
— |
— |
— |
7 |
130.3 |
7.43–7.39 (m) |
CH |
C-4b, C-5, C-6, C-7, C-8a, C-8 |
— |
— |
8 |
121.1 |
7.43–7.39 (m) |
CH |
C-4b, C-5, C-6, C-7, C-8a, C-8 |
— |
— |
8a |
144.8 |
— |
C |
— |
— |
— |
9a |
190.7 |
— |
C |
— |
|
|
10 |
21.9 |
1.12 (d) |
CH3 |
C-1, C-2, C-3, C-4a, C-9a |
H-2 |
H-1a, H-1b, H-3a, H-3b |
11 |
28.6 |
2.30 (sep) |
CH |
C-4a, C-8a, C-9a, C-12 |
— |
— |
12 |
16.8 |
1.20 (d) |
CH3 |
C-4a, C-10, C-11 |
H-11 |
H-4b, H-10 |
13 |
16.2 |
0.28 (d) |
CH3 |
C-4a, C-11, C-12 |
H-11 |
H-1b, H-11, H-10 |
2.3. Spectral data of anti-2,3,4,4a-tetrahydro-1H-carbazole 3b
Anti-2,3,4,4a-tetrahydro-1H-carbazole 3b was obtained as a yellow gummy solid, with the [M+] at m/z 305.0761 in HR EI-MS spectrum, while the IR spectrum showed peak at 1584 cm−1 characteristic of an 2,3,4,4a-tetrahydro-1H-carbazole moiety. The 13C-NMR showed three methyl, three methylene, five methine (three aromatic) and five quaternary carbons, indicating an isomeric 2,3,4,4a-tetrahydro-1H-carbazole (Table 2). Methine (δ 2.33) showed COSY correlations with H-12 and H-13, which allowed assignment of i-Pr unit. This was also confirmed by HMBC correlations of methyl protons at δ 0.26 with C-11 (δ 28.6) and C-12 (δ 17.1) signals. Triplet of doublet (δ 1.26) and multiplet (δ 2.33) were identified as methylene protons correlating with a carbon signal at δ 29.3 (C-4) in HSQC. While, correlation of methine C-11 (δ 28.6) with multiplet at δ 2.33 indicated that this signal is an overlap of two proton signals H-11 and H-4. From HSQC experiment, it was observed that m + d (δ 2.53) and dd (δ 2.74) showed correlations with methylene carbon at δ 35.8 (C-1). While, methine C-2 (δ 31.6) also showed HSQC interaction with multiplet at δ 2.53, which indicates that it is also an overlap signal between H-1 and H-2.
Table 2 NMR Chemical shift data of compound 3b
C# |
δC |
δH |
Mult. |
HBMC |
COSY |
NOESY |
Axial proton. Equatorial proton. |
4b |
29.3 |
2.38–2.29 (m) |
CH2 |
C-3, C-8a, C-9a, C-12, C-13 |
— |
— |
4a |
— |
1.27 (td) |
CH2 |
C-4a |
— |
— |
2 |
31.6 |
2.57–2.50 (m + d) |
CH |
C-3, C-4a, C-9a, C-10 |
H-3a, H-3b, H-4b |
H-1a |
3b |
26.3 |
1.40–1.35 (dm) |
CH2 |
C-12 |
H-3a, H-4b |
H-2, H-3a, H-11 |
3a |
— |
2.02 (tt) |
CH2 |
C-1, C-2, C-4a, C-10 |
H-4b |
H-1a, H-2, H-4b |
1b |
35.8 |
2.57–2.50 (m + d) |
CH2 |
C-3, C-4a, C-9a, C-10 |
— |
H-2, H-4b |
1a |
— |
2.74 (dd) |
CH2 |
C-2, C-6, C-9a, C-10 |
— |
— |
4a |
62.1 |
— |
C |
— |
— |
— |
4b |
118.0 |
— |
C |
— |
— |
— |
5 |
126.4 |
7.44–7.42 (m) |
CH |
C-4a, C-4b, C-5, C-6, C-7, C-8a, C-8 |
— |
— |
6 |
154.5 |
— |
C |
— |
— |
— |
7 |
130.5 |
7.44–7.42 (m) |
CH |
C-4a, C-4b, C-5, C-6, C-7, C-8a, C-8 |
— |
— |
8 |
121.4 |
7.44–7.42 (m) |
CH |
C-4a, C-4b, C-5, C-6, C-7, C-8a, C-8 |
— |
— |
8a |
145.2 |
— |
C |
— |
— |
— |
9a |
189.2 |
— |
C |
— |
|
|
10 |
18.1 |
0.81 (d) |
CH3 |
C-1, C-2, C-3 |
H-2 |
H-2, H-3b, H-4a |
11 |
28.6 |
2.38–2.29 (m) |
CH |
C-4a, C-8a, C-9a, C-12, C-13 |
H-12, H-13 |
H-1a |
12 |
17.1 |
1.20 (d) |
CH3 |
C-4a, C-11, C-13 |
H-11 |
— |
13 |
16.5 |
0.26 (d) |
CH3 |
C-4a, C-11, C-12 |
H-11 |
H-1a, H-11, H-12 |
The key difference between compounds 2j and 3b is the presence of isolated methyl protons at high field which indicates that C-10 is also shielded by C
N, which is only possible by placing it in axial position. Furthermore, assignment of methyl (C-10) was carried out on the basis of HMBC correlations with C-1 (δ 35.8), C-2 (δ 31.6), and C-3 (δ 26.8). Position of C-1 was deduced from the HMBC correlations of 2,3,4,4a-tetrahydro-1H-carbazole carbon (δ 189.2) with methylene protons, H-1ax and H-1eq. This assignment was also confirmed by HMBC correlations of H-1ax (δ 2.74) with C-2 (δ 31.6) and C-10 (δ 18.1). Assignment of C-3 was made by HMBC correlations of methylene protons (H-3eq) with methyl (C-12) and HMBC correlations of H-3ax with C-4a and C-10. This was also confirmed by its COSY correlation with vicinal protons, H-4ax and H-4eq. A methylene proton (H-4ax) showed COSY correlations with H-3ax and H-3eq. The stereochemistry at various centers was deduced by NOESY experiments. Methyl H-10 showed interaction with protons at δ 1.26 (H-4ax) and at δ 2.53 (H-2) which indicated that these protons are syn to H-10 and are axially oriented. In addition, methyl H-10 showed NOE interaction with δ 1.47 (H-3), indicating that this H-3 has equatorial position. The NOESY correlations between methyl protons (H-13) and one of the methylene protons, H-1 (δ 2.74), showed that this proton is syn to H-13 hence axially oriented.
2.4. In vitro AChE and BChE inhibitory activity
2,3,4,4a-Tetrahydro-1H-carbazole compounds syn-2(a–q) and anti-3(a–b) were evaluated for AChE and BChE inhibition according to a modified ELISA method,23 using galantamine as standard (Table 3). The results revealed that most of the synthesized compounds exhibited a moderate to low inhibitory activities. In case of AChE inhibitory activity, unsubstituted analogue 2a was found to be most active in the series, with IC50 value 9.7 ± 0.2 μM. In comparison, compound 2c exhibited a moderate inhibition, while compounds 2d and 2(p–q) were found to be weakly active. Rest of the compounds 2b, 2(e–o) and 3(a–b) displayed less than 50% inhibition and were considered inactive. Structure activity relationship (SAR) study suggested that substituents at aromatic ring may influence the bioactivities of the compounds. The compounds with hydrogen bonding ability such as, fluoro, cyano and methoxy substituents showed a moderate to weak activity. Comparison of unsubstituted isomeric 2,3,4,4a-tetrahydro-1H-carbazoles 2a and 3a revealed that only syn-2,3,4,4a-tetrahydro-1H-carbazole 2a was found to be active against AChE inhibitory activity.
Table 3 Cholinesterases (AChE and BChE) inhibitory activities of 2,3,4,4a-tetrahydro-1H-carbazole syn-2(a–q) and anti-3(a–b)
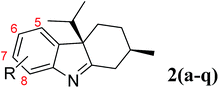
|
Comp no. |
Yield (%) |
IC50 ± SEM (μM) |
Selectivityc |
AChE |
BChE |
Standard inhibitor. Not active. Selectivity ratio = IC50 ratio (BChE/AChE).24 |
2a R = H |
40 |
9.7 ± 0.2 |
18.6 ± 1 |
1.92 |
2b R = 8F |
36 |
NAb |
35.6 ± 1 |
— |
2c R = 7F |
42 |
50 ± 1 |
48.9 ± 0.05 |
0.98 |
2d R = 6F |
40 |
136.2 ± 0.5 |
95.6 ± 1 |
0.70 |
2e R = 8Cl |
30 |
NA |
23.2 ± 1 |
— |
2f R = 37Cl |
42 |
NA |
53.7 ± 0.5 |
— |
2g R = 6Cl |
33 |
NA |
NA |
— |
2h R = 8Br |
35 |
NA |
81.4 ± 1 |
— |
2i R = 7Br |
35 |
NA |
19.9 ± 0.5 |
— |
2j R = 6Br |
45 |
NA |
NA |
— |
2k R = 8Me |
38 |
NA |
19.9 ± 0.5 |
— |
2l R = 7Me |
56 |
NA |
18.4 ± 1 |
— |
2m R = 6Me |
54 |
NA |
125.3 ± 0.0 |
— |
2n R = 6i-Pr |
56 |
NA |
NA |
— |
2o R = 7OMe |
32 |
NA |
37.9 ± 1.4 |
— |
2p R = 6OMe |
36 |
147.2 ± 0.5 |
24.4 ± 1 |
0.17 |
2q R = 6CN |
30 |
102.4 ± 1.7 |
42.2 ± 0.5 |
0.41 |
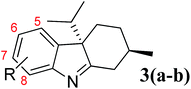 |
3a R = H |
39 |
NA |
24 ± 0.5 |
— |
3b R = 6Br |
35 |
NA |
145 ± 1.1 |
— |
Galantaminea |
|
0.93 ± 0.1 |
4.2 ± 0.3 |
4.52 |
In case of BChE inhibitory activity, seven analogues include unsubstituted 2a, 3a, 2-chloro 2e, 7-bromo 2i, 8-methyl 2k, 7-methyl 2l and 6-methoxy 2p were found to be the most potent in the series, as reflected by their IC50 values. In contrast, compounds 2(b–c), 2f, 2l, 2o and 2q were found to be moderate inhibitors, while rest of the compounds 2d, 2h, 2m and 3b (anti) exhibited a weak inhibitory activity. It was suggested from activity results that substitution at C-6 resulted in a marked decrease in activity, except substitution with fluoro, cyano and methoxy groups. In conclusion, substitution at C-6 can be considered as a site for hydrogen bonding with the active residues of catalytic gorge. Replacement of methyl group from C-6 to C-8 or C-7 positions increased activity up to seven folds. Moreover, comparison between alkyl substituted analogues indicated that exchange of methyl with i-Pr group, as in compound 2n, resulted in loss of activity. For the halogenated compounds, structure activity relationship results revealed that substitution of fluorine and chorine at C-8 position increased the activity, as compared to substitution with bromine. While replacement of bromine from C-8 to C-7 position, resulted in 4-times increase in activity. Comparison among BChE activity of isomeric 2,3,4,4a-tetrahydro-1H-carbazoles showed that both unsubstituted syn-2a and anti-3a 2,3,4,4a-tetrahydro-1H-carbazoles show good and comparable activity. While, 6-bromo substituted isomers 2j and 3b showed insignificant activity.
Because of more aliphatic substitution in BChE, less aromatic residues are present in it, which make catalytic gorge of BChE much wider than that of AChE. This aliphatic lining in catalytic site gorge of BChE is responsible for its selectivity against different inhibitors.4 Hence, due to large size of the title compound, they were anticipated to be more active in BChE inhibition than AChE. In this context, most active syn-2,3,4,4a-tetrahydro-1H-carbazoles 2e, 2i, 2k, 2l, 2p and anti-2,3,4,4a-tetrahydro-1H-carbazole 3a exhibited selectivity for BChE inhibition. However, the unsubstituted syn-2,3,4,4a-tetrahydro-1H-carbazole 2a was found to be active against both enzymes and displayed a weak selectivity ratio.
Furthermore, synthetic 2,3,4,4a-tetrahydro-1H-carbazoles were also tested against various enzyme inhibitory activities, such as xanthine oxidase, α-chymotrypsin, urease, phosphodiesterase and carbonic anhydrase-II activity, as well as against DPPH radical scavenging activities but none of the synthesized compounds were found to be active. Compound 2h and 2n showed weak inhibition with IC50 value of 21.3 ± 0.3 and 21.1 ± 0.2 μM respectively, while rest of the compounds displayed % inhibition less than 30, therefore considered as non-toxic Some synthesized 2,3,4,4a-tetrahydro-1H-carbazoles displayed selectivity inhibition against BChE and can serve as target for healing of neurodegeneration.
Type of inhibition plays an important role in identifying site of inhibitor binding with enzyme. The classical competitive inhibitors bind to active site of free enzyme, while non-competitive inhibitors bind at allosteric sites of free enzyme. In contrast, uncompetitive inhibitors do not bind with free enzyme but to enzyme–substrate complex, leaving complex inactive. On the other hand, combination of above given types results in mixed type inhibitors. They have ability to bind with free enzyme as well as enzyme–substrate complex.
Enzyme kinetic studies against cholinesterases inhibition were carried out with active 2,3,4,4a-tetrahydro-1H-carbazoles. Type of inhibition and other parameters including Ki, K′i Vmax, and Km were obtained from Lineweaver–Burk and Dixon plots, as presented in Table 4. Kinetic studies of AChE inhibitors identified compounds 2a (R = H), 2c (R = 7F), 2d (R = 6F), 2p (R = 6OMe), and 2q (R = 6CN) as mixed type inhibitors. Owing to mixed type inhibitory potency, compounds 2a, 2c, 2d, 2p, and 2q can bind with enzyme whether it is attached with substrate or not. As K′i > Ki, these inhibitors tend to bind more with free enzyme, rather than binding with enzyme–substrate complex. Thereby, compound 2a, 2c, 2d, 2p, and 2q behave more like competitive inhibitors. In addition, comparing Ki values showed that compound 2a (R = H) (Ki = 0.03 ± 0.04 mM), 2c (R = 7F) (Ki = 0.03 ± 0.01 mM) and 2p (R = 6OMe) (Ki = 0.03 ± 0.01 mM) possess strong binding affinity with the target enzyme than other compounds 2d, and 2q (Table 4).
Table 4 Kinetic parameters from steady state inhibition data of AChE and BChE
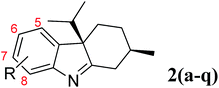
|
Comp no. |
Ki ± SEM (mM) |
K′i (mM) |
Inhibition |
Mixed type inhibitor. |
AChE |
2a R = H |
0.03 ± 0.04 |
0.07 |
MTa |
2c R = 7F |
0.03 ± 0.01 |
0.04 |
MTa |
2d R = 6F |
0.05 ± 0.07 |
0.14 |
MTa |
2p R = 6OMe |
0.03 ± 0.01 |
0.06 |
MTa |
2q R = 6CN |
0.04 ± 0.02 |
0.07 |
MTa |
![[thin space (1/6-em)]](https://www.rsc.org/images/entities/char_2009.gif) |
BChE |
2a R = H |
0.05 ± 0.01 |
0.04 |
MTa |
2b R = 8F |
0.03 ± 0.002 |
0.03 |
MTa |
2c R = 7F |
0.04 ± 0.03 |
0.07 |
MTa |
2d R = 6F |
0.11 ± 0.07 |
0.19 |
MTa |
2e R = 8Cl |
0.04 ± 0.01 |
0.03 |
MTa |
2i R = 7Br |
0.07 ± 0.03 |
0.10 |
MTa |
2k R = 8Me |
0.03 ± 0.01 |
0.05 |
MTa |
2l R = 7Me |
0.10 ± 0.13 |
0.09 |
MTa |
2p R = 6OMe |
0.11 ± 0.01 |
0.12 |
MTa |
2q R = 6CN |
0.21 ± 0.14 |
0.38 |
MTa |
In case of BChE inhibition, kinetic studies revealed compounds 2a, 2b, 2c, 2d, 2e, 2i, 2k, 2l, 2p, and 2q as mixed type inhibitors with Ki values ranging between 0.03 ± 0.002 to 0.21 ± 0.14 mM. Compounds 2i (0.10 mM), 2k (0.05 mM), and 2p (0.12 mM) have K′i value slightly greater than Ki values of 2i (0.07 ± 0.03 mM), 2k (0.03 ± 0.01 mM), and 2p (0.10 ± 0.13 mM), respectively, therefore they inhibit more through competitive mode of inhibition. In case of compound 2a, 2e and 2l, K′i values (0.04 and 0.03 mM) have slightly variation in Ki values (0.05 ± 0.01 and 0.04 ± 0.01 mM), respectively, hence they tend to bind with enzyme–substrate complex. Overall, Ki values for BChE revealed that compound 2b (Ki = 0.03 ± 0.002 mM) and 2k (0.03 ± 0.01 mM) possessed highest binding affinity while compound 2q (Ki = 0.21 ± 0.14 mM) possess ∼10 fold weaker binding capacity. Unsubstituted 2,3,4,4a-tetrahydro-1H-carbazole 2a (Ki = 0.05 ± 0.01 mM) showed binding affinity ∼2 times weaker than compound 2b and 2k for BChE. In contrast, unsubstituted 2,3,4,4a-tetrahydro-1H-carbazole 2a (Ki = 0.03 ± 0.04 mM) displayed binding affinity twice better for AChE than BChE (see ESI† for kinetic graphs).
3. Conclusion
In conclusion, the Fischer indole reaction between L-menthone and substituted phenyl hydrazines results in regioselective 2,3,4,4a-tetrahydro-1H-carbazoles (syn-2(a–q) and anti-3(a–b)) formation, instead of the typical indole product. The diastereoisomeric ratio between syn-2 and anti-3 2,3,4,4a-tetrahydro-1H-carbazole was found to range from 1
:
1 to 1.8
:
1, as determined from 1H NMR spectra, The pure compounds 2(a–b) and 3(a–b) were evaluated for various bioactivities. The synthetic 2,3,4,4a-tetrahydro-1H-carbazoles were found active only against cholinesterases, AChE and BChE. The activity of 2,3,4,4a-tetrahydro-1H-carbazoles provides a new scaffold for cholinesterase inhibition. Further modifications of active 2,3,4,4a-tetrahydro-1H-carbazoles can potentially lead to suitable drug candidate for AD therapy.
4. Experimental
4.1. Material and methods
L-Menthone (96%) and phenyl hydrazine hydrochlorides were purchased from Aldrich and Alfa Aesar and were used without further purification. Thin-layer chromatography (TLC) was developed on aluminum-supported pre-coated silica gel aluminum plates (Kieselgel 60, 254, E. Merck, Germany). NMR Spectra were recorded on Avance Bruker 300 and 400 MHz in CDCl3. EI-MS Spectra were recorded on Finnigan MAT-312, Germany, and JEOL MSRoute JMS600H, Japan instruments. IR Spectra was obtained by using Bruker-Vector instrument. UV Spectra was recorded on Hitachi U-3200 ND Thermoscientific Evolution 310 UV-Vis spectrophotometer. All the cholinesterase reactions were performed in triplicate in a 96-well micro plate on SpectraMax340 (Molecular Devices, CA USA). Electric eel acetylcholinesterase (EC 3.1.1.7), Equine serum butyrylcholinesterase (EC 3.1.1.8), acetylcholine iodide, butyrylcholine chloride and 5,5′-dithio-bis (2-nitrobenzoic) acid were purchased from Sigma (St. Louis., Mo, USA). Analytical grades buffers were used. The Bruker Smart APEX II single-crystal X-ray diffractometer, fitted with CCD detector, was used to collect the data. Data was reduced and solved by using SAINT program and direct methods, respectively.25,26 Finally the structures were solved by program SHELXL97 (Sheldrick 1997) by using full-matrix least-square calculation on F2.27,28 The figures were plotted by using ORTEP program.29
4.2. General procedure for the synthesis of 2,3,4,4a-tetrahydro-1H-carbazoles of L-menthone
To a solution of L-menthone (1 g, 6.5 mmol) in acetic acid (5 mL), a stochiometeric amount of phenyl hydrazine hydrochlorides (6.8 mmol) was added and refluxed under nitrogen atmosphere for 30 min. Progress of reaction was monitored via TLC. On completion, reaction mixture was diluted with distilled water and extracted with ethyl acetate (20 mL × 3). The combined organic layers was washed with 5% aqueous NaOH (10 mL × 2) and brine (10 mL × 2), then dried using anhydrous sodium sulphate and concentrated in vacuo. Crude product was purified by column chromatography over silica gel using n-hexanes and ethyl acetate (9.9
:
0.1 → 9.0
:
1.0) as eluent.
(2R,4aR)-4a-Isopropyl-2-methyl-2,3,4,4a-tetrahydro-1H-carbazole (2a). Yield = 40%; white solid; Rf = 0.26 (EtOAc/hex 2
:
8); IR (CH2Cl2): νmax/cm−1: 2960, 2927, 2855, 1619 (C
N), 1468; UV (MeOH) nm: 250, 219; 1H NMR: (300 MHz, CDCl3): δ 7.55 (d, J8,7 = 7.8 Hz, 1H, H-8), 7.29 (m, 2H, H-5/H-7), 7.12 (td, J6,(5,7) = 7.5 Hz, J6,8 = 0.6 Hz, 1H, H-6), 2.82 (ddd, J1-eq,1-ax = 12.9 Hz, J1-eq,2-ax = 4.2 Hz, J1-eq,3-eq = 1.5 Hz, 1H, 1-eq CH2), 2.54 (dt, J4-eq,4-ax = 13.8 Hz, J15-eq,(3-ax,3-eq) = 2.7 Hz, 1H, 4-eq CH2), 2.43 (sep, J 11,(12,13) = 6.9 Hz, 1H, 11-CH), 2.20 (t, J1-ax,(1-eq,2-ax) = 12.3 Hz, 1H, 1-ax CH2), 1.57 (m + qd, 3H, 2-ax CH/3-eq CH2/3-ax CH2), 1.21 (d, J12,11 = 6.9 Hz, 3H, 12-CH3), 1.12 (d, J10,2-ax = 6.3 Hz, 3H, 10-CH3), 1.00 (td, J4-ax,(4-eq,3-ax) = 13.4 Hz, J4-ax,3-eq = 4.5 Hz, 1H, 4-ax CH2), 0.25 (d, J13,11 = 6.6 Hz, 3H, 13-CH3); 13C NMR: (300 MHz, CDCl3): δ 190.1 (C), 155.6 (C), 142.6 (C), 127.3 (CH), 124.0 (CH), 123.1 (CH), 120.0 (CH), 60.8 (C), 38.0 (CH2), 36.7 (CH), 33.6 (CH2), 29.2 (CH2), 28.6 (CH), 22.1 (CH3), 16.9 (CH3), 16.3 (CH3); EI-MS: m/z (rel. abund.%): 227 (M+), 212, 185, 143; HR-EIMS: calcd for C16H21N (M): m/z 227.1674, found: 227.1674.
(2R,4aS)-4a-Isopropyl-2-methyl-2,3,4,4a-tetrahydro-1H-carbazole (3a). Yield = 39%; white liquid; Rf = 0.38 (EtOAc/hex 2
:
8); IR (CH2Cl2): νmax cm−1: 2963, 2934, 2875, 1714, 1617 (C
N), 1585, 1466; UV (MeOH) nm: 260, 213; 1H NMR: (300 MHz, CDCl3): δ 7.58 (d, J8,7 = 7.5 Hz, 1H, H-8), 7.29 (m, 2H, H-5/H-7), 7.10 (t, J6,(7,5) = 6.9 Hz, 1H, H-6), 2.75 (dd, J1-ax,1-eq = 13.1 Hz, J1-ax,2-eq = 6.3 Hz, 1H, 1-ax CH2), 2.54 (m + dt, 2H, 1-eq CH2/2-eq CH), 2.34 (m, 2H, 4-eq CH2/11-CH), 2.02 (tt, J3-ax,(3-eq,4-ax) = 14.1 Hz, J3-ax,(2-eq,4-eq) = 4.2 Hz, 1H, 3-ax CH2), 1.35 (dm, 1H, 3-eq CH2), 1.26 (td, J4-ax,(3-ax,4-eq) = 14.1 Hz, J4-ax,3-eq = 3.9 Hz, 1H, 4-ax CH2), 1.20 (d, J12,11 = 6.9 Hz, 3H, 12-CH3), 0.81 (d, J10,2-ax = 7.2 Hz, 3H, 10-CH3), 0.23 (d, J13,11 = 6.6 Hz, 3H, 13-CH3); 13C NMR: (300 MHz, CDCl3): δ 190.4 (C), 155.5 (C), 142.7 (C), 127.3 (CH), 124.0 (CH), 122.9 (CH), 119.9 (CH), 61.5 (C), 43.1 (CH2), 37.0 (CH2), 29.3 (CH), 29.1 (CH2), 26.7 (CH), 21.1 (CH3), 16.9 (CH3), 16.4 (CH3); EI-MS: m/z (rel. abund.%): 227 (M+), 212, 185, 143; HR-EIMS: calcd for C16H21N (M): m/z 227.1674, found: 227.1677.
(2R,4aR)-8-Fluoro-4a-isopropyl-2-methyl-2,3,4,4a-tetrahydro-1H-carbazole (2b). Yield = 36%; yellow liquid; Rf = 0.40 (EtOAc/hex 2
:
8); IR (CH2Cl2): νmax cm−1: 2959, 2928, 2869, 1583 (C
N), 1480, 1457; UV (MeOH) nm: 253, 221; 1H NMR: (300 MHz, CDCl3): δ 7.05 (m, 3H, H-5/H-6/H-7), 2.88 (ddd, J1-eq,1-ax = 12.8 Hz, J1-eq,2-ax = 4.2 Hz, J1-eq,3-eq = 0.9 Hz, 1H, 1-eq CH2), 2.53 (dt, J4-eq,4-ax = 13.8 Hz, J4-eq,(3-ax,3-eq) = 2.7 Hz, 1H, 4-eq CH2), 2.33 (sep, J11,(12,13) = 6.6 Hz, 1H, 11-CH), 2.19 (t, J1-ax,(1-eq,2-ax) = 12.6 Hz, 1H, 1-ax CH2), 1.60 (m + qd, 3H, 2-ax CH/3-eq CH2/3-ax CH2), 1.20 (d, J12,11 = 6.9 Hz, 3H, 12-CH3), 1.12 (d, J10,2-ax = 6.3 Hz, 3H, 10-CH3), 0.98 (td, J4-ax,(4-eq,3-ax) = 13.4 Hz, J4-ax,3-eq = 4.5 Hz, 1H, 4-ax CH2), 0.27 (d, J13,11 = 6.6 Hz, 3H, 13-CH3); 13C NMR: (300 MHz, CDCl3): δ 190.9 (C), 155.9 (C), 152.6 (C), 146.4 (C), 125.4 (CH), 119.0 (CH), 114.6 (CH), 61.8 (C), 38.2 (CH2), 36.9 (CH), 33.8 (CH2), 29.2 (CH2), 28.8 (CH), 22.1 (CH3), 16.9 (CH3), 16.4 (CH3); EI-MS: m/z (rel. abund.%): 245 (M+), 230, 203, 161; HR-EIMS: calcd for C16H20NF (M): m/z 245.1580, found: 245.1578.
(2R,4aR)-7-Fluoro-4a-isopropyl-2-methyl-2,3,4,4a-tetrahydro-1H-carbazole (2c). Yield = 42%; yellow liquid; Rf = 0.35 (EtOAc/hex 2
:
8); IR (CH2Cl2): νmax cm−1: 2962, 2930, 2874, 1620, 1582 (C
N), 1472; UV (MeOH) nm: 251, 223; 1H NMR: (300 MHz, CDCl3): δ 7.23 (m, 2H, H-6/H-8), 6.83 (m, 1H, H-5), 2.78 (ddd, J1-eq,1-ax = 12.9 Hz, J1-eq,2-ax = 4.2 Hz, J1-eq,3-eq = 1.5 Hz, 1H, 1-eq CH2), 2.52 (dt, J4-eq,4-ax = 14.1 Hz, J4-eq,(3-ax,3-eq) = 2.7 Hz, 1H, 4-eq CH2), 2.24 (sep + t, 2H, 1-ax CH2/11-CH), 1.57 (m + qd, 3H, 2-ax CH/3-eq CH2/3-ax CH2), 1.18 (d, J12,11 = 6.9 Hz, 3H, 12-CH3), 1.12 (d, J10,2-ax = 6.3 Hz, 3H, 10-CH3), 0.99 (td, J4-ax,(4-eq,3-ax) = 13.5 Hz, J4-ax,3-eq = 4.5 Hz, 1H, 4-ax CH2), 0.25 (d, J13,11 = 6.9 Hz, 3H, 13-CH3); 13C NMR: (300 MHz, CDCl3): δ 192.7 (C), 164.4 (C), 161.1 (C), 138.3 (C), 123.4 (CH), 110.5 (CH), 107.6 (CH), 60.6 (C), 38.2 (CH2), 36.8 (CH), 33.9 (CH2), 29.2 (CH2), 28.8 (CH), 22.1 (CH3), 17.0 (CH3), 16.3 (CH3); EI-MS: m/z (rel. abund.%): 245 (M+), 230, 203, 161; HR-EIMS: calcd for C16H20NF (M): m/z 245.1580, found: 245.1584.
(2R,4aR)-6-Fluoro-4a-isopropyl-2-methyl-2,3,4,4a-tetrahydro-1H-carbazole (2d). Yield = 40%; yellow liquid; Rf = 0.21 (EtOAc/hex 2
:
8); IR (CH2Cl2): νmax cm−1: 2960, 2928, 2871, 1619 (C
N), 1590, 1459, 1265, 1174; UV (MeOH) nm: 272, 261, 248, 223; 1H NMR: (400 MHz, CDCl3): δ 7.23 (m, 2H, H-5/H-7), 6.82 (m, 1H, H-8), 2.80 (ddd, J1-eq,1-ax = 12.8 Hz, J1-eq,2-ax = 2.8 Hz, J1-eq,3-eq = 2.0 Hz, 1H, 1-eq CH2), 2.51 (dt, J4-eq,4-ax = 14.0 Hz, J4-eq,(3-ax,3-eq) = 2.8 Hz, 1H, 4-eq CH2), 2.28 (sep, J11,(12,13) = 6.8 Hz, 1H, 11-CH), 2.19 (t, J1-ax,(1-eq,2-ax) = 12.4 Hz, 1H, 1-ax CH2), 1.62 (m, 2H, 2-ax CH/3-eq CH2), 1.48 (qd, J3-ax,(2-ax,3-eq,4-ax) = 13.6 Hz, J3-ax,4-eq = 3.6 Hz, 1H, 3-ax CH2), 1.18 (d, J12,11 = 6.8 Hz, 3H, 12-CH3), 1.12 (d, J10,2-ax = 6.4 Hz, 3H, 10-CH3), 0.98 (td, J4-ax,(4-eq,3-ax) = 13.6 Hz, J4-ax,3-eq = 4.0 Hz, 1H, 4-ax CH2), 0.25 (d, J13,10 = 6.8 Hz, 3H, 13-CH3);13C NMR: (400 MHz, CDCl3): δ 190.1 (C), 161.6 (C), 151.7 (C), 144.5 (C), 120.4 (CH), 113.8 (CH), 110.8 (CH), 61.5 (C), 38.1 (CH2), 36.8 (CH), 33.6 (CH2), 29.2 (CH2), 28.6 (CH), 22.1 (CH3), 16.8 (CH3), 16.4 (CH3); EI-MS: m/z (rel. abund.%): 245 (M+), 230, 203, 161; HR-EIMS: calcd for C16H20NF (M): m/z 245.1580, found: 245.1586.
(2R,4aR)-8-Chloro-4a-isopropyl-2-methyl-2,3,4,4a-tetrahydro-1H-carbazole (2e). Yield = 30%; yellow solid; Rf = 0.62 (EtOAc/hex 2
:
8); IR (CH2Cl2): νmax cm−1: 2960, 2929, 2363, 2337, 1719, 1608, 1580 (C
N), 1459; UV (MeOH) nm: 259, 217; 1H NMR: (400 MHz, CDCl3): δ 7.29 (d, J7,6 = 7.8 Hz, 1H, H-7), 7.20 (d, J5,6 = 7.2 Hz, 1H, H-5), 7.05 (t, J6,(7,5) = 7.5 Hz, 1H, H-6), 2.97 (ddd, 1H, J1-eq,1-ax = 12.6 Hz, J1-eq,2-ax = 2.7 Hz, J1-eq,3-eq = 0.8 Hz, 1-eq CH2), 2.52 (dt, J4-eq,4-ax = 13.8 Hz, J4-eq,(3-ax,3-eq) = 3.0 Hz, 1H, 4-eq CH2), 2.30 (sep, J11,(12,13) = 6.9 Hz, 1H, 11-CH), 2.19 (t, J1-ax,(1-eq,2-ax) = 12.6 Hz, 1H, 1-ax CH2), 1.57 (m + qd, 3H, 2-ax CH/3-eq CH2/3-ax CH2), 1.18 (d, J12,11 = 6.9 Hz, 3H, 12-CH3), 1.11 (d, J10,2-ax = 6.3 Hz, 3H, 10-CH3), 1.00 (td, J4-ax,(4-eq,3-ax) = 13.5 Hz, J4-ax,3-eq = 4.2 Hz, 1H, 4-ax CH2), 0.26 (d, J13,11 = 6.9 Hz, 3H, 13-CH3); 13C NMR: (300 MHz, CDCl3): 192.3 (C), 151.7 (C), 144.7 (C), 128.0 (CH), 125.3 (CH), 125.1 (C), 121.6 (CH), 62.4 (C), 38.0 (CH2), 37.0 (CH), 33.9 (CH2), 29.1 (CH2), 28.9 (CH), 22.1 (CH3), 16.9 (CH3), 16.4 (CH3); EI-MS: m/z (rel. abund.%): 261 (M)+, 263 (M + 2)+, 246, 219, 177; HR-EIMS: calcd for C16H20NCl (M): m/z 261.1284, found: 261.1296.
(2R,4aR)-7-Chloro-4a-isopropyl-2-methyl-2,3,4,4a-tetrahydro-1H-carbazole (2f). Yield = 42%; yellow liquid; Rf = 0.40 (EtOAc/hex 2
:
8); IR (CH2Cl2): νmax cm−1: 2960, 2928, 2871, 1580 (C
N), 1455; UV (MeOH) nm 222; 1H NMR: (300 MHz, CDCl3): δ 7.53 (d, J8,6 = 1.8 Hz, 1H, H-8), 7.22 (d, J5,6 = 8.1 Hz, 1H, H-5), 7.11 (dd, J6,5 = 8.1 Hz, J6,8 = 1.8 Hz, 1H, H-6), 2.81 (ddd, J1-eq,1-ax = 12.9 Hz, J1-eq,2-ax = 4.1 Hz, J1-eq,3-eq = 1.2 Hz, 1H, 1-eq CH2), 2.52 (dt, J4-eq,4-ax = 13.8 Hz, J4-eq,(3-ax,3-eq) = 3.0 Hz, 1H, 4-eq CH2), 2.28 (sep, J11,(12,13) = 6.9 Hz, 1H, 11-CH), 2.20 (t, J1-ax,(1-eq,2-ax) = 12.6 Hz, 1H, 1-ax CH2), 1.60 (m + qd, 3H, 2-ax CH/3-eq CH2/3-ax CH2), 1.19 (d, J12,11 = 6.9 Hz, 3H, 12-CH3), 1.12 (d, J10,2-ax = 6.3 Hz, 3H, 10-CH3), 0.97 (td, J4-ax,(4-eq,3-ax) = 13.7 Hz, J4-ax,3-eq = 4.2 Hz, 1H, 4-ax CH2), 0.26 (d, J13,11 = 6.9 Hz, 3H, 13-CH3);13C NMR: (300 MHz, CDCl3): δ 192.3 (C), 157.0 (C), 141.2 (C), 133.1 (C), 124.1 (CH), 123.8 (CH), 120.6 (CH), 60.9 (C), 38.2 (CH2), 36.9 (CH), 33.7 (CH2), 29.2 (CH2), 28.8 (CH), 22.2 (CH3), 17.4 (CH3), 17.0 (CH3); EI-MS: m/z (rel. abund.%): 261 (M)+, 263 (M + 2)+, 246, 219, 177; HR-EIMS: calcd for C16H20NCl (M): m/z 261.1284, found: 261.1287.
(2R,4aR)-6-Chloro-4a-isopropyl-2-methyl-2,3,4,4a-tetrahydro-1H-carbazole (2g). Yield = 33%; white solid; Rf = 0.25 (EtOAc/hex 2
:
8); IR (CH2Cl2): νmax cm−1: 2959, 2927, 2871, 1582 (C
N), 1448; UV (MeOH) nm: 272, 261, 247, 230; 1H NMR: (400 MHz, CDCl3): δ 7.44 (d, J8,7 = 7.6 Hz, 1H, H-8), 7.47 (m, 2H, H-5/H-7), 2.79 (ddd, J1-eq,1-ax = 12.8 Hz, J1-eq,2-ax = 2.8 Hz, J1-eq,3-eq = 1.2 Hz, 1H, 1-eq CH2), 2.49 (dt, J4-eq,4-ax = 13.6 Hz, J4-eq,(3-ax,3-eq) = 1.2 Hz, 1H, 4-eq CH2), 2.28 (sep, J11,(12,13) = 6.8 Hz, 1H, 11-CH), 2.17 (t, J1-ax,(1-eq,2-ax) = 12.4 Hz, 1H, 1-ax CH2), 1.63 (m, 2H, 2-ax CH/3-eq CH2), 1.49 (qd, J3-ax,(2-ax,3-eq,4-ax) = 13.6 Hz, J3-ax,4-eq = 3.2 Hz, 1H, 3-ax CH2), 1.18 (d, J12,11 = 6.8 Hz, 3H, 12-CH3), 1.10 (d, J10,2-ax = 6.4 Hz, 3H, 10-CH3), 0.99 (td, J4-ax,(4-eq,3-ax) = 13.6 Hz, J4-ax,3-eq = 4.0 Hz, 1H, 4-ax CH2), 0.26 (d, J13,11 = 6.8 Hz, 3H, 13-CH3);13C NMR: (400 MHz, CDCl3): δ 190.9 (C), 154.3 (C), 144.6 (C), 130.1 (C), 127.7 (CH), 123.7 (CH), 120.9 (CH), 61.6 (C), 38.1 (CH2), 36.8 (CH), 33.7 (CH2), 29.2 (CH2), 28.8 (CH2), 22.1 (CH3), 17.0 (CH3), 16.4 (CH3); EI-MS: m/z (rel. abund.%): 261 (M)+, 263 (M+2)+, 246, 219, 177; HR-EIMS: calcd for C16H20NCl (M): m/z 261.1284, found: 261.1277.
(2R,4aR)-8-Bromo-4a-isopropyl-2-methyl-2,3,4,4a-tetrahydro-1H-carbazole (2h). Yield = 35%; brown liquid; Rf = 0.49 (EtOAc/hex 2
:
8); IR (CH2Cl2): νmax cm−1: 2959, 2927, 2871, 1578 (C
N), 1457, 1416, 758; UV (MeOH) nm: 273, 268, 261, 252, 244, 228; 1H NMR: (300 MHz, CDCl3): δ 7.43 (d, J7,6 = 7.2 Hz, 1H, H-7), 7.25 (d, J5,6 = 6.9 Hz, 1H, H-5), 6.97 (t, J6,(7,5) = 7.8 Hz, 1H, H-6), 2.93 (ddd, J1-eq,1-ax = 12.9 Hz, J1-eq,2-ax = 3.0 Hz, J1-eq,3-eq = 1.6 Hz, 1H, 1-eq CH2), 2.49 (dt, J4-eq,4-ax = 13.8 Hz, J4-eq,(3-ax,3-eq) = 3.0 Hz, 1H, 4-eq CH2), 2.28 (sep, J11,(12,13) = 6.9 Hz, 1H, 11-CH), 2.16 (t, J1-ax,(1-eq,2-ax) = 12.6 Hz, 1H, 1-ax CH2), 1.55 (m + qd, 3H, 2-ax CH/3-eq CH2/3-ax CH2), 1.17 (d, J12,11 = 6.9 Hz, 3H, 12-CH3), 1.09 (d, J10,2-ax = 6.6 Hz, 3H, 10-CH3), 0.99 (td, J4-ax,(5-eq,3-ax) = 13.5 Hz, J4-ax,3-eq = 4.5 Hz, 1H, 4-ax CH2), 0.25 (d, J13,11 = 6.6 Hz, 3H, 13-CH3); 13C NMR: (400 MHz, CDCl3): δ 192.0 (C), 153.7 (C), 144.6 (C), 130.9 (CH), 125.5 (CH), 122.1 (CH), 114.1 (C), 62.7 (C), 38.1 (CH2), 36.8 (CH), 33.8 (CH2), 29.1 (CH2), 28.9 (CH), 22.0 (CH3), 16.9 (CH3), 16.4 (CH3); EI-MS: m/z (rel. abund.%): 305 (M)+, 307 (M + 2)+, 290, 263, 221; HR-EIMS: calcd for C16H20NBr (M): m/z 305.0779, found: 305.0763.
(2R,4aR)-7-Bromo-4a-isopropyl-2-methyl-2,3,4,4a-tetrahydro-1H-carbazole (2i). Yield = 35%; yellow liquid; Rf = 0.41 (EtOAc/hex 2
:
8); IR (CH2Cl2): νmax cm−1: 2959, 2927, 2870, 1578 (C
N), 1452; UV (MeOH) nm: 260, 253, 236, 230; 1H NMR: (300 MHz, CDCl3): δ 7.69 (d, J8,6 = 1.5 Hz, 1H, H-8), 7.26 (dd, J6,5 = 7.8 Hz, J6,8 = 1.8 Hz, 1H, H-6), 7.17 (d, J5,6 = 7.8 Hz, 1H, H-5), 2.81 (ddd, J1-eq,1-ax = 13.1 Hz, J1-eq,2-ax = 4.2 Hz, J1-eq,3-eq = 1.5 Hz, 1H, 1-eq CH2), 2.51 (dt, J4-eq,4-ax = 14.1 Hz, J4-eq,(3-ax,3-eq) = 2.7 Hz, 1H, 4-eq CH2), 2.29 (sep, J11,(12,13) = 6.9 Hz, 1H, 11-CH), 2.20 (t, J1-ax,(1-eq,2-ax) = 12.6 Hz, 1H, 1-ax CH2), 1.56 (m + qd, 3H, 2-ax CH/3-eq CH2/3-ax CH2), 1.18 (d, J12,11 = 6.9 Hz, 3H, 12-CH3), 1.12 (d, J10,2-ax = 6.3 Hz, 3H, 10-CH3), 0.98 (td, J4-ax,(4-eq,3-ax) = 13.4 Hz, J4-ax,3-eq = 4.2 Hz, 1H, 4-ax CH2), 0.26 (d, J13,11 = 6.9 Hz, 3H, 13-CH3); 13C NMR: (300 MHz, CDCl3): δ 192.2 (C), 157.3 (C), 141.8 (C), 127.0 (CH), 124.3 (CH), 123.5 (CH), 121.0 (C), 61.0 (C), 38.2 (CH2), 36.8 (CH), 33.7 (CH2), 29.2 (CH2), 28.7 (CH), 22.1 (CH3), 17.0 (CH3), 16.4 (CH3); EI-MS: m/z (rel. abund.%): 305 (M)+, 307 (M + 2)+, 290, 263, 221; HR-EIMS: calcd for C16H20NBr (M): m/z 305.0779, found: 305.0769.
(2R,4aR)-6-Bromo-4a-isopropyl-2-methyl-2,3,4,4a-tetrahydro-1H-carbazole (2j). Yield = 45%; yellow solid; Rf = 0.25 (EtOAc/hex 2
:
8); IR (CH2Cl2): νmax cm−1: 2960, 2928, 2871, 1718, 1582 (C
N), 1450, 825; UV (MeOH) nm: 273, 266, 261, 248, 242, 226; 1H NMR: (300 MHz, CDCl3): δ 7.41 (m, 3H, H-5/H-7/H-8), 2.80 (ddd, J1-eq,1-ax = 12.9 Hz, J1-eq,2-ax = 4.2 Hz, J 1-eq, 3-eq = 1.5 Hz, 1H, 1-eq CH2), 2.50 (dt, J4-eq,4-ax = 14.1 Hz, J4-eq,(3-ax,3-eq) = 2.7 Hz, 1H, 4-eq CH2), 2.30 (sep, J11,(12,13) = 6.9 Hz, 1H, 11-CH), 2.18 (t, J1-ax,(1-eq,2-ax) = 12.6 Hz, 1H, 1-ax CH2), 1.62 (m, 2H, 2-ax CH/3-eq CH2) 1.51 (qd, J3-ax,(2ax,3-eq,4-ax) = 13.2 Hz, J3-ax,4eq = 3.2 Hz, 1H, 3-ax CH2), 1.20 (d, J12,11 = 6.9 Hz, 3H, 12-CH3), 1.12 (d, J10,2-ax = 6.3 Hz, 3H, 10-CH3), 1.01 (td, J4-ax,(4-eq,3-ax) = 13.5 Hz, J4-ax,3-eq = 3.9 Hz, 1H, 4-ax CH2), 0.28 (d, J13,11 = 6.6 Hz, 3H, 13-CH3); 13C NMR: (300 MHz, CDCl3): 190.7 (C), 154.4 (C), 144.8 (C), 130.3 (C), 126.3 (CH), 121.1 (CH), 118.0 (C), 61.3 (C), 37.8 (CH2), 36.6 (CH), 33.4 (CH2), 28.9 (CH2), 28.5 (CH), 21.9 (CH3), 16.8 (CH3), 16.2 (CH3); EI-MS: m/z (rel. abund.%): 305 (M)+, 307 (M + 2)+, 290, 263, 221; HR-EIMS: calcd for C16H20NBr (M): m/z 305.0769, found: 305.0763; X-ray crystallographic data: Yellow crystals, C16H20BrN, Monoclinic, space group P21, a = 9.5180(12), b = 6.7119(9), c = 11.6243(14), β = 90.850(3)°, V = 742.52(16) Å3, Z = 2, ρcalc = 1.370 mg m−3, F(000) = 316, μ (Mo Kα = 0.71073 Å, crystal dimensions 0.51 × 0.31 × 0.17, 1.75° < θ < 25.5°, 4189 reflections were collected, of which, 2505 reflections were observed (Rint = 0.0195). The R values were: R1 = 0.0302, wR2 = 0.0693 for I > 2σ(I), and R1 = 0.0392, wR2 = 0.0726 for all data; max/min residual electron density: 0.348/−0.162 e Å−3 (CCDC 949401).
(2R,4aS)-6-Bromo-4a-isopropyl-2-methyl-2,3,4,4a-tetrahydro-1H-carbazole (3b). Yield = 35%; gummy yellow solid; Rf = 0.34 (EtOAc/hex 2
:
8); IR (CH2Cl2): νmax cm−1: 2961, 2928, 2856, 1726, 1608, 1584 (C
N), 1445, 801; UV (MeOH) nm: 262, 213; 1H NMR: (300 MHz, CDCl3): δ 7.43 (m, 3H, H-5/H-7/H-8), 2.74 (dd, J1-ax,1-eq = 13.1 Hz, J1-ax,2-eq = 6.0 Hz, 1H, 1-ax CH2), 2.54 (dt + m, 2H, 1-eq CH2/2-ax CH), 2.34 (m, 2H, 4-eq CH2/11-CH), 2.02 (tt, J3-ax,(3-eq,4-ax) = 14.1 Hz, J3-ax,(2-eq,4-eq) = 4.2 Hz, 1H, 3-ax CH2), 1.38 (dm, 1H, 3-eq CH2), 1.27 (td, J4-ax,(3-ax,4-eq) = 14.4 Hz, J4-ax,3-eq = 3.9 Hz, 1H, 4-ax CH2), 1.20 (d, J12,11 = 6.9 Hz, 3H, 12-CH3), 0.81 (d, J10,2-ax = 7.2 Hz, 3H, 10-CH3), 0.26 (d, J13,11 = 6.9 Hz, 3H, 13-CH3); 13C NMR: (300 MHz, CDCl3): δ 189.2 (C), 154.5 (C), 145.2 (C), 130.5 (CH), 126.4 (CH), 121.4 (CH), 118.0 (C), 62.1 (C), 35.8 (CH2), 31.6 (CH), 29.3 (CH2), 28.6 (CH), 26.3 (CH2), 18.1 (CH3), 17.1 (CH3), 16.5 (CH3); EI-MS: m/z (rel. abund.%): 305 (M)+, 307 (M+2)+, 290, 263, 221; HR-EIMS: calcd for C16H20NBr (M): m/z 305.0769, found: 305.0761.
(2R,4aR)-4a-Isopropyl-2,8-dimethyl-2,3,4,4a-tetrahydro-1H-carbazole (2k). Yield = 38%; pale yellow liquid; Rf = 0.33 (EtOAc/hex 2
:
8); IR (CH2Cl2): νmax cm−1: 2960, 2927.1, 2873, 1584 (C
N), 1457; UV (MeOH) nm: 272, 250, 246; 1H NMR: (300 MHz, CDCl3): δ 7.13 (t, J5,6/7,6 = 8.1 Hz, 2H, H-5/H-7), 7.02 (t, J6,(7,5) = 7.5 Hz, 1H, H-6), 2.89–2.83 (ddd, J1-eq,1-ax = 12.9 Hz, J1-eq,2-ax = 4.2 Hz, J1-eq,3-eq = 0.9 Hz, 1H, 1-eq CH2), 2.57 (s, 3H, CH3), 2.51 (dt, J4-eq,4-ax = 13.8 Hz, J4-eq,(3-ax,3-eq) = 3.0 Hz, 1H, 4-eq CH2), 2.29 (sep, J11,(12,13) = 6.6 Hz, 1H, 11-CH), 2.20 (t, J1-ax,(1-eq,2-ax) = 12.6 Hz, 1H, 1-ax CH2), 1.56 (m + qd, 3H, 2-ax CH/3-eq CH2/3-ax CH2), 1.20 (d, J12,11 = 6.9 Hz, 3H, 12-CH3), 1.11 (d, J10,2-ax = 6.3 Hz, 3H, 10-CH3), 0.99 (td, J4-ax,(4-eq,3-ax) = 13.4 Hz, J4-ax,3-eq = 4.5 Hz, 1H, 4-ax CH2), 0.25 (d, J13,11 = 6.9 Hz, 3H, 13-CH3); 13C NMR: (300 MHz, CDCl3): 189.2 (C), 154.1 (C), 142.6 (C), 129.6 (C) 128.8 (CH), 124.0 (CH), 120.6 (C), 60.9 (C), 38.2 (CH2), 37.0 (CH), 33.9 (CH2), 29.3 (CH2), 28.7 (CH), 22.2 (CH3), 17.1 (CH3), 16.9 (CH3), 16.4 (CH3); EI-MS: m/z (rel. abund.%): 241 (M)+, 226, 199, 157; HR-EIMS: calcd for C17H23N (M): m/z 241.1830, found: 241.1830.
(2R,4aR)-4a-Isopropyl-2,7-dimethyl-2,3,4,4a-tetrahydro-1H-carbazole (2l). Yield = 56%; yellow liquid; Rf = 0.30 (EtOAc/hex 2
:
8); IR (CH2Cl2): νmax cm−1: 2960, 2927, 2871, 1720, 1664, 1628, 1588 (C
N), 1462, 1445; UV (MeOH) nm: 263, 216, 196; 1H NMR: (300 MHz, CDCl3): δ 7.40 (s, 1H, H-8), 7.20 (d, J6,5 = 7.5 Hz, 1H, H-6), 6.96 (d, J5,6 = 7.5 Hz, 1H, H-5), 2.88 (dd, J1-eq,1-ax = 12.3 Hz, J1-eq,2-ax = 3.0 Hz, 1H, 1-eq CH2), 2.53 (dt, J4-eq,4-ax = 13.8 Hz, J4-eq,(3-ax,3-eq) = 2.1 Hz, 1H, 4-eq CH2), 2.38 (s, 3H, CH3), 2.29 (sep, J11,(12,13) = 6.9 Hz, 1H, 11-CH), 2.19 (t, J1-ax,(1-eq,2-ax) = 12.3 Hz, 1H, 1-ax CH2), 1.57 (m + qd, 3H, 2-ax CH/3-eq CH2/3-ax CH2), 1.19 (d, J12,11 = 6.9 Hz, 3H, 12-CH3), 1.12 (d, J10,2-ax = 6.3 Hz, 3H, 10-CH3), 0.99 (td, J4-ax,(4-eq,3-ax) = 13.2 Hz, J4-ax,3-eq = 4.2 Hz, 1H, 4-ax CH2), 0.28 (d, J13,11 = 6.9 Hz, 3H, 13-CH3); 13C NMR: (300 MHz, CDCl3): 191.1 (C), 139.5 (C), 137.6 (C), 125.2 (CH), 122.9 (CH), 120.6 (CH), 60.7 (C), 37.9 (CH2), 37.0 (CH), 34.0 (CH2), 29.3 (CH2), 28.8 (CH), 22.2 (CH3), 21.5 (CH3), 17.0 (CH3), 16.4 (CH3); EI-MS: m/z (rel. abund.%): 241 (M)+, 226, 199, 157; HR-EIMS: calcd for C17H23N (M): m/z 241.1830, found: 241.1824.
(2R,4aR)-4a-Isopropyl-2,6-dimethyl-2,3,4,4a-tetrahydro-1H-carbazole (2m). Yield = 54%; pale yellow liquid; Rf = 0.26 (EtOAc/hex 2
:
8); IR (CH2Cl2): νmax cm−1: 2958, 2870, 1585 (C
N), 1460; UV (MeOH) nm: 272, 267, 260, 248, 229; 1H NMR: (300 MHz, CDCl3): δ 7.42 (d, J8,7 = 7.8 Hz, 1H, H-8), 7.10 (d, J7,8 = 10.2 Hz, 2H, H-5/H-7), 2.78 (dd, J1-eq,1-ax = 12.8 Hz, J1-eq,2-ax = 3.0 Hz, 1H, 1-eq CH2), 2.49 (dt, J4-eq,4-ax = 13.8 Hz, J4-eq,(3-ax,3-eq) = 2.7 Hz, 1H, 4-eq CH2), 2.35 (s, 3H, CH3), 2.28 (sep, J11,12 = 6.9 Hz, 1H, 11-CH), 2.17 (t, J1-ax,(1-eq,2-ax) = 12.6 Hz, 1H, 1-ax CH2), 1.56 (m + qd, 3H, 2-ax CH/3-eq CH2/3-ax CH2), 1.19 (d, J12,11 = 6.6 Hz, 3H, 12-CH3), 1.10 (d, J10,2-ax = 6.6 Hz, 3H, 10-CH3), 0.98 (td, J4-ax,(4-eq,3-ax) = 13.5 Hz, J4-ax,3-eq = 4.2 Hz, 1H, 4-ax CH2), 0.26 (d, J13,11 = 6.9 Hz, 3H, 13-CH3); 13C NMR: (300 MHz, CDCl3): 189.1 (C), 153.4 (C), 142.9 (C), 133.6 (C), 127.9 (CH), 124.0 (CH), 119.5 (CH), 60.6 (C), 38.0 (CH2), 36.7 (CH), 33.7 (CH2), 29.3 (CH2), 28.6 (CH), 22.1 (CH3), 21.4 (CH3), 16.9 (CH3), 16.3 (CH3); EI-MS: m/z (rel. abund.%): 241 (M)+, 226, 199, 157; HR-EIMS: calcd for C17H23N (M): m/z 241.1830, found: 241.1829.
(2R,4aR)-4a,6-Diisopropyl-2-methyl-2,3,4,4a-tetrahydro-1H-carbazole (2n). Yield = 56%; yellow liquid; Rf = 0.64 (EtOAc/hex 2
:
8); IR (CH2Cl2): νmax cm−1: 2956, 2928, 2871, 1585 (C
N), 1463; UV (MeOH) nm: 222; 1H NMR: (300 MHz, CDCl3): δ 7.47–7.44 (d, J8,7 = 8.4 Hz, 1H, H-8), 7.15 (m, 2H, H-5/H-7), 2.92 (sep, J17,(18,19) = 6.6 Hz, 1H, 17-CH), 2.79 (ddd, J1-eq,1-ax = 12.8 Hz, J1-eq,2-ax = 3.0 Hz, J1-eq,3-eq = 3.0 Hz, 1H, 1-eq CH2), 2.51 (dt, J4-eq,4-ax = 13.8 Hz, J4-eq,(3-ax,3-eq) = 2.7 Hz, 1H, 4-eq CH2), 2.29 (sep, J11,(12,13) = 6.6 Hz, 1H, 11-CH), 2.18 (t, J1-ax,(1-eq,2-ax) = 12.6 Hz, 1H, 1-ax CH2), 1.56 (m + qd, 3H, 2-ax CH/3-eq CH2/3-ax CH2), 1.22 (d, J18,17/19,17 = 5.7 Hz, 6H, 18-CH3/19-CH3), 1.18 (d, J12,11 = 6.3 Hz, 3H, 12-CH3), 1.11 (d, J10,2-ax = 6.3 Hz, 3H, 10-CH3), 1.00 (td, J4-ax,(4-eq,3-ax) = 13.5 Hz, J4-ax,3-eq = 4.2 Hz, 1H, 4-ax CH2), 0.25 (d, J13,11 = 6.9 Hz, 3H, 13-CH3); 13C NMR: (300 MHz, CDCl3): δ 189.8 (C), 153.0 (C), 145.3 (C), 142.7 (C), 125.5 (CH), 121.5 (CH), 119.5 (CH), 60.8 (C), 38.0 (CH2), 36.9 (CH), 34.1 (CH), 33.8 (CH2), 29.3 (CH2), 28.8 (CH2), 24.4 (CH3), 24.3 (CH3), 22.2 (CH3), 17.1 (CH3), 16.5 (CH3); EI-MS: m/z (rel. abund.%): 269 (M)+, 254, 227, 212; HR-EIMS: calcd for C19H27N (M): m/z 269.2143, found: 269.2155.
(2R,4aR)-4a-Isopropyl-7-methoxy-13-methyl-2,3,4,4a-tetrahydro-1H-carbazole (2o). Yield = 32%; greenish yellow liquid; Rf = 0.17 (EtOAc/hex 2
:
8); IR (CH2Cl2): νmax cm−1: 2957, 2928, 2870, 1588 (C
N), 1484, 1459, 1265, 1087 (C–O); UV (MeOH) nm: 256, 228; 1H NMR: (300 MHz, CDCl3): δ 7.22 (m, 2H, H-6/H-8), 6.70 (d, J5,6 = 8.1 Hz, 1H, H-5), 3.78 (s, 3H, OCH3), 2.80 (dt + ddd, 2H, 1-eq CH2/4-eq CH2), 2.23 (sep + t, 2H, 1-ax CH2/11-CH), 1.64 (m, 2H, 2-ax CH/3-eq CH2), 1.45 (qd, J3-ax,(2-ax,3-eq,4-ax) = 12.8 Hz, J3-ax,4-eq = 3.3 Hz, 1H, 3-ax CH2) 1.23 (d, J12,11 = 6.9 Hz, 3H, 12-CH3) 1.10 (d, J10,2-ax = 6.3 Hz, 3H, 10-CH3), 1.06 (td, J4-ax,(4-eq,3-ax) = 13.7 Hz, J4-ax,3-eq = 3.9 Hz, 1H, 4-ax CH2), 0.29 (d, J13,11 = 6.6 Hz, 3H, 13-CH3); 13C NMR: (300 MHz, CDCl3): δ 191.0 (C), 157.5 (C), 156.2 (C), 129.7 (C), 128.6 (CH), 113.3 (CH), 107.6 (CH), 62.7 (C), 55.0 (OCH3), 38.1 (CH2), 36.8 (CH), 32.0 (CH2), 30.0 (CH), 29.4 (CH2), 22.3 (CH3), 18.4 (CH3), 17.0 (CH3); EI-MS: m/z (rel. abund.%): 257 (M)+, 242, 214, 173; HR-EIMS: calcd for C17H23NO (M): m/z 257.1780, found: 257.1775.
(2R,4aR)-4a-Isopropyl-6-methoxy-2-methyl-2,3,4,4a-tetrahydro-1H-carbazole (2p). Yield = 36%; brownish yellow liquid; Rf = 0.11 (EtOAc/hex 2
:
8); IR (CH2Cl2): νmax cm−1: 2961, 2929, 2872, 1715, 1618, 1592 (C
N), 1474, 1437, 1277 (C–O); UV (MeOH) nm: 273, 212; 1H NMR: (300 MHz, CDCl3): δ 7.45 (d, J8,7 = 8.7 Hz, 1H, H-8), 6.89 (d, J5,7 = 2.4 Hz, 1H, H-5), 6.82 (dd, J7,8 = 8.4 Hz, J7,5 = 2.7 Hz, 1H, H-7), 3.80 (s, 3H, OCH3), 2.87 (ddd, J1-eq,1-ax = 12.9 Hz, J1-eq,2-ax = 2.7 Hz, 1H, 1-eq CH2), 2.48 (dt, J4-eq,4-ax = 13.8 Hz, J4-eq,(3-ax,3-eq) = 2.7 Hz, 1H, 4-eq CH2), 2.29 (sep, J11,(12,13) = 6.9 Hz, 1H, 11-CH), 2.16 (t, J1-ax,(1-eq,2-ax) = 12.9 Hz, 1H, 1-ax CH2), 1.55 (m + qd, 3H, 2-ax CH/3-eq CH2/3-ax CH2), 1.18 (d, J12,11 = 6.9 Hz, 3H, 12-CH3), 1.10 (d, J10,2-ax = 6.3 Hz, 3H, 10-CH3), 1.01 (td, J4-ax,(4-eq,3-ax) = 13.4 Hz, J4-ax,3-eq = 4.5 Hz, 1H, 4-ax CH2), 0.27 (d, J13,11 = 6.6 Hz, 3H, 13-CH3); 13C NMR: (300 MHz, CDCl3): δ 188.3 (C), 157.1 (C), 149.5 (C), 144.3 (C), 120.1 (CH), 111.6 (CH), 110.6 (CH), 61.1 (C), 55.69 (OCH3), 38.0 (CH2), 36.9 (CH), 33.8 (CH2), 29.4 (CH2), 28.6 (CH), 22.2 (CH3), 16.8 (CH3), 16.5 (CH3); EI-MS: m/z (rel. abund.%): 257 (M)+, 242, 214, 173; HR-EIMS: calcd for C17H23NO (M): m/z 257.1780, found: 257.1778.
(2R,4aR)-4a-Isopropyl-2-methyl-2,3,4,4a-tetrahydro-1H-carbazole-6-carbonitrile (2q). Yield = 30%; white solid; Rf = 0.16 (EtOAc/hex 2
:
8); IR (CH2Cl2): νmax cm−1: 2958, 2926, 2860, 2225 (C
N), 1578 (C
N), 1456; UV (MeOH) nm: 272, 267, 225; 1H NMR: (300 MHz, CDCl3): δ 7.60 (s + d, 3H, H-5/H-7/H-8), 2.85 (ddd, J1-eq,1-ax = 13.1 Hz, J1-eq,2-ax = 4.2 Hz, J1-eq,3-eq = 1.8 Hz, 1H, 1-eq CH2), 2.56 (dt, J4-eq,4-ax = 13.8 Hz, J4-eq,(3-ax,3-eq) = 2.7 Hz, 1H, 4-eq CH2), 2.34 (sep, J11,(12,13) = 6.9 Hz, 1H, 11-CH), 2.24 (t, J1-ax,(1-eq,2-ax) = 12.9 Hz, 1H, 1-ax CH2), 1.56 (m + qd, 3H, 2-ax CH/3-eq CH2/3-ax CH2), 1.22 (d, J12,11 = 6.9 Hz, 3H, 12-CH3), 1.14 (d, J10,2-ax = 6.3 Hz, 3H, 10-CH3), 1.00 (td, J4-ax,(4-eq,3-ax) = 13.5 Hz, J4-ax,3-eq = 2.4 Hz, 1H, 4-ax CH2), 0.25 (d, J13,11 = 6.6 Hz, 3H, 13-CH3); 13C NMR: (300 MHz, CDCl3): δ 194.9 (C), 159.4 (C), 143.8 (C), 132.6 (CH), 126.8 (CH), 120.9 (CH), 119.7 (CN), 107.6 (C), 61.7 (C), 38.4 (CH2), 37.0 (CH), 33.7 (CH2), 29.0 (CH2), 29.0 (CH), 22.1 (CH3), 17.2 (CH3), 16.4 (CH3); EI-MS: m/z (rel. abund.%): 252 (M)+, 237, 210, 168; HR-EIMS: calcd for C17H23N2 (M): m/z 252.1626, found: 252.1623.
4.3. Assay for AChE and BChE inhibitory activity
Cholinesterase inhibitory activities were measured by modifying the Ellman's spectrophotometric method.23 Acetylthiocholine iodide and butyrylthiocholine chloride were used as substrates, while 5,5′-dithio-bis(2-nitrobenzoic) acid (DTNB) was used for measuring the activity. 150 μL of 100 mM sodium phosphate buffer (pH = 8.0), 10 μL of test compound solution in 0.2 mM concentration and 20 μL acetylcholinesterase or butyrylcholinesterase solution were mixed and incubated for 15 min at 25 °C. The reaction was initiated with the addition of 10 μL DTNB and 10 μL acetylthiocholine iodide or butyrylthiocholine chloride. Enzymatic hydrolysis of acetylthiocholine iodide or butyrylthiocholine chloride yielded corresponding choline that reacted with DTNB to yield yellow 5-thio-2-nitrobenzoate anion. This resulting yellow colored anion was monitored spectrophotometrically at a wavelength of 412 nm (SpectraMax-340). The test compounds and the controls were dissolved in methanol. All the reactions were performed in a triplicate.
4.4. Determination of kinetic parameters
The type of inhibition was determined by Lineweaver–Burk plots and its secondary plots. Effect of the inhibitor (test sample) on both Km (Michaelis–Menten constant) and Vmax (maximal velocity) values was monitored by using Lineweaver–Burk plot which was plotted between the reciprocal of the rate of the reactions and the reciprocal of the substrate concentration. The secondary replots of the Lineweaver–Burk were made by two methods; first reciprocal of Vmax, determined form intersection point on the y-axis of Lineweaver–Burk plot, was plotted against different concentrations of the respective inhibitor. Secondly, for the mixed-type inhibitions, the slope of line of concentration of inhibitor on Lineweaver–Burk plot was plotted against concentration of inhibitor. Ki Values (the dissociation constant of enzyme–inhibitor complex) were calculated from Dixon and Lineweaver–Burk plots, whereas K′i Values (the dissociation constant of substrate bound enzyme–inhibitor complex) were calculated from replots of Lineweaver–Burk plot.
4.5. Assay for xanthine oxidase inhibitory activity
Xanthine oxidase inhibitory activity was performed by using literature procedure.30 Xanthine oxidase (0.003 units per well) from butter milk was dissolved in Phosphate buffer (pH 7.4, 50 mM), whilst solution of test samples was made in DMSO. 10 μL of test sample, 20 μL of enzyme and 150 μL of buffer was taken in 96 well plates and allowed to react at 30 °C for 10 min. After pre-incubation, 20 μL of xanthine (0.15 mM) was added and change in absorbance was measured at 295 nm for 30 min. Allopurinol was taken as positive control. % inhibition was calculated using formula: 100 − [(ODtest compound/ODcontrol) × 100].
4.6. Assay for α-chymotrypsin inhibitory activity
α-Chymotrypsin inhibitory activity is conducted by modifying Carnell's procedure.31 α-chymotrypsin (12 Units per mL prepared in buffer) and test compound (0.5 mM) were taken in DMSO and pre-incubated for 25 min at 30 °C. The reaction commenced by the addition of N-succinyl-L-phenylalanine-p-nitroanilide (0.4 mM prepared in the buffer) and change in absorbance due to release of p-nitroanilide was continuously monitored at λmax 410 nm. The % inhibition was calculated using formula: [(OD/min of test compound/(OD/min of 100 − positive control)] × 100.
4.7. Assay for urease inhibitory activity
Urease inhibitory activity was performed by determining ammonia production according to indophenol method.32 The mixture of 25 μL of enzyme (Jack bean urease) and 55 μL of buffers having 100 mM urea was incubated with 5 μL of test compounds (0.5 mM) in 96-well plates for 15 min at 30 °C. After that, 45 μL each phenol reagent (1% w/v phenol and 0.005% w/v sodium nitroprusside) and 70 μL of alkali reagent (0.5% w/v NaOH and 0.1% active chloride NaOCl) were introduced in each well and pH was maintained at 6.8. Thiourea was used as the standard inhibitor of urease. Change in absorbance was measured after 50 min using a microplate reader (Molecular Device, USA) at 630 nm and change in absorbance per min was processed using softMax Pro software (molecular Device, USA). % inhibition was calculated using formula: 100 − (ODtestwell/ODcontrol) × 100.
4.8. Assay for phosphodiesterase-I inhibitory activity
Phosphodiesterase-I inhibitory activity was performed using snake venom according to procedure given in literature.33 All experiments were conducted in triplicate. 33 mM Tris–HCl buffer (pH 8.8) and 30 mM Mg-acetate were taken in a microtiter plate with 0.000742 U per well final concentration of enzyme. 0.33 mM bis-(p-nitropheny1) phosphate (Sigma) was used as substrate, while cystein and EDTA (Merck) were used as positive controls. Enzyme and test samples were pre-incubated for 30 min and monitored spectrophotometrically on a microtitre plate reader (SpectraMax, Molecular Devices) at 37 °C. Rate (change in O.D/min) of release of p-nitrophenol from p-nitrophenyl phosphate was measured at 410 nm.
4.9. Assay for carbonic anhydrase-II inhibitory activity
Carbonic anhydrase-II inhibitory activity was carried out by monitoring hydrolysis of 4-nitrophenyl acetate (4-NPA) to 4-nitrophenol (a yellow colored compound) according to reported procedure.34 The reaction was conducted in 20 mM buffer containing HEPES and Tris (pH 7.4) at 25–28 °C. 140 μL of HEPES–Tris solution, 20 μL of aqueous solution of purified bovine erythrocyte carbonic anhydrase II (freshly prepared, 0.1 mg mL−1 of deionized water for 96-well), 20 μL of test compound in DMSO and 20 μL of 0.7 mM 4-PNA in ethanol was added to 96-well plate and allowed to react for 15 min. Change in absorbance due to release of 4-nitrophenol was formed was measured using SPECTRA-max 340 spectrophotometer, Molecular Devices (USA) at 400 nm for 30 min (after 1 min interval).
4.10. Assay for 1,1-diphenyl-2-picrylhydrazyl radical (DPPH) scavenging activity
1,1-Diphenyl-2-picrylhydrazyl radical (DPPH) scavenging assay was performed according to literature protocol.35 95 μL of DPPH in ethanol (300 μM) and 5 μL of test sample in DMSO (1 mM) were mixed in 96-well microtiter plates (Molecular Devices, Spectra Max 340, USA) and incubated at 37 °C for 30 min. Propyl gallate (PG) was used as positive control and decrease in absorbance was measured at λmax 515 nm. The % radical scavenging activity of test samples in comparison with a DMSO treated control group was determined using the formula: 100 − [(ODtest compound/ODcontrol) × 100].
4.11. Assay for cytotoxicity against PC-3 cell lines
Cytotoxicity assay was done using freshly isolated human neutrophils according to literature protocol given in literature.36 Freshly isolated human neutrophils (1 × 107 cells per mL) and test sample with different concentrations were mixed up to total volume of 200 μL and pre-incubated at 450 nm for 30 min. After taking pre-incubation reading, WST-1 (250 μM) was added and plate was re-incubated for 3 h with shaking in water bath at 37 °C. Absorbance was measured at 450 nm using microplate reader (Spectra Max 340, molecular sieves) and % cell viability is calculated using formula: [(ODtest compound × 100/ODcontrol) − 100].
Conflict of interest
The authors have declared no conflict of interest.
Acknowledgements
We are grateful to H. E. J., Research Institute of Chemistry, International Center for Chemical and Biological Sciences, University of Karachi, Karachi-75270, Pakistan and Higher Education Commission, Pakistan for providing financial assistance.
References
- Alzheimer's Association, Alzheimer's disease facts and figures, Alzheimer's Dementia, 2014, 10, e47–e92 CrossRef.
- I. Bolea, J. Juárez-Jiménez, C. de los Ríos, M. Chioua, R. Pouplana, F. J. Luque, M. Unzeta, J. Marco-Contelles and A. Samadi, J. Med. Chem., 2011, 54, 8251–8270 CrossRef CAS PubMed.
- N. Toda, K. Tago, S. Marumoto, K. Takami, M. Ori, N. Yamada, K. Koyama, S. Naruto, K. Abe and R. Yamazaki, Bioorg. Med. Chem., 2003, 11, 1935–1955 CrossRef CAS PubMed.
- A. N. Çokuğraş, Turk. J. Biochem., 2003, 28, 54–61 Search PubMed.
- N. H. Greig, T. Utsuki, D. K. Ingram, Y. Wang, G. Pepeu, C. Scali, Q.-S. Yu, J. Mamczarz, H. W. Holloway and T. Giordano, Proc. Natl. Acad. Sci. U. S. A., 2005, 102, 17213–17218 CrossRef CAS PubMed.
- M. M. Ismail, M. M. Kamel, L. W. Mohamed and S. I. Faggal, Molecules, 2012, 17, 4811–4823 CrossRef CAS PubMed.
- X.-Y. Zheng, Z.-J. Zhang, G.-X. Chou, T. Wu, X.-m. Cheng, C.-H. Wang and Z.-T. Wang, Arch. Pharmacal Res., 2009, 32, 1245–1251 CrossRef CAS PubMed.
- L. Savini, G. Campiani, A. Gaeta, C. Pellerano, C. Fattorusso, L. Chiasserini, J. M. Fedorko and A. Saxena, Bioorg. Med. Chem. Lett., 2001, 11, 1779–1782 CrossRef CAS PubMed.
- J. Badiger, K. Manjulatha, M. Girish, A. Sharif and M. Purohit, ARKIVOC, 2009, 12, 217–231 Search PubMed.
- A. Ataei-Azimi, B. D. Hashemloian, H. Ebrahimzadeh and A. Majd, Afr. J. Biotechnol., 2008, 7, 2834–2839 CAS.
- A. D. Napper, J. Hixon, T. McDonagh, K. Keavey, J.-F. Pons, J. Barker, W. T. Yau, P. Amouzegh, A. Flegg and E. Hamelin, J. Med. Chem., 2005, 48, 8045–8054 CrossRef CAS PubMed.
- S. Štolc, Life Sci., 1999, 65, 1943–1950 CrossRef.
- A. Chaskar, H. Deokar, V. Padalkar, K. Phatangare and S. Patil, J. Korean Chem. Soc., 2010, 54, 411–413 CrossRef CAS.
- I. Andreadou, A. Tasouli, E. Bofilis, M. Chrysselis, E. Rekka, A. Tsantili-Kakoulidou, E. Iliodromitis, T. Siatra and D. T. Kremastinos, Chem. Pharm. Bull., 2002, 50, 165–168 CrossRef CAS PubMed.
- C. Q. Shi, Z. Q. Liu, W. Q. Lin and Y. W. Chen, Chin. Chem. Lett., 2007, 18, 899–901 CrossRef CAS.
- P. G. Becher, J. Beuchat, K. Gademann and F. Jüttner, J. Nat. Prod., 2005, 68, 1793–1795 CrossRef CAS PubMed.
- Y. Rook, K.-U. Schmidtke, F. Gaube, D. Schepmann, B. Wünsch, J. R. Heilmann, J. Lehmann and T. Winckler, J. Med. Chem., 2010, 53, 3611–3617 CrossRef CAS PubMed.
- N. Khorana, K. Changwichit, K. Ingkaninan and M. Utsintong, Bioorg. Med. Chem. Lett., 2012, 22, 2885–2888 CrossRef CAS PubMed.
- T. Kondo, T. Okada and T.-A. Mitsudo, J. Am. Chem. Soc., 2002, 124, 186–187 CrossRef CAS PubMed.
- G. A. Kraus and H. Guo, Org. Lett., 2008, 10, 3061–3063 CrossRef CAS PubMed.
- X. Li, Y. Du, Z. Liang, X. Li, Y. Pan and K. Zhao, Org. Lett., 2009, 11, 2643–2646 CrossRef CAS PubMed.
- F. M. Miller and W. N. Schinske, J. Org. Chem., 1978, 43, 3384–3388 CrossRef CAS.
- G. L. Ellman, K. D. Courtney, V. Andres Jr and R. M. Featherstone, Biochem. Pharmacol., 1961, 7, 88–95 CrossRef CAS PubMed.
- Y. Kia, H. Osman, R. Suresh Kumar, A. Basiri and V. Murugaiyah, Bioorg. Med. Chem. Lett., 2014, 24, 1815–1819 CrossRef CAS PubMed.
-
(a) SMART, Data Collection Software, Version 4.050, Siemens Analytical X-Ray Instruments Inc., Madison, WI, 1996 Search PubMed;
(b) SAINT, Data Reduction Software, Version 4.050, Siemens Analytical X-Ray Instruments Inc., Madison, WI, 1996 Search PubMed.
- P. T. Beurskens, G. Admiraal, G. Beurskens, W. P. Bosman, R. de Gelder, R. Israel and J. M. M. Smits, The DIRDIF-94 program system, technicall report of the crystallography laboratory, University of Nijmegen, Netherlands, 1994 Search PubMed.
- G. M. Sheldrick, SHELXTL 5.04/VMS, An integrated system for solving, rening and displaying crystal structures from diraction data, Siemens Analytical X-ray Instruments Inc., Madison, WI, 1995 Search PubMed.
- G. M. Sheldrick, SHELXTL-PC (Version 5.1), Siemens Analytical Instruments Inc, WI, USA, Madison, 1997 Search PubMed.
- C. K. Johnson, ORTEP: ORTEPII, Report ORNL-5138, Oak Ridge National Laboratory, Oak Ridge, Tennessee, 1976 Search PubMed.
- M. A. Whidden, J. M. McClung, D. J. Falk, M. B. Hudson, A. J. Smuder, W. B. Nelson and S. K. Powers, J. Appl. Physiol., 2009, 106, 385–394 CrossRef CAS PubMed.
- W. E. Razzell and H. G. Khorana, J. Biol. Chem., 1959, 234, 2114–2117 CAS.
- M. Weatherburn, Anal. Chem., 1967, 39, 971–974 CrossRef CAS.
- R. Mamillapalli, R. Haimovitz, M. Ohad and M. Shinitzky, FEBS Lett., 1998, 436, 256–258 CrossRef CAS PubMed.
- R. P. Shank, D. R. Doose, A. J. Streeter and M. Bialer, Epilepsy Res., 2005, 63, 103–112 CrossRef CAS PubMed.
- I. Khan, S. Ali, S. Hameed, N. H. Rama, M. T. Hussain, A. Wadood, R. Uddin, Z. Ul-Haq, A. Khan, S. Ali and M. I. Choudhary, Eur. J. Med. Chem., 2010, 45, 5200–5207 CrossRef CAS PubMed.
- T. Mosmann, J. Immunol. Methods, 1983, 65, 55–63 CrossRef CAS PubMed.
Footnote |
† Electronic supplementary information (ESI) available: 1H and 13C NMR spectra of all novel 2,3,4,4a-tetrahydro-1H-carbazole compounds are provided in the supplementary information. Kinetic data of some compounds including active have also been provided. CCDC 949401. For ESI and crystallographic data in CIF or other electronic format see DOI: 10.1039/c5ra10461k |
|
This journal is © The Royal Society of Chemistry 2015 |