DOI:
10.1039/C5RA09517D
(Paper)
RSC Adv., 2015,
5, 59167-59185
One-pot three-component synthesis of 1,2,3-triazoles using magnetic NiFe2O4–glutamate–Cu as an efficient heterogeneous catalyst in water†
Received
21st May 2015
, Accepted 1st July 2015
First published on 2nd July 2015
Abstract
A novel magnetic NiFe2O4 supported glutamate–copper catalyst was prepared in a convenient and ecofriendly manner and characterized using FTIR, XRD, EDX, TEM, SEM, XPS and VSM. This magnetic catalyst exhibited excellent catalytic activity for the synthesis of a wide variety of 1,4-disubstituted-1,2,3-triazoles via one-pot three-component click reactions of sodium azide, non-activated terminal alkynes, and different azide precursors such as epoxides, benzyl chlorides, and aryl boronic acids at room temperature in water. This procedure has some advantages such as an aqueous reaction medium, ambient reaction conditions, high yields, wide substrate scope, easy separation of the catalyst using an external magnet and efficient recycling.
Introduction
Heterocyclic compounds containing nitrogen-atoms occupy an important position in natural product and medical chemistry. 1,2,3-Triazoles are well-known five-membered heterocyclic molecules that have attracted much interest due to their wide range of applications in the fields of pharmaceuticals, agrochemicals, and materials chemistry.1 The interesting properties of 1,2,3-triazoles encourage the development of convenient and mild methods for their synthesis.2 The most popular method for the construction of the 1,2,3-triazole framework is the Cu(I)-catalyzed 1,3-dipolar Huisgen cycloaddition reaction of azides with alkynes (CuAAC).3 However, this procedure involves a one-pot two component reaction in which azides were prepared prior to the cycloaddition.4 To overcome this drawback, one-pot three-component methodologies for in situ generation of azides have been developed. Various different azide precursors such as epoxides, benzyl halides, and aryl boronic acids have been used in this CuAAC reaction to construct a wide range of 1,4-disubstituted-1,2,3-triazole framework. In general, homogeneous copper(I) catalysts have been used to catalyze these three-component reactions.5 In order to overcome the difficulty of catalyst separation, some heterogeneous catalytic system have been developed, including Cu(II)-hydrotalcite,6 Cu(I)-modified zeolite,7 copper nanoparticles on activated carbon,8 Cu(I)@phosphorated SiO2 (ref. 9) copper supported on the SiO2 nanoparticle,10 CuFe2O4 magnetic nanoparticles11 for the synthesis of β-hydroxy-1,2,3-triazoles, copper nanoparticles supported on agarose,12 Cu(I) on waste oyster shell powder,13 Fe3O4 magnetic nanoparticle (MNP)-supported copper(I),14 Cu(I) on charcoal,15 copper nanoparticles supported on activated carbon,16 nano ferrite–glutathione–copper (nano-FGT–Cu),17 Cu(I) supported on alumina (Cu/Al2O3),18 polymer-supported copper,19 Cu2O nanocubes,20 copper immobilized onto a triazole functionalized magnetic nanoparticle,21 cellulose supported cuprous iodide nanoparticles,22 supported CuBr on graphene oxide/Fe3O4,23 Cu(II) PBS-bridged PMOs24 for the synthesis of 1-alkyl-4-aryl-1,2,3-triazoles, and CuFe2O4 nanoparticles,25 Fe3O4 nanoparticle-supported Cu(II)–β-cyclodextrin complex,26 clay supported Cu(II)27 for the synthesis of 1-aryl-1,2,3-triazoles. Despite these achievements, some reported catalytic systems have significant limitations such as the lack of regioselectivity, high temperature, prolonged reaction times and limited substrate scope. Therefore, the further development of simple, efficient, eco-friendly and economically viable processes for the synthesis of 1,2,3-triazoles is still highly desirable.
In view of the principles of green chemistry, the improvement of reaction process, media and catalyst, is still the challenging in the current chemistry. In this regard, multicomponent reactions (MCRs) has been adopted as a practical and extraordinary synthetic tool to create molecular complexity and biologically active heterocyclic compounds in a single reaction vessel ensuring high atom economy, good overall yields and high selectivity, shorter reaction times, operational simplicity, minimizing waster and energy.28 Furthermore, replacement of conventional hazardous organic solvents by safe and green reaction media has always been a thread in the sustainable chemistry.29 There is no doubt that, water can be considered as an attractive green solvent, because it is abundant, non-toxic and environmentally benign. Besides, water is also known to enhance the reaction rates and effect the selectivity of a lot of organic transformations owing to its strong hydrogen bonding ability.30 In addition, for economic and industrial point of view, the development of efficient and recoverable heterogeneous catalyst systems become one of the most important topics of research in organic synthesis. Various materials have been employed as the support to produce heterogeneous catalyst, such as mesoporous silica,31 activated carbon,32 polymer,33 and biomass.34 Recently, magnetic nanoparticles have emerged as a robust, high-surface-area heterogeneous catalyst support. Magnetic recoverability is an additional attribute of these materials compared to most of the heterogeneous catalyst deployed as it eliminates the necessity of the catalyst filtration or centrifugation after completion of the reaction.35–37 With these three aspects of green synthesis in mind, the combination of our interest in designing novel magnetic nanocatalysts38 and the development of environmentally benign synthetic methodologies,39 herein, we report the preparation, characterization and investigation of catalytic activity of magnetic nano NiFe2O4–glutamate–Cu for synthesis of a wide variety of 1,4-disubstituted-1,2,3-triazoles via one-pot three-component click reactions of sodium azide, non-activated terminal alkynes, and different azide precursors such as epoxides, benzyl chlorides, and aryl boronic acids in water at room temperature (Scheme 1).
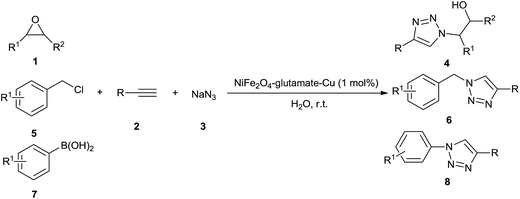 |
| Scheme 1 One-pot synthesis of 1,2,3-triazoles catalyzed by NiFe2O4–glutamate–Cu in water. | |
Results and discussion
As shown in Scheme 2, the catalyst was prepared in a three-step process. At first, NiFe2O4 NPs were synthesized by a chemical precipitation technique of FeCl3 and NiCl2 in the basic condition. Then, the anchoring L-glutamic acid on the surface of NiFe2O4 NPs was done in MeOH, followed by the addition of Cu(OAc)2 and NaBH4 at a basic condition, affording the immobilized Cu complex catalyst NiFe2O4–glutamate–Cu(0). Characterization of the prepared novel magnetic nano catalyst was performed by different physicochemical methods such as X-ray diffraction (XRD), Fourier transform infrared (FT-IR), energy dispersive spectrum (EDS), transmission electron microscopy (TEM), scanning electron microscopy (SEM), vibrating sample magnetometry (VSM), and inductively coupled plasma mass spectrometry (ICP-MS).
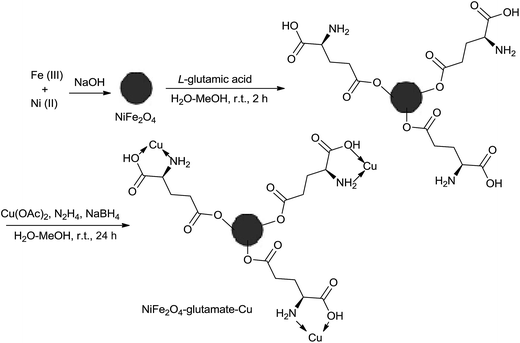 |
| Scheme 2 Synthesis of NiFe2O4–glutamate–Cu. | |
Fig. 1 shows the XRD patterns of the prepared NiFe2O4–glutamate–Cu(0) nanoparticles. The position and relative intensities of observed diffraction peaks appearing at 2θ = 30.56°, 36.00°, 37.2°, 57.68° and 63.40° corresponding to the diffractions of (2 2 0), (3 1 1), (2 2 2), (4 2 2) and (4 4 0), well matched with literature data of NiFe2O4 (JCPDS 10-0325), indicating retention of the crystalline cubic spinel structure during functionalization of MNPs. The XRD data (JCPDS 01-085-1326) clearly demonstrates the presence of metallic copper with the characteristic (1 1 1) and (2 0 0) Bragg's peaks located at 2θ = 43.77° and 2θ = 50.81° respectively. No impurity peak was observed, indicating that high purity crystalline NiFe2O4–glutamate–Cu(0) nanoparticles were successfully synthesized.
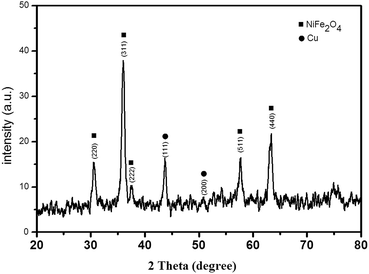 |
| Fig. 1 XRD pattern of NiFe2O4–glutamate–Cu. | |
The FT-IR spectra of NiFe2O4, NiFe2O4 anchored with glutamic acid and assembly of magnetic nanoparticles and complex of glutamic acid with Cu (NiFe2O4–glutamate–Cu) are depicted in Fig. 2. The presence of Fe–O and Ni–O bonds in the magnetic particles is confirmed by the characteristic peaks appeared at 1038 and 411 cm−1, respectively. The absorption at 604 cm−1 is assigned to the Cu–O bridge. The bands at 1624, 1482, 1336, 1383, 1007, and 946 cm−1 are the characteristic absorptions of glutamate, which shows evidence for the formation of a glutamate shell.
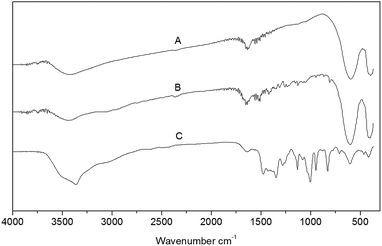 |
| Fig. 2 IR spectra of NiFe2O4 (A), NiFe2O4–glutamate (B) and NiFe2O4–glutamate–Cu (C). | |
The EDS of NiFe2O4–glutamate–Cu also shows that the elements, including Fe, Ni, O, C, N and Cu are present in the structure of this material (Fig. 3). Also, to verify the Cu content in the catalyst, it was treated with concentrated HCl and HNO3 (3/1 ratio of HCl and HNO3) to digest the material and then was analyzed by inductively coupled plasma mass spectrometry (ICP-MS) analysis. The Cu content was found to be 10.3%.
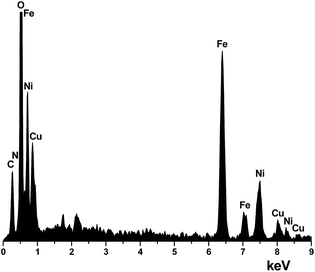 |
| Fig. 3 EDS spectrum of NiFe2O4–glutamate–Cu. | |
The chemical composition of the NiFe2O4–glutamate–Cu nanoparticles were also characterized by X-ray photoelectron spectroscopy (XPS) analysis (Fig. 4), which revealed the characteristic peaks for C 1s (283.8 eV), O 1s (530.0 eV), Fe 2p (711.6 eV), Ni 2p (855.7 eV), and Cu 2p (936.7 eV). Furthermore, the high-resolution narrow scan for Cu 2p in NiFe2O4 displays energy peaks of 2p3/2 and Cu 2p5/2 at 933.2 and 953.3 eV, clearly indicating the formation of the Cu(0) nanoparticles on the NiFe2O4 nanosheets (Fig. 4b).
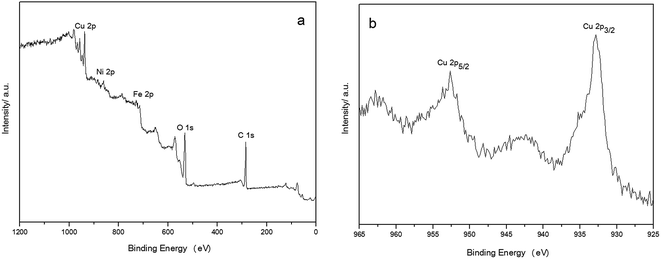 |
| Fig. 4 XPS spectrum of NiFe2O4–glutamate–Cu: wide scan (a), high-resolution spectrum for the Cu (b). | |
The shape, size and morphology of the synthesized NiFe2O4–glutamate–Cu NPs were analyzed by using TEM and SEM. The TEM image shows that NiFe2O4–glutamate–Cu NPs have a core–shell structure. Further observation confirmed the formation of a glutamate layer around the NiFe2O4 nanoparticles (Fig. 5). The SEM image shows that morphology of the particles is spherical or quasi-spherical and the surface configuration of NPs is quite rough with smaller subunits (Fig. 6). The average nanoparticle diameter of NiFe2O4–glutamate–Cu was estimated to be 20–22 nm based on the TEM image, which is also in accordance with the result calculated by the Debye–Scherrer's equation. The specific surface area of NiFe2O4–glutamate–Cu(0) obtained by the N2 adsorption isotherms was 37.0 m2 g−1, pore volume was 0.315 cm−3 g−1 and pore diameter was 11.02 nm.
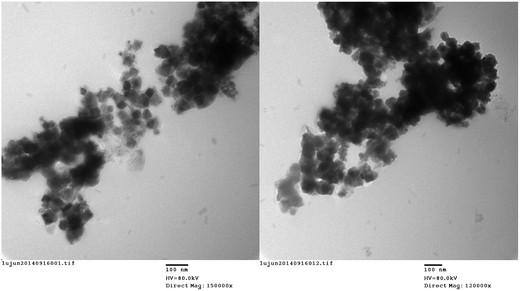 |
| Fig. 5 TEM micrographs of NiFe2O4–glutamate–Cu: (left) “fresh” catalyst and (right) “recovered” catalyst. | |
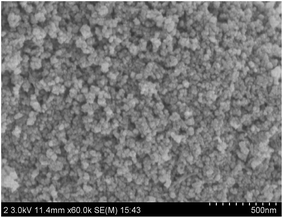 |
| Fig. 6 SEM image of NiFe2O4–glutamate–Cu. | |
The magnetic properties of the prepared NiFe2O4–glutamate–Cu(0) were measured by VSM at room temperature with the field sweeping from −30
000 to +30
000 oersted (Fig. 7). The magnetic hysteresis curve of NiFe2O4–glutamate–Cu(0) revealed that it possessed superparamagnetic behavior, and its saturation magnetization values was found to be 33.8 emu g−1, indicating that it could be efficiently separated by an external permanent magnet.
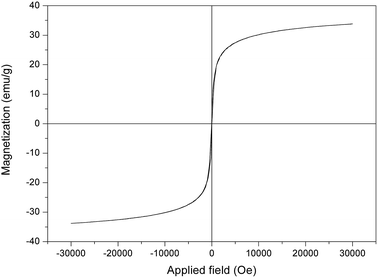 |
| Fig. 7 Magnetization curve of NiFe2O4–glutamate–Cu. | |
In order to study the efficiency of the prepared NiFe2O4–glutamate–Cu, the reaction of styrene oxide, sodium azide and ethynylbenzene is adopted as a model reaction. The influence of different parameters such as kinds of catalysts, solvents, catalyst concentration was examined to obtain the best possible combination. When the reaction was attempted without a catalyst in water, that product was not detected even after 6 h (Table 1, entry 1). Some copper salts such as CuSO4·5H2O and Cu(OAc)2·H2O, nano materials such as nano Fe3O4, γ-Fe2O3, CoFe2O4, NiFe2O4, CuFe2O4 and CuFeO2 were test. However, the reaction efficiency was not improved. A clear improvement of the yield was observed when CuI, CuCl, CuFeO2 was added, however, the yields are still far from satisfactory. Considering the importance of ligands in the traditional copper-catalyzed reactions, 10-phenantholine (10 mol%) was added to above model reaction in the presence of CuFeO2. It was observed that the yield was significantly improved (entry 12). To our delight, the use of our prepared NiFe2O4–glutamate–Cu gave 4a in an excellent yield (entry 13).
Table 1 Screening catalysts for the three-component reaction of styrene oxide, ethynylbenzene and sodium azidea
Then, the influence of solvents on the reaction was also investigated. Among the different solvents screened, water was found to be the best solvent of choice and afforded the product in high yield. The use of the mixture of ethanol and water afforded the desired product in lower yield. The formatting product 4a was not observed using ethanol, dichloromethane, toluene, acetonitrile, and N,N-dimethylformamide (DMF) as solvents.
The amount of catalyst required for this transformation was investigated. The result showed that 1 mol% of catalyst was sufficient to complete the reaction. The use of excessive catalyst neither increased the yield nor shortened the reaction time, while a decrease in the amount of catalyst decreased the yield. Based on the above results, the optimal conditions were established to be the use of 1 mol% NiFe2O4–glutamate–Cu as the catalyst in H2O at room temperature.
Again, in order to demonstrate the practicality of this process, we investigated the scalability of this model reaction on a 50 mmol scale. Indeed, the reaction proceeded similar to the case on a smaller scale and the desired product was obtained in 96% yield in 1.5 h (Table 1, entry 22).
For a heterogeneous catalyst, it is important to test the ease of separation, recoverability and reusability in the practical applications. The recyclability of the catalyst was investigated in model reaction. After completion of the reaction, the product was extracted with ethyl acetate. The catalyst was recovered by an external magnet and washed several times with ethyl acetate. Then it was reused in the subsequent run after dying under vacuum at 60 °C. As shown in Fig. 8, the recovered catalyst couple be directly reused in ten successive runs without significant loss of activity. It is clear from the TEM micrograph in Fig. 5 that the catalyst is stable after reaction.
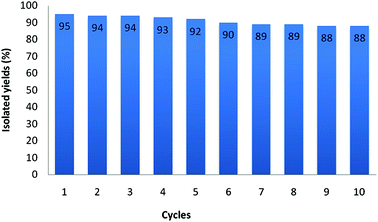 |
| Fig. 8 Reusability of NiFe2O4–glutamate–Cu in the model reaction. | |
With the optimum reaction conditions in hand, the scope and generality of this three-component reaction were explored by treating different epoxides, terminal alkynes and sodium azide to generate various hydroxymethylated 1,2,3-triazoles. We first investigated structurally diverse terminal alkynes under the optimal conditions. As shown in Table 2, the reaction is quite general and the corresponding triazoles were obtained in good to excellent yields. Various aryl alkynes, regardless of the presence of electron-donating or electron-withdrawing groups reacted with styrene oxide and sodium azide smoothly to afford the desired products in high to excellent yields with high regioselectivity. Heterocyclic alkyne such as 2-pyridylacetylene worked well and provided the expected in 80% yield (Table 2, entry 12). Surprisingly, this three-component reaction also proceeded successfully in the case of aliphatic alkynes although the yields were lower than aryl alkynes (entries 13 and 14).
Table 2 Scope of reaction of epoxides with alkynes and sodium azide catalyzed by magnetic NiFe2O4–glutamate–Cu

|
Entry |
Epoxide |
Alkyne |
Product |
Time (h) |
Yielda (%) |
M.p. (°C) |
Isolated yield. Chiral HPLC conditions: Chiralpak OD-H, hexane/isopropanol = 95 : 5, flow rate = 1.0 mL min−1, wavelength = 245 nm, retention time: 47.3 min (minor), 58.9 nm (major). |
1 |
 |
 |
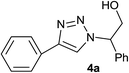 |
1.5 |
95 |
126–127, 125–127 (ref. 8) |
2 |
 |
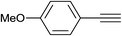 |
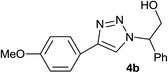 |
2.5 |
88 |
138–139 |
3 |
 |
 |
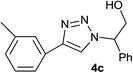 |
4.0 |
86 |
115–116 (ref. 6) |
4 |
 |
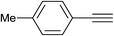 |
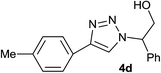 |
2.0 |
90 |
126–127, 125–127 (ref. 9) |
5 |
 |
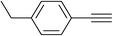 |
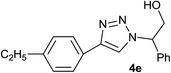 |
2.0 |
93 |
146–147 |
6 |
 |
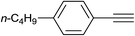 |
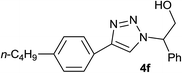 |
2.5 |
92 |
124–125 |
7 |
 |
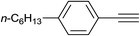 |
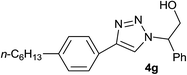 |
2.5 |
87 |
105–106 |
8 |
 |
 |
 |
2.5 |
90 |
114–115 (ref. 11) |
9 |
 |
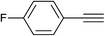 |
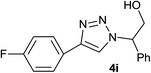 |
2.0 |
93 |
99–100 |
10 |
 |
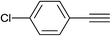 |
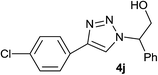 |
2.5 |
93 |
128–129 |
11 |
 |
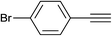 |
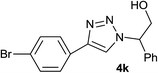 |
3.0 |
85 |
149–150 |
12 |
 |
 |
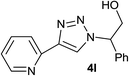 |
4.0 |
80 |
130–131, 112.7–115.3 (ref. 8) |
13 |
 |
 |
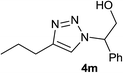 |
5.0 |
71 |
61–62, 62–64 (ref. 9) |
14 |
 |
 |
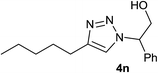 |
6.0 |
62 |
Oil |
15 |
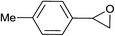 |
 |
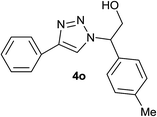 |
2.5 |
90 |
124–126, 125–127 (ref. 9) |
16 |
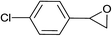 |
 |
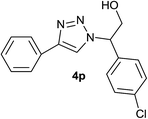 |
5.0 |
88 |
140–141, 139.6–142.1 (ref. 8) |
17 |
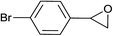 |
 |
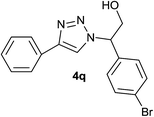 |
5.0 |
85 |
170–171 |
18 |
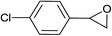 |
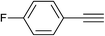 |
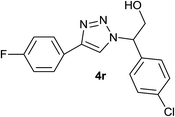 |
5.0 |
80 |
120–121 |
19 |
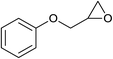 |
 |
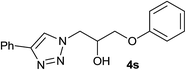 |
4.0 |
86 |
125–126, 125–127 (ref. 8) |
20 |
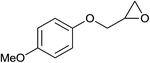 |
 |
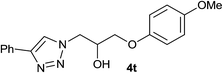 |
2.5 |
92 |
110–111 (ref. 11) |
21 |
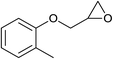 |
 |
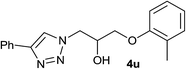 |
2.5 |
90 |
Oil (ref. 11) |
22 |
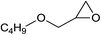 |
 |
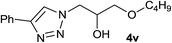 |
3.0 |
90 |
Oil |
23b |
 |
 |
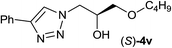 |
3.0 |
89 |
Oil |
24 |
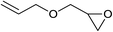 |
 |
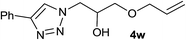 |
2.5 |
93 |
71–72, 71.5–72 (ref. 5c) |
25 |
 |
 |
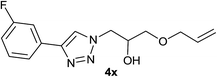 |
3.0 |
85 |
Oil |
26 |
 |
 |
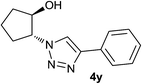 |
2.5 |
90 |
Oil |
27 |
 |
 |
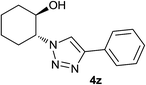 |
2.5 |
89 |
170–171, 168–171 (ref. 9) |
28 |
 |
 |
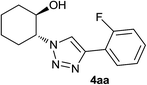 |
4.0 |
85 |
149–150 |
29 |
 |
 |
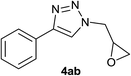 |
2.0 |
95 |
85–86, 82–83 (ref. 40) |
30 |
 |
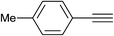 |
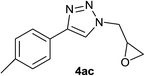 |
2.0 |
90 |
65–66 |
31 |
 |
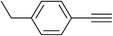 |
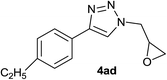 |
2.0 |
93 |
146–147 |
32 |
 |
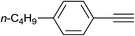 |
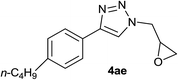 |
3.0 |
90 |
57–58 |
33 |
 |
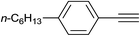 |
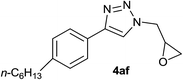 |
2.5 |
87 |
65–66 |
34 |
 |
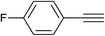 |
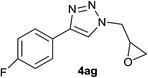 |
2.0 |
93 |
93–94 |
35 |
 |
 |
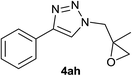 |
3.0 |
92 |
Oil |
Next, the reactivities of various epoxides were evaluated. Satisfactorily, styrene oxide having either electron-donating or electron-withdrawing substituents worked well and delivered expected products in high to excellent yields. In the case of 2-monosubstituted aryl epoxides, a major primary alcohol triazole regioisomer was obtained during the reaction, which was consistent with published report.11 On the other hand, in the case of glycidic ethers, the ring-opening occurred predominantly through an attack by sodium azide at the less substituted carbon atom of the epoxide, followed by cycloaddition with terminal alkynes to afford the major secondary alcohol triazole regioisomer, which is agreement with an SN2 pathway. The probable reason may be that in aryl epoxides the positive charge at benzylic position is stabilized due to conjugation with the phenyl ring, thus leading to the predominant product primary alcohol triazole by nucleophilic attack at the more stable benzylic carbon. Whereas in case of glycidic ethers, steric factor predominates over electronic factors thereby facilitating the attack at the sterically less crowded carbon atom of the epoxide ring. As predicated, some simple aliphatic oxiranes such as 2-(butoxymethyl)oxirane (entry 22), and 2-((allyloxy)methyl)oxirane (entries 24 and 25), secondary alcohol triazole regioisomer was obtained as major product corresponding to ring opening at the terminal oxirane carbon, in agreement with an SN2 pathway. Cyclohexene oxide underwent ring opening exclusively via a trans-stereospecific pathway to provide only one trans isomer (entries 26–28). Encouraged by these results, the reaction was attempted with an enantiomerically pure chiral epoxide (entry 23). To our satisfaction, the corresponding product (S)-4v was obtained in high yield with excellent ee, indicating no racemization during the reaction.
Under the same conditions, epichlorohydrin was also employed in this MCR. It was found that the corresponding epoxytriazoles were obtained in high yields (Table 2, entries 29–35). The resulting product 4ab can also further react with terminal alkynes and sodium azide to give bis(triazoles) 4ai and 4aj (Scheme 3).
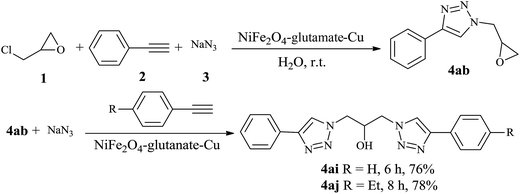 |
| Scheme 3 Synthesis of bis(triazoles). | |
Subsequently, to further demonstrate the applicability of our procedures, we then performed three-component reaction of benzyl chloride, terminal alkynes and sodium azide under above conditions. As shown in Table 3, phenylacetylene derivatives bearing electron-donating or electron-withdrawing substituents on the phenyl group worked well and furnished the corresponding 1-alkyl-4-aryl-1,2,3-triazole derivatives in high yields. While terminal alkynes bearing alkyl groups, as expected, are less reactive in this three-component reaction, and led to lower yield of products (Table 3, entries 6 and 7). Furthermore, benzyl chloride derivatives bearing an electron-donating or electron-withdrawing group on the phenyl ring underwent the one-pot reaction smoothly and provided the desired product in high yields.
Table 3 Scope of reaction of benzyl chloride with alkynes and sodium azide catalyzed by magnetic NiFe2O4–glutamate–Cu
Finally, it was found that this catalytic system can efficiently catalyze one-pot aromatic azidonation of aryl boronic acids followed by regioselective azide–alkyne 1,3-dipolar cycloaddition reaction to obtain corresponding 1-aryl-1,2,3-triazole derivatives. Various different aromatic and aliphatic alkynes were investigated. As shown in Table 4, the reaction proceeded smoothly with substituted aromatic ethynylbenzenes possessing electronically different substituents and afforded high yields of the desired products (Table 4, entries 1–9). 2-Ethynylthiophene also participated in the reaction and gave 1,4-diary-1,2,3-triazole 8j in high yield. Under the same reaction conditions, aliphatic alkyne showed less efficiency (Table 4, entry 14). In addition, reaction yields were good for substituted aromatic boronic acids, regardless the electronic nature and substitution pattern on the aryl moiety.
Table 4 Scope of reaction of aryl boronic acid with alkynes and sodium azide catalyzed by magnetic NiFe2O4–glutamate–Cu

|
Entry |
R1 |
Alkyne |
Product |
Time (h) |
Yield (%) |
M.p. (°C) |
1 |
H |
 |
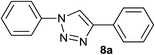 |
3.0 |
95 |
176–178, 175–177 (ref. 27) |
2 |
H |
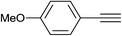 |
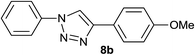 |
5.0 |
88 |
157–158, 152.5–154.5 (ref. 42) |
3 |
H |
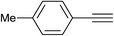 |
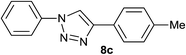 |
5.0 |
90 |
175–176, 173–174 (ref. 5g) |
4 |
H |
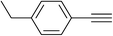 |
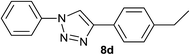 |
5.0 |
89 |
132–133, 128–130 (ref. 43) |
5 |
H |
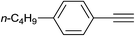 |
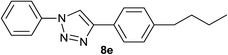 |
5.5 |
86 |
120–121, 118–121 (ref. 44) |
6 |
H |
 |
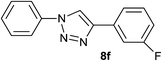 |
6.0 |
85 |
185–186 (ref. 45) |
7 |
H |
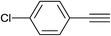 |
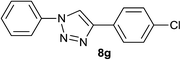 |
4.0 |
92 |
210–211, 207–209 (ref. 43) |
8 |
H |
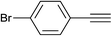 |
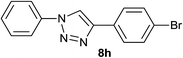 |
5.5 |
89 |
215–216, 212–214 (ref. 43) |
9 |
H |
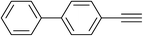 |
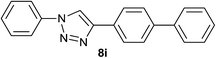 |
6.0 |
82 |
233–234 (ref. 46) |
10 |
H |
 |
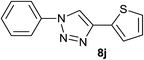 |
4.0 |
90 |
132–133, 127–129 (ref. 43) |
11 |
4-Me |
 |
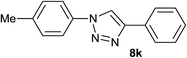 |
4.0 |
94 |
168–169, 165–167 (ref. 41) |
12 |
3-F |
 |
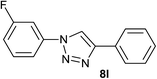 |
4.0 |
94 |
181–182 |
13 |
4-Cl |
 |
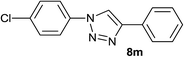 |
5.0 |
92 |
185–186, 224–226 (ref. 43) |
14 |
H |
 |
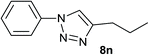 |
4.0 |
65 |
Oil2d |
These results successfully demonstrated that this catalytic system can be readily applied to the combinatorial click synthesis of 1,4-disubstituted-1,2,3-triazoles.
Conclusion
In summary, magnetic nano NiFe2O4 supported glutamate–copper catalyst has been successfully prepared by a relatively simple route. It has been demonstrated to be an efficient catalyst for one-pot synthesis of a wide variety of 1,4-disubstituted-1,2,3-triazoles via three-component click reactions of sodium azide, non-activated terminal alkynes, and different azide precursors such as epoxides, benzyl chloride, and aryl boronic acids in water at room temperature. Aqueous reaction medium, ambient reaction condition, high yields, wide substrate scope, easy separation of catalyst using an external magnet, efficient recycling are outstanding features of this protocol that make it to be an important addition to the existing methods for synthesis of 1,4-disubstituted-1,2,3-triazoles.
Experimental section
General information
The IR spectra were obtained as KBr pellets or liquid films on KBr pellets with a Bruker-TENSOR 27 spectrometer. XRD measurement was performed on a PANalytical X'Pert Pro X-ray diffractometer using Cu-Kα radiation as the X-ray source in the 2θ range of 20–80°. Transmission electron microscope (TEM) observation was performed by using a Hitachi H-7650 microscope at 80 kV. The morphology of the magnetic nanoparticles was observed by a Hitachi S-4800 SEM instrument. Melting points were determined using an X-4 digital melting point apparatus and are uncorrected. NMR spectra were recorded with a Bruker DRX-500 spectrometer at 500 MHz for 1H NMR and 125 MHz for 13C NMR using CDCl3 as the solvent and TMS as an internal standard. Mass spectra were performed on a ThermoFinnigan LCQ Advantage instrument with an ESI source (4.5 keV).
Preparation of NiFe2O4 magnetic nanoparticles
To a mixture of FeCl3 (2.7 g) and NiCl2 (1.2 g) in 50 mL water, 25 mL NaOH (1.5 mol L−1) and oleic was added slowly. The mixture was stirred for 1 h at 80 °C. The obtained precipitated nanoparticles were separated magnetically, washed with water and ethanol until the pH reached 7, and dried under vacuum at 60 °C for 2 h.
Surface modification of nano-NiFe2O4
The obtained nano NiFe2O4 (0.5 g) was dispersed in a mixture of water and methanol (20 mL, 3
:
1 volume), and sonicated for 15 min at room temperature. Then, glutamate (0.3 g) dissolved in water (5 mL) was added, and the resulting mixture was stirred for 2 h. The prepared glutamate functionalized magnetic nanoparticles were separated by magnetic decantation, and then washed with water and ethanol, and dried under vacuum at 60 °C for 2 h.
Immobilization of Cu on magnetic nanoparticles
Glutamate-functionalized nano-NiFe2O4 (1 g) was ultrasonically dispersed in a mixture of water and methanol (10 mL, 1
:
1). Cu(OAc)2 (0.1 g) solution in water (5 mL) was added to the reaction mixture. Hydrazine monohydrate solution in water was added drop wise to adjust the pH of this mixture to 9.0, followed by the addition of 0.1 g of NaBH4. The reaction mixture was then stirred for 24 h at room temperature. The catalyst was harvested with the aid of a magnet, washed several times with water and ethanol, and dried under vacuum at 60 °C for 2 h.
General procedure for synthesis of 1,2,3-triazoles in water
NaN3 (1.1 mmol), alkyne (1 mmol), epoxide or benzyl chloride, or phenylboronic acid (1 mmol), were added to a suspension of NiFe2O4–glutamate–Cu (1.0 mol% Cu, 6.3 mg NiFe2O4–glutamate–Cu) in H2O (2 mL). The reaction mixture was stirred at room temperature and monitored by TLC. After completion of the reaction, the catalyst was easily removed from reaction mixture using an external magnet. Then, water (30 mL) was added to the resulting mixture, followed by extraction with EtOAc (3 × 10 mL). The organic layer was dried with MgSO4, concentrated under reduced pressure. The crude product was purified by flash silica gel column chromatography eluting with petroleum ether–ethyl acetate to afford the desired products.
Characterization data
2-Phenyl-2-(4-phenyl-1H-1,2,3-triazol-1-yl)ethanol (4a). White solid; IR (KBr): 3393, 3095, 2928, 1458, 1429, 1223, 1157, 1074, 1051, 1030, 762, 696 cm−1; 1H NMR (CDCl3, 500 MHz) δ 3.20–3.18 (m, 1H), 4.23 (ddd, J = 3.5 Hz, J = 9.0 Hz, J = 12.5 Hz, 1H), 4.64 (ddd, J = 5.0 Hz, J = 8.0 Hz, J = 12.5 Hz, 1H), 5.67 (dd, J = 4.0 Hz, J = 8.5 Hz, 1H), 7.27–7.26 (m, 2H), 7.33 (t, J = 7.5 Hz, 1H), 7.42–7.38 (m, 5H), 7.69 (s, 1H), 7.70 (d, J = 7.0 Hz, 2H) ppm; 13C NMR (CDCl3, 125 MHz) δ 65.1, 67.3, 120.6, 125.7, 127.2, 128.3, 128.8, 129.0, 129.2, 130.2, 136.1, 147.7 ppm. ESI-MS: m/z = 266 (M + 1)+.
2-(4-(4-Methoxyphenyl)-1H-1,2,3-triazol-1-yl)-2-phenylethanol (4b). White solid; IR (KBr): 3396, 3095, 2929, 1406, 1417, 1255, 1176, 1074, 1051, 1090, 756, 696 cm−1; 1H NMR (CDCl3, 500 MHz) δ 3.15 (dd, J = 5.5 Hz, J = 9.5 Hz, 1H), 3.84 (s, 3H), 4.22 (ddd, J = 4.0 Hz, J = 9.0 Hz, J = 13.0 Hz, 1H), 4.63 (ddd, J = 5.5 Hz, J = 8.5 Hz, J = 13.0 Hz, 1H), 5.65 (dd, J = 3.5 Hz, J = 8.0 Hz, 1H), 6.94 (d, J = 8.5 Hz, 2H), 7.24–7.26 (m, 2H), 7.42–7.38 (m, 3H), 7.60 (s, 1H), 7.72 (d, J = 8.5 Hz, 2H) ppm; 13C NMR (CDCl3, 125 MHz) δ 55.3, 65.2, 67.3, 114.2, 119.8, 123.0, 127.0, 127.2, 129.0, 129.2, 136.2, 147.6, 159.7 ppm. ESI-MS: m/z = 296 (M + 1)+; HRMS calcd for C17H18N3O2 (M + H)+: 296.1394; found: 296.1397.
2-Phenyl-2-(4-(m-tolyl)-1H-1,2,3-triazol-1-yl)ethanol (4c). White solid; IR (KBr): 3419, 3086, 2924, 1491, 1417, 1219, 1136, 1087, 1051, 1028, 775, 698 cm−1; 1H NMR (CDCl3, 500 MHz) δ 2.38 (s, 3H), 3.15 (dd, J = 5.5 Hz, J = 9.0 Hz, 1H), 4.22 (ddd, J = 4.0 Hz, J = 9.0 Hz, J = 13.0 Hz, 1H), 4.63 (ddd, J = 5.5 Hz, J = 8.5 Hz, J = 13.0 Hz, 1H), 5.67 (dd, J = 4.0 Hz, J = 8.5 Hz, 1H), 7.14 (d, J = 7.5 Hz, 1H), 7.24 (d, J = 2.0 Hz, 2H), 7.29 (t, J = 7.5 Hz, 1H), 7.42–7.38 (m, 3H), 7.57 (d, J = 7.5 Hz, 1H), 7.64 (s, 1H), 7.68 (s, 1H) ppm; 13C NMR (CDCl3, 125 MHz) δ 21.4, 65.1, 67.3, 120.5, 122.8, 126.4, 127.2, 128.7, 129.0, 129.1, 129.2, 130.1, 136.1, 138.5, 147.8 ppm. Anal. calcd for C17H17N3O: C, 73.10; H, 6.13; N, 15.04. Found: C, 73.25; H, 6.27; N, 15.06. ESI-MS: m/z = 280 (M + 1)+.
2-Phenyl-2-(4-(p-tolyl)-1H-1,2,3-triazol-1-yl)ethanol (4d). White solid; IR (KBr): 3385, 3093, 2930, 1456, 1221, 1182, 1074, 1049, 756, 696 cm−1; 1H NMR (CDCl3, 500 MHz) δ 2.37 (s, 3H), 4.22 (dd, J = 3.5 Hz, J = 12.5 Hz, 1H), 4.63 (dd, J = 8.5 Hz, J = 12.5 Hz, 1H), 5.66 (dd, J = 3.5 Hz, J = 8.0 Hz, 1H), 7.22 (d, J = 8.0 Hz, 2H), 7.25 (s, 2H), 7.39 (d, J = 6.0 Hz, 3H), 7.65 (s, 1H), 7.69 (d, J = 8.0 Hz, 2H) ppm; 13C NMR (CDCl3, 125 MHz) δ 21.3, 53.1, 65.3, 67.3, 120.2, 125.6, 127.1, 127.4, 129.0, 129.2, 129.5, 136.1, 138.2, 147.9 ppm. ESI-MS: m/z = 280 (M + 1)+.
2-(4-(4-Ethylphenyl)-1H-1,2,3-triazol-1-yl)-2-phenylethanol (4e). White solid; IR (KBr): 3392, 3080, 2928, 1489, 1417, 1220, 1116, 1074, 1049, 1030, 754, 696 cm−1; 1H NMR (CDCl3, 500 MHz) δ 1.26 (t, J = 3.5 Hz, 3H), 2.67 (q, J = 7.5 Hz, 2H), 3.17 (dd, J = 5.5 Hz, J = 9.5 Hz, 1H), 4.22 (ddd, J = 4.0 Hz, J = 9.0 Hz, J = 13.0 Hz, 1H), 4.63 (ddd, J = 5.5 Hz, J = 8.5 Hz, J = 13.0 Hz, 1H), 5.66 (dd, J = 4.0 Hz, J = 8.5 Hz, 1H), 7.25–7.23 (m, 4H), 7.42–7.38 (m, 3H), 7.65 (s, 1H), 7.71 (d, J = 8.0 Hz, 2H) ppm; 13C NMR (CDCl3, 125 MHz) δ 29.7, 32.0, 65.2, 67.3, 120.3, 125.7, 127.2, 128.3, 129.0, 129.1, 130.9, 136.2, 144.5, 147.8 ppm. ESI-MS: m/z = 294 (M + 1)+; HRMS calcd for C18H20N3O (M + H)+: 294.1601; found: 294.1604.
2-(4-(4-Butylphenyl)-1H-1,2,3-triazol-1-yl)-2-phenylethanol (4f). White solid; IR (KBr): 3385, 3080, 2928, 1458, 1437, 1225, 1184, 1076, 1051, 750, 696 cm−1; 1H NMR (CDCl3, 500 MHz) δ 0.93 (t, J = 7.0 Hz, 3H), 1.36 (dd, J = 7.0 Hz, J = 14.5 Hz, 2H), 1.61 (t, J = 7.5 Hz, 2H), 2.62 (t, J = 8.0 Hz, 2H), 3.19 (s, 1H), 4.22 (dd, J = 3.5 Hz, J = 7.0 Hz, 1H), 4.62 (dd, J = 11.0 Hz, J = 19.5 Hz, 1H), 5.66 (dd, J = 3.0 Hz, J = 8.0 Hz, 1H), 7.22 (d, J = 8.0 Hz, 4H), 7.38 (s, 3H), 7.65 (s, 1H), 7.70 (d, J = 8.0 Hz, 2H) ppm; 13C NMR (CDCl3, 125 MHz) δ 13.9, 22.3, 33.5, 35.4, 65.3, 67.3, 120.2, 125.6, 127.1, 127.6, 128.9, 129.0, 129.2, 136.1, 143.3, 147.9 ppm. ESI-MS: m/z = 322 (M + 1)+; HRMS calcd for C20H24N3O (M + H)+: 322.1914; found: 322.1916.
2-(4-(4-Hexylphenyl)-1H-1,2,3-triazol-1-yl)-2-phenylethanol (4g). White solid; IR (KBr): 3392, 3080, 2918, 1491, 1411, 1224, 1120, 1074, 1051, 1030, 752, 696 cm−1; 1H NMR (CDCl3, 500 MHz) δ 0.88 (t, J = 6.5 Hz, 3H), 1.30 (s, 5H), 1.60 (dd, J = 6.5 Hz, J = 14.5 Hz, 3H), 2.62 (t, J = 7.5 Hz, 2H), 3.27 (s, 1H), 4.22 (dd, J = 12.5 Hz, 1H), 4.63 (dd, J = 8.0 Hz, J = 12.0 Hz, 1H), 5.66 (dd, J = 3.5 Hz, J = 8.0 Hz, 1H), 7.25–7.21 (m, 4H), 7.39 (d, J = 6.0 Hz, 3H), 7.65 (s, 1H), 7.69 (d, J = 8.0 Hz, 2H) ppm; 13C NMR (CDCl3, 125 MHz) δ 14.1, 22.6, 29.0, 31.4, 31.7, 35.8, 65.2, 67.3, 120.3, 125.6, 127.2, 127.6, 128.9, 129.0, 129.1, 136.2, 143.3, 147.8 ppm. ESI-MS: m/z = 350 (M + 1)+; HRMS calcd for C22H28N3O (M + H)+: 350.2227; found: 350.2232.
2-(4-(3-Fluorophenyl)-1H-1,2,3-triazol-1-yl)-2-phenylethanol (4h). White solid; IR (KBr): 3385, 3082, 2924, 1501, 1437, 1226, 1132, 1072, 1051, 779, 675 cm−1; 1H NMR (CDCl3, 500 MHz) δ 3.12 (s, 1H), 4.23 (d, J = 12.0 Hz, 1H), 4.64 (dd, J = 8.5 Hz, J = 12.0 Hz, 1H), 5.68 (dd, J = 3.5 Hz, J = 8.0 Hz, 1H), 7.02 (t, J = 8.5 Hz, 1H), 7.27 (d, J = 2.5 Hz, 2H), 7.43–7.34 (m, 4H), 7.53–7.50 (m, 1H), 7.55 (d, J = 7.5 Hz, 1H), 7.71 (s, 1H) ppm; 13C NMR (CDCl3, 125 MHz) δ 60.2, 62.7, 107.7, 107.9, 110.3 (d, 2JCF = 21.1 Hz), 116.3, 122.5, 124.3, 124.4, 125.7 (d, 4JCF = 8.4 Hz), 127.6 (d, 3JCF = 8.1 Hz), 131.2, 141.8, 158.4 (d, 1JCF = 244.0 Hz) ppm. ESI-MS: m/z = 284 (M + 1)+.
2-(4-(4-Fluorophenyl)-1H-1,2,3-triazol-1-yl)-2-phenylethanol (4i). White solid; IR (KBr): 3383, 3095, 2932, 1456, 1236, 1180, 1157, 1074, 1053, 1028, 752, 696 cm−1; 1H NMR (CDCl3, 500 MHz) δ 4.23 (dd, J = 3.5 Hz, J = 12.5 Hz, 1H), 4.64 (dd, J = 8.5 Hz, J = 12.5 Hz, 1H), 5.67 (dd, J = 3.5 Hz, J = 8.0 Hz, 1H), 7.10 (t, J = 8.5 Hz, 2H), 7.26 (s, 2H), 7.40 (d, J = 6.0 Hz, 3H), 7.66 (s, 1H), 7.76 (dd, J = 6.0 Hz, J = 8.0 Hz, 2H) ppm; 13C NMR (CDCl3, 125 MHz) δ 65.0, 67.4, 115.8 (d, 2JCF = 21.8 Hz), 120.4, 126.4 (d, 4JCF = 3.3 Hz), 127.2, 127.4 (d, 3JCF = 8.0 Hz), 129.0, 129.2, 136.0, 146.7, 162.8 (d, 1JCF = 271.1 Hz) ppm. ESI-MS: m/z = 284 (M + 1)+; HRMS calcd for C16H15FN3O (M + H)+: 284.1194; found: 284.1195.
2-(4-(4-Chlorophenyl)-1H-1,2,3-triazol-1-yl)-2-phenylethanol (4j). White solid; IR (KBr): 3383, 3086, 2929, 1454, 1226, 1186, 1076, 1049, 1028, 756, 715, 696 cm−1; 1H NMR (CDCl3, 500 MHz) δ 3.15–3.12 (m, 1H), 4.23 (ddd, J = 3.0 Hz, J = 12.0 Hz, J = 21.5 Hz, 1H), 4.64 (ddd, J = 6.0 Hz, J = 12.5 Hz, J = 18.5 Hz, 1H), 5.67 (dd, J = 3.5 Hz, J = 8.0 Hz, 1H), 7.26 (s, 2H), 7.40–7.37 (m, 5H), 7.69 (s, 1H), 7.72 (d, J = 8.5 Hz, 2H) ppm; 13C NMR (CDCl3, 125 MHz) δ 65.1, 67.4, 120.6, 126.9, 127.2, 128.7, 129.0, 129.1, 129.2, 134.1, 135.9, 146.6 ppm. ESI-MS: m/z = 300 (M + 1)+; HRMS calcd for C16H15ClN3O (M + H)+: 300.0898; found: 300.0894.
2-(4-(4-Bromophenyl)-1H-1,2,3-triazol-1-yl)-2-phenylethanol (4k). White solid; IR (KBr): 3383, 3086, 2970, 1470, 1456, 1228, 1072, 1049, 821, 756 cm−1; 1H NMR (CDCl3, 500 MHz) δ 3.13 (dd, J = 5.5 Hz, J = 9.0 Hz, 1H), 4.23 (ddd, J = 4.0 Hz, J = 9.0 Hz, J = 12.5 Hz, 1H), 4.64 (ddd, J = 5.5 Hz, J = 8.0 Hz, J = 13.0 Hz, 1H), 5.67 (dd, J = 3.5 Hz, J = 8.0 Hz, 1H), 7.27 (s, 2H), 7.42–7.37 (m, 3H), 7.53 (d, J = 8.5 Hz, 2H), 7.66 (d, J = 8.0 Hz, 2H), 7.70 (s, 1H) ppm; 13C NMR (CDCl3, 125 MHz) δ 65.1, 67.4, 120.7, 122.2, 127.2, 128.9, 129.1, 129.2, 130.9, 132.0, 135.9, 146.7 ppm. ESI-MS: m/z = 344 (M + 1)+; HRMS calcd for C16H15BrN3O (M + H)+: 344.0393; found: 344.0398.
2-Phenyl-2-(4-(pyridin-2-yl)-1H-1,2,3-triazol-1-yl)ethanol (4l). White solid; IR (KBr): 3392, 3082, 2953, 1475, 1423, 1234, 1179, 1076, 1058, 1039, 825, 786, 605 cm−1; 1H NMR (CDCl3, 500 MHz) δ 3.37 (s, 1H), 4.24 (d, J = 11.0 Hz, 1H), 4.63 (t, J = 10.5 Hz, 1H), 5.71 (dd, J = 3.5 Hz, J = 8.0 Hz, 1H), 7.22 (t, J = 6.5 Hz, 1H), 7.28 (d, J = 7.5 Hz, 2H), 7.38 (d, J = 6.5 Hz, 3H), 7.78 (t, J = 8.0 Hz, 1H), 8.11 (s, 1H), 8.17 (d, J = 7.5 Hz, 1H), 8.52 (d, J = 4.5 Hz, 1H) ppm; 13C NMR (CDCl3, 125 MHz) δ 65.0, 67.4, 120.3, 122.9, 123.0, 127.3, 129.0, 129.1, 135.9, 137.1, 147.9, 149.1, 149.9 ppm. ESI-MS: m/z = 267 (M + 1)+.
2-Phenyl-2-(4-propyl-1H-1,2,3-triazol-1-yl)ethanol (4m). Oil; IR (KBr): 3414, 2929, 1460, 1419, 1055, 653, 538 cm−1; 1H NMR (CDCl3, 500 MHz) δ 0.95 (t, J = 7.5 Hz, 3H), 1.77–1.67 (m, 2H), 2.68 (t, J = 7.5 Hz, 2H), 2.88–2.86 (m, 1H), 4.18 (ddd, J = 4.0 Hz, J = 8.5 Hz, J = 12.5 Hz, 1H), 4.61 (ddd, J = 6.0 Hz, J = 8.5 Hz, J = 12.5 Hz, 1H), 5.65 (dd, J = 4.5 Hz, J = 9.0 Hz, 1H), 7.18 (d, J = 8.0 Hz, 2H), 7.35–7.31 (m, 4H) ppm; 13C NMR (CDCl3, 125 MHz) δ 13.7, 22.0, 25.4, 27.4, 65.0, 65.8, 120.3, 127.0, 128.4, 128.7, 136.4, 150.1 ppm. ESI-MS: m/z = 232 (M + 1)+.
2-(4-Pentyl-1H-1,2,3-triazol-1-yl)-2-phenylethanol (4n). Oil; IR (KBr): 3414, 3090, 2930, 1492, 1435, 1209, 1109, 1068, 752, 600 cm−1; 1H NMR (CDCl3, 500 MHz) δ 0.91–0.87 (m, 3H), 1.54–1.48 (m, 2H), 1.74–1.68 (m, 2H), 2.36 (t, J = 7.0 Hz, 2H), 2.69 (t, J = 7.5 Hz, 2H), 4.19–4.16 (m, 1H), 4.59 (dd, J = 8.5 Hz, J = 12.0 Hz, 1H), 5.65 (dd, J = 4.0 Hz, J = 8.5 Hz, 1H), 7.19 (d, J = 7.0 Hz, 2H), 7.35–7.27 (m, 4H) ppm; 13C NMR (CDCl3, 125 MHz) δ 14.0, 22.3, 25.4, 27.8, 30.9, 64.9, 65.8, 120.3, 127.0, 128.5, 128.8, 136.3, 150.3 ppm. ESI-MS: m/z = 260 (M + 1)+; HRMS calcd for C15H21N3O (M + H)+: 260.1767; found: 260.1763.
2-(4-Phenyl-1H-1,2,3-triazol-1-yl)-2-(p-tolyl)ethanol (4o). White solid; IR (KBr): 3385, 3088, 2928, 1511, 1419, 1224, 1155, 1087, 1049, 767, 692 cm−1; 1H NMR (CDCl3, 500 MHz) δ 2.36 (s, 3H), 3.13–3.10 (m, 1H), 4.19 (ddd, J = 3.5 Hz, J = 8.5 Hz, J = 12.5 Hz, 1H), 4.62 (ddd, J = 4.5 Hz, J = 9.0 Hz, J = 13.0 Hz, 1H), 5.63 (dd, J = 4.0 Hz, J = 8.5 Hz, 1H), 7.15 (d, J = 8.0 Hz, 2H), 7.20 (d, J = 8.0 Hz, 2H), 7.33 (t, J = 7.0 Hz, 1H), 7.41 (t, J = 7.5 Hz, 2H), 7.67 (s, 1H), 7.79 (d, J = 7.5 Hz, 2H) ppm; 13C NMR (CDCl3, 125 MHz) δ 16.4, 60.5, 62.4, 115.7, 120.9, 122.4, 123.5, 124.1, 125.1, 125.5, 128.3, 134.3, 142.9 ppm. ESI-MS: m/z = 280 (M + 1)+.
2-(4-Chlorophenyl)-2-(4-phenyl-1H-1,2,3-triazol-1-yl)ethanol (4p). White solid; IR (KBr): 3392, 3086, 2935, 1491, 1224, 1157, 1074, 1051, 765, 692 cm−1; 1H NMR (CDCl3, 500 MHz) δ 3.14 (s, 1H), 4.24 (d, J = 11.5 Hz, 1H), 4.59 (dd, J = 8.0 Hz, J = 12.0 Hz, 1H), 5.65 (dd, J = 4.0 Hz, J = 8.0 Hz, 1H), 7.22 (d, J = 8.0 Hz, 2H), 7.38–7.32 (m, 3H), 7.41 (t, J = 7.5 Hz, 2H), 7.70 (s, 1H), 7.79 (d, J = 8.0 Hz, 2H) ppm; 13C NMR (CDCl3, 125 MHz) δ 65.0, 66.6, 120.5, 125.7, 128.4, 128.6, 128.9, 129.4, 130.1, 134.6, 135.1, 147.9 ppm. ESI-MS: m/z = 300 (M + 1)+.
2-(4-Bromophenyl)-2-(4-phenyl-1H-1,2,3-triazol-1-yl)ethanol (4q). White solid; IR (KBr): 3392, 3082, 2924, 1521, 1419, 1222, 1120, 1074, 1049, 765, 692 cm−1; 1H NMR (CDCl3, 500 MHz) δ 3.09 (s, 1H), 4.24 (dd, J = 2.5 Hz, J = 11.5 Hz, 1H), 4.59 (dd, J = 8.0 Hz, J = 12.0 Hz, 1H), 5.63 (dd, J = 3.5 Hz, J = 8.0 Hz, 1H), 7.15 (d, J = 8.5 Hz, 2H), 7.34 (t, J = 7.5 Hz, 1H), 7.42 (t, J = 7.5 Hz, 2H), 7.53 (d, J = 8.5 Hz, 2H) 7.70 (s, 1H), 7.79 (d, J = 7.5 Hz, 2H) ppm; 13C NMR (CDCl3, 125 MHz) δ 65.0, 66.6, 120.5, 123.2, 125.7, 128.4, 128.9, 130.1, 130.9, 132.4, 135.1, 148.0 ppm. ESI-MS: m/z = 344 (M + 1)+; HRMS calcd for C16H15BrN3O (M + H)+: 344.0393; found: 344.0399.
2-(4-Chlorophenyl)-2-(4-(4-fluorophenyl)-1H-1,2,3-triazol-1-yl)ethanol (4r). White solid; IR (KBr): 3383, 3091, 2928, 1456, 1234, 1157, 1074, 1049, 705 cm−1; 1H NMR (CDCl3, 500 MHz) δ 3.11 (s, 1H), 4.23 (dd, J = 5.0 Hz, J = 11.0 Hz, 1H), 4.59 (t, J = 10.0 Hz, 1H), 5.64 (dd, J = 4.0 Hz, J = 8.0 Hz, 1H), 7.10 (t, J = 9.0 Hz, 2H), 7.22 (d, J = 8.5 Hz, 2H), 7.37 (d, J = 8.5 Hz, 2H), 7.66 (s, 1H), 7.75 (d, J = 5.0 Hz, 1H), 7.77 (d, J = 5.5 Hz, 1H) ppm; 13C NMR (CDCl3, 125 MHz) δ 64.9, 66.6, 115.8 (d, 2JCF = 21.8 Hz), 120.3, 126.3 (d, 4JCF = 3.4 Hz), 127.4 (d, 3JCF = 8.0 Hz), 128.6, 129.4, 134.5, 135.1, 146.9, 162.8 (d, 1JCF = 246.3 Hz) ppm. ESI-MS: m/z = 318 (M + 1)+; HRMS calcd for C16H14ClFN3O (M + H)+: 318.0804; found: 318.0808.
1-Phenoxy-3-(4-phenyl-1H-1,2,3-triazol-1-yl)propan-2-ol (4s). Oil; IR (KBr): 3086, 2924, 1458, 1236, 1176, 1078, 1043, 763, 692 cm−1; 1H NMR (CDCl3, 500 MHz) δ 4.00 (dd, J = 6.0 Hz, J = 9.5 Hz, 1H), 4.06 (dd, J = 5.0 Hz, J = 9.5 Hz, 1H), 4.53 (s, 1H), 4.61 (dd, J = 7.0 Hz, J = 14.0 Hz, 1H), 4.75 (dd, J = 3.0 Hz, J = 14.0 Hz, 1H), 6.93 (d, J = 8.5 Hz, 2H), 7.01 (t, J = 7.5 Hz, 1H), 7.32 (t, J = 8.0 Hz, 2H), 7.36 (d, J = 7.5 Hz, 1H), 7.43 (t, J = 7.5 Hz, 2H), 7.81 (d, J = 8.0 Hz, 2H), 7.91 (s, 1H) ppm; 13C NMR (CDCl3, 125 MHz) δ 29.7, 53.3, 68.9, 114.6, 121.4, 121.6, 125.6, 128.2, 128.8, 129.7, 130.2, 147.5, 158.2 ppm. ESI-MS: m/z = 296 (M + 1)+.
1-(4-Methoxyphenoxy)-3-(4-phenyl-1H-1,2,3-triazol-1-yl)propan-2-ol (4t). White solid; IR (KBr): 3421, 3086, 2926, 1508, 1458, 1288, 1122, 1076, 1031, 769, 696 cm−1; 1H NMR (CDCl3, 500 MHz) δ 3.19 (d, J = 4.5 Hz, 1H), 3.77 (s, 3H), 3.93 (dd, J = 6.0 Hz, J = 9.5 Hz, 1H), 3.99 (dd, J = 5.0 Hz, J = 9.5 Hz, 1H), 4.49 (s, 1H), 4.58 (dd, J = 7.0 Hz, J = 14.5 Hz, 1H), 4.72 (dd, J = 3.0 Hz, J = 14.0 Hz, 1H), 6.84 (t, J = 10.5 Hz, 4H), 7.33 (t, J = 8.5 Hz, 1H), 7.41 (t, J = 7.5 Hz, 2H), 7.79 (d, J = 7.5 Hz, 2H), 7.89 (s, 1H) ppm; 13C NMR (CDCl3, 125 MHz) δ 29.7, 55.7, 69.0, 69.7, 114.8, 115.6, 121.3, 125.6, 128.2, 128.8, 130.3, 147.6, 152.3, 154.4 ppm. ESI-MS: m/z = 326 (M + 1)+.
1-(4-Phenyl-1H-1,2,3-triazol-1-yl)-3-(o-tolyloxy)propan-2-ol (4u). Oil; IR (KBr): 3392, 3120, 2926, 1458, 1244, 1188, 1078, 1028, 750, 696 cm−1; 1H NMR (CDCl3, 500 MHz) δ 2.27 (s, 3H), 3.28 (d, J = 3.5 Hz, 1H), 4.03 (ddd, J = 5.0 Hz, J = 9.5 Hz, J = 14.5 Hz, 2H), 4.61–4.55 (m, 2H), 4.76 (dd, J = 2.0 Hz, J = 13.5 Hz, 1H), 6.80 (d, J = 8.0 Hz, 1H), 6.91 (t, J = 7.5 Hz, 1H), 7.15 (t, J = 7.5 Hz, 2H), 7.33 (t, J = 7.5 Hz, 1H), 7.41 (t, J = 8.0 Hz, 2H), 7.78 (d, J = 8.0 Hz, 2H), 7.89 (s, 1H) ppm; 13C NMR (CDCl3, 125 MHz) δ 29.7, 53.1, 68.9, 69.2, 111.3, 121.2, 121.4, 125.7, 126.6, 127.1, 128.2, 128.9, 130.4, 131.0, 147.8, 156.1 ppm. ESI-MS: m/z = 310 (M + 1)+.
1-Butoxy-3-(4-phenyl-1H-1,2,3-triazol-1-yl)propan-2-ol (4v). Oil; IR (KBr): 3419, 3103, 2928, 1458, 1431, 1228, 1130, 1070, 767, 696 cm−1; 1H NMR (CDCl3, 500 MHz) δ 0.92 (t, J = 7.5 Hz, 3H), 1.37 (dd, J = 7.5 Hz, J = 15.0 Hz, 2H), 1.59–1.54 (m, 2H), 3.40 (dd, J = 5.5 Hz, J = 9.5 Hz, 1H), 3.49 (ddd, J = 4.5 Hz, J = 9.5 Hz, J = 14.0 Hz, 3H), 4.25 (t, J = 10.0 Hz, 1H), 4.43 (dd, J = 7.0 Hz, J = 14.0 Hz, 1H), 4.59 (dd, J = 3.5 Hz, J = 14.0 Hz, 1H), 7.32 (t, J = 7.5 Hz, 1H), 7.40 (t, J = 7.5 Hz, 2H), 7.78 (d, J = 8.0 Hz, 2H), 7.88 (s, 1H) ppm; 13C NMR (CDCl3, 125 MHz) δ 13.9, 19.3, 31.6, 53.2, 69.2, 71.5, 71.6, 121.2, 125.6, 128.1, 128.8, 130.4, 147.5 ppm. ESI-MS: m/z = 276 (M + 1)+; HRMS calcd for C15H22N3O2 (M + H)+: 276.1707; found: 276.1711.
1-(Allyloxy)-3-(4-phenyl-1H-1,2,3-triazol-1-yl)propan-2-ol (4w). White solid; IR (KBr): 3385, 3010, 2930, 1471, 1350, 1136, 1089, 981, 871, 767, 696 cm−1; 1H NMR (CDCl3, 500 MHz) δ 3.00 (d, J = 4.5 Hz, 1H), 3.41 (dd, J = 6.0 Hz, J = 9.5 Hz, 1H), 3.54 (dd, J = 4.5 Hz, J = 9.5 Hz, 1H), 4.03 (d, J = 5.5 Hz, 2H), 4.27 (d, J = 4.0 Hz, 1H), 4.47 (dd, J = 7.0 Hz, J = 14.0 Hz, 1H), 4.61 (dd, J = 3.5 Hz, J = 14.0 Hz, 1H), 5.23 (d, J = 10.5 Hz, 1H), 5.29 (d, J = 17.0 Hz, 1H), 5.90 (ddd, J = 5.5 Hz, J = 10.5 Hz, J = 16.0 Hz, 1H), 7.34 (t, J = 7.0 Hz, 1H), 7.43 (t, J = 7.5 Hz, 2H), 7.82 (d, J = 8.0 Hz, 2H), 7.91 (s, 1H) ppm; 13C NMR (CDCl3, 125 MHz) δ 53.2, 69.2, 71.0, 72.5, 117.8, 121.3, 125.6, 128.1, 128.8, 130.4, 134.1, 147.5 ppm. ESI-MS: m/z = 260 (M + 1)+.
1-(Allyloxy)-3-(4-(3-fluorophenyl)-1H-1,2,3-triazol-1-yl)propan-2-ol (4x). Oil; IR (KBr): 3392, 2924, 1471, 1230, 1118, 1074, 1055, 999, 785, 688 cm−1; 1H NMR (CDCl3, 500 MHz) δ 3.48–3.45 (m, 1H), 3.55–3.52 (m, 1H), 3.72–3.68 (m, 1H), 4.03 (d, J = 5.5 Hz, 2H), 4.29 (s, 1H), 4.41 (ddd, J = 2.0 Hz, J = 7.5 Hz, J = 9.5 Hz, 1H), 4.60 (dd, J = 1.5 Hz, J = 13.5 Hz, 1H), 5.21 (d, J = 10.5 Hz, 1H), 5.28 (d, J = 17.0 Hz, 1H), 5.90 (ddd, J = 5.5 Hz, J = 11.0 Hz, J = 16.5 Hz, 1H), 6.99 (t, J = 8.0 Hz, 1H), 7.35–7.31 (m, 1H), 7.43 (d, J = 10.0 Hz, 1H), 7.48 (d, J = 7.5 Hz, 1H), 7.91 (s, 1H) ppm; 13C NMR (CDCl3, 125 MHz) δ 24.9, 48.7, 53.5, 64.4, 66.4, 67.7, 107.7, 107.9, 110.1 (d, 2JCF = 21.1 Hz), 112.9, 117.1, 125.6 (d, 4JCF = 8.5 Hz), 127.7 (d, 3JCF = 8.6 Hz), 158.3 (d, 1JCF = 244.2 Hz) ppm. ESI-MS: m/z = 278 (M + 1)+; HRMS calcd for C14H17FN3O2 (M + H)+: 278.1299; found: 278.1303.
2-(4-Phenyl-1H-1,2,3-triazol-1-yl)cyclopentanol (4y). Oil; IR (KBr): 3304, 3093, 2928, 1456, 1232, 1074, 1055, 1028, 769, 696 cm−1; 1H NMR (CDCl3, 500 MHz) δ 1.81 (ddd, J = 8.0 Hz, J = 14.0 Hz, J = 16.0 Hz, 1H), 1.96–1.90 (m, 2H), 2.21 (ddd, J = 6.0 Hz, J = 13.0 Hz, J = 19.0 Hz, 2H), 2.38 (ddd, J = 7.0 Hz, J = 14.0 Hz, J = 20.5 Hz, 1H), 4.03 (s, 1H), 4.58 (ddd, J = 7.0 Hz, J = 14.0 Hz, J = 22.0 Hz, 2H), 7.31 (t, J = 7.0 Hz, 1H), 7.38 (t, J = 8.0 Hz, 2H), 7.68 (d, J = 4.5 Hz, 3H) ppm; 13C NMR (CDCl3, 125 MHz) δ 20.2, 29.4, 31.7, 68.1, 77.9, 119.1, 125.7, 128.2, 128.9, 130.5, 147.5 ppm. ESI-MS: m/z = 230 (M + 1)+; HRMS calcd for C13H16N3O (M + H)+: 230.1288; found: 230.1294.
2-(4-Phenyl-1H-1,2,3-triazol-1-yl)cyclohexanol (4z). White solid; IR (KBr): 3306, 3093, 2924, 1456, 1437, 1236, 1165, 1074, 1055, 1028, 767, 698 cm−1; 1H NMR (CDCl3, 500 MHz) δ 1.53–1.42 (m, 3H), 2.02–1.88 (m, 3H), 2.24 (d, J = 10.0 Hz, 2H), 3.21 (s, 1H), 4.09 (dd, J = 5.0 Hz, J = 9.5 Hz, 1H), 4.19 (ddd, J = 4.5 Hz, J = 10.0 Hz, J = 13.0 Hz, 1H), 7.32 (t, J = 7.5 Hz, 1H), 7.39 (t, J = 7.5 Hz, 2H), 7.75 (d, J = 7.5 Hz, 2H), 7.78 (s, 1H) ppm; 13C NMR (CDCl3, 125 MHz) δ 24.1, 24.8, 31.6, 33.8, 67.2, 72.6, 119.6, 125.5, 128.0, 128.8, 130.4, 146.9 ppm. ESI-MS: m/z = 244 (M + 1)+.
2-(4-(3-Fluorophenyl)-1H-1,2,3-triazol-1-yl)cyclohexanol (4aa). White solid; IR (KBr): 3317, 3095, 2926, 1458, 1419, 1240, 1199, 1087, 1053, 794, 692 cm−1; 1H NMR (CDCl3, 500 MHz) δ 1.53–1.41 (m, 3H), 2.03–1.88 (m, 3H), 2.24 (dd, J = 6.0 Hz, J = 9.0 Hz, 2H), 2.96 (d, J = 3.0 Hz, 1H), 4.07 (ddd, J = 5.5 Hz, J = 9.5 Hz, J = 13.5 Hz, 1H), 4.20 (ddd, J = 4.0 Hz, J = 9.5 Hz, J = 13.5 Hz, 1H), 7.02 (t, J = 8.5 Hz, 1H), 7.39–7.34 (m, 1H), 7.48 (d, J = 10.0 Hz, 1H) 7.54 (d, J = 7.5 Hz, 1H), 7.81 (s, 1H) ppm; 13C NMR (CDCl3, 125 MHz) δ 19.4, 20.1, 25.0, 26.7, 62.8, 67.8, 107.4, 107.6, 110.0 (d, 2JCF = 20.9 Hz), 115.6, 116.2, 125.5 (d, 4JCF = 8.4 Hz), 127.7 (d, 3JCF = 8.4 Hz), 158.3 (d, 1JCF = 244.1 Hz) ppm. ESI-MS: m/z = 262 (M + 1)+; HRMS calcd for C14H17FN3O (M + H)+: 262.1350; found: 262.1355.
1-(Oxiran-2-ylmethyl)-4-phenyl-1H-1,2,3-triazole (4ab). White solid; IR (KBr): 3392, 3094, 2926, 1458, 1417, 1220, 1176, 1074, 1049, 1026, 761, 696 cm−1; 1H NMR (CDCl3, 500 MHz) δ 2.56 (t, J = 2.0 Hz, 1H), 2.93 (t, J = 4.0 Hz, 1H), 3.42–3.40 (m, 1H), 4.37 (dd, J = 6.5 Hz, J = 15.0 Hz, 1H), 4.87 (d, J = 14.5 Hz, 1H), 7.34 (t, J = 7.5 Hz, 1H), 7.43 (t, J = 8.0 Hz, 2H), 7.85 (d, J = 8.0 Hz, 2H), 7.90 (s, 1H) ppm; 13C NMR (CDCl3, 125 MHz) δ 45.3, 50.1, 51.9, 120.5, 125.8, 128.3, 128.9, 130.4, 148.2 ppm. ESI-MS: m/z = 202 (M + 1)+.
1-(Oxiran-2-ylmethyl)-4-(p-tolyl)-1H-1,2,3-triazole (4ac). White solid; IR (KBr): 3398, 3080, 2922, 1506, 1419, 1220, 1174, 1072, 1047, 746, 663 cm−1; 1H NMR (CDCl3, 500 MHz) δ 2.38 (s, 3H), 2.58 (dd, J = 2.5 Hz, J = 4.5 Hz, 1H), 2.93 (t, J = 4.5 Hz, 1H), 3.41 (ddd, J = 3.0 Hz, J = 5.5 Hz, J = 9.0 Hz, 1H), 4.36 (dd, J = 6.5 Hz, J = 15.0 Hz, 1H), 4.86 (dd, J = 2.5 Hz, J = 14.5 Hz, 1H), 7.24 (d, J = 7.5 Hz, 2H), 7.73 (d, J = 8.5 Hz, 2H), 7.86 (s, 1H) ppm; 13C NMR (CDCl3, 125 MHz) δ 21.3, 45.3, 50.1, 51.9, 120.2, 125.7, 127.6, 129.6, 138.2, 148.3 ppm. ESI-MS: m/z = 216 (M + 1)+; HRMS calcd for C12H14N3O (M + H)+: 216.1131; found: 216.1133.
4-(4-Ethylphenyl)-1-(oxiran-2-ylmethyl)-1H-1,2,3-triazole (4ad). White solid; IR (KBr): 3400, 3066, 2928, 1521, 1220, 1072, 1047, 761, 677 cm−1; 1H NMR (CDCl3, 500 MHz) δ 1.25 (t, J = 4.0 Hz, 3H), 2.57 (t, J = 2.5 Hz, 1H), 2.68 (q, J = 7.5 Hz, J = 15.5 Hz, 2H), 2.93 (t, J = 4.0 Hz, 1H), 3.42–3.40 (m, 1H), 4.36 (dd, J = 6.0 Hz, J = 14.5 Hz, 1H), 4.86 (dd, J = 2.5 Hz, J = 14.5 Hz, 1H), 7.27 (d, J = 6.5 Hz, 2H), 7.76 (d, J = 8.0 Hz, 2H), 7.87 (s, 1H) ppm; 13C NMR (CDCl3, 125 MHz) δ 28.7, 29.7, 45.3, 50.1, 51.9, 120.2, 125.7, 127.8, 128.4, 144.5, 148.3 ppm. ESI-MS: m/z = 230 (M + 1)+; HRMS calcd for C13H16N3O (M + H)+: 230.1288; found: 230.1296.
4-(4-Butylphenyl)-1-(oxiran-2-ylmethyl)-1H-1,2,3-triazole (4ae). White solid; IR (KBr): 3404, 3064, 2924, 1450, 1419, 1220, 1072, 1049, 746 cm−1; 1H NMR (CDCl3, 500 MHz) δ 0.94 (t, J = 7.5 Hz, 3H), 1.41–1.33 (m, 2H), 1.65–1.59 (m, 2H), 2.57 (dd, J = 2.5 Hz, J = 4.5 Hz, 1H), 2.64 (t, J = 7.5 Hz, 2H), 2.93 (t, J = 4.5 Hz, 1H), 3.41 (ddd, J = 2.5 Hz, J = 4.0 Hz, J = 6.5 Hz, 1H), 4.36 (dd, J = 6.0 Hz, J = 14.5 Hz, 1H), 4.86 (dd, J = 2.5 Hz, J = 14.5 Hz, 1H), 7.25 (d, J = 8.0 Hz, 2H), 7.75 (d, J = 8.5 Hz, 2H), 7.86 (s, 1H) ppm; 13C NMR (CDCl3, 125 MHz) δ 14.0, 22.3, 33.5, 35.4, 45.3, 50.1, 51.8, 120.2, 125.7, 127.8, 128.9, 143.2, 148.3 ppm. ESI-MS: m/z = 258 (M + 1)+; HRMS calcd for C15H20N3O (M + H)+: 258.1601; found: 258.1605.
4-(4-Hexylphenyl)-1-(oxiran-2-ylmethyl)-1H-1,2,3-triazole (4af). White solid; IR (KBr): 3385, 3101, 2918, 1521, 1419, 1220, 1122, 1072, 1049, 821, 759 cm−1; 1H NMR (CDCl3, 500 MHz) δ 0.88 (t, J = 7.0 Hz, 3H), 1.36–1.29 (m, 6H), 1.63 (t, J = 7.0 Hz, 2H), 2.57 (dd, J = 2.5 Hz, J = 4.5 Hz, 1H), 2.63 (t, J = 8.0 Hz, 2H), 2.93 (t, J = 4.5 Hz, 1H), 3.41 (ddd, J = 2.5 Hz, J = 4.0 Hz, J = 6.5 Hz, 1H), 4.36 (dd, J = 6.5 Hz, J = 15.0 Hz, 1H), 4.86 (dd, J = 2.5 Hz, J = 14.5 Hz, 1H), 7.24 (d, J = 8.0 Hz, 2H), 7.75 (d, J = 8.0 Hz, 2H), 7.86 (s, 1H) ppm; 13C NMR (CDCl3, 125 MHz) δ 14.1, 22.6, 29.0, 31.4, 31.7, 35.7, 52.9, 63.7, 65.6, 121.2, 125.5, 127.5, 128.9, 143.2, 147.5 ppm. ESI-MS: m/z = 286 (M + 1)+; HRMS calcd for C17H24N3O (M + H)+: 286.1914; found: 286.1920.
4-(4-Fluorophenyl)-1-(oxiran-2-ylmethyl)-1H-1,2,3-triazole (4ag). White solid; IR (KBr): 3392, 3107, 2926, 1498, 1417, 1219, 1159, 1070, 1049, 759, 705 cm−1; 1H NMR (CDCl3, 500 MHz) δ 2.58 (dd, J = 2.5 Hz, J = 4.5 Hz, 1H), 2.94 (t, J = 4.0 Hz, 1H), 3.41 (ddd, J = 3.0 Hz, J = 6.5 Hz, J = 9.0 Hz, 1H), 4.35 (dd, J = 6.5 Hz, J = 15.0 Hz, 1H), 4.88 (dd, J = 2.5 Hz, J = 14.5 Hz, 1H), 7.13 (t, J = 8.5 Hz, 2H), 7.80 (d, J = 5.5 Hz, 1H), 7.82 (d, J = 5.5 Hz, 1H), 7.86 (s, 1H) ppm; 13C NMR (CDCl3, 125 MHz) δ 45.3, 50.0, 51.9, 115.8 (d, 2JCF = 21.6 Hz), 120.3, 126.7 (d, 4JCF = 3.0 Hz), 127.5 (d, 3JCF = 8.1 Hz), 147.3, 162.7 (d, 1JCF = 270.8 Hz) ppm. ESI-MS: m/z = 220 (M + 1)+; HRMS calcd for C11H11FN3O (M + H)+: 220.0881; found: 220.0879.
1-((2-Methyloxiran-2-yl)methyl)-4-phenyl-1H-1,2,3-triazole (4ah). Oil; IR (KBr): 3392, 3120, 2929, 1460, 1230, 1074, 1045, 1028, 765, 696 cm−1; 1H NMR (CDCl3, 500 MHz) δ 1.35 (s, 1H), 2.62 (d, J = 4.5 Hz, 1H), 2.74 (d, J = 4.5 Hz, 1H), 4.40 (d, J = 14.5 Hz, 1H), 4.70 (d, J = 15.0 Hz, 1H), 7.34 (t, J = 13.0 Hz, 1H), 7.43 (t, J = 7.5 Hz, 2H), 7.85 (d, J = 7.5 Hz, 2H), 7.90 (s, 1H) ppm; 13C NMR (CDCl3, 125 MHz) δ 18.6, 52.0, 55.1, 55.6, 120.5, 125.7, 128.2, 128.9, 130.5, 148.2 ppm. ESI-MS: m/z = 216 (M + 1)+; HRMS calcd for C12H14N3O (M + H)+: 216.1131; found: 216.1137.
1,3-Bis(4-phenyl-1H-1,2,3-triazol-1-yl)propan-2-ol (4ai)47. White solid, 252–253 °C; IR (KBr): 3383, 3093, 2924, 1458, 1227, 1159, 1084, 764, 692 cm−1; 1H NMR (DMSO-d6, 500 MHz) δ 4.47–4.39 (m, 3H), 4.64–4.60 (m, 2H), 5.80–5.78 (m, 1H), 7.33 (t, J = 7.5 Hz, 2H), 7.45 (t, J = 7.5 Hz, 4H), 7.85 (d, J = 7.0 Hz, 4H), 8.55 (s, 2H) ppm; 13C NMR (DMSO-d6, 125 MHz) δ 53.7, 68.8, 123.0, 125.6, 128.3, 129.4, 131.3, 146.6 ppm. ESI-MS: m/z = 347 (M + 1)+.
1-(4-(4-Ethylphenyl)-1H-1,2,3-triazol-1-yl)-3-(4-phenyl-1H-1,2,3-triazol-1-yl)propan-2-ol (4aj). Yellow solid, 219–220 °C; IR (KBr): 3385, 3088, 2928, 1464, 1226, 1157, 1082, 1028, 762, 691 cm−1; 1H NMR (DMSO-d6, 500 MHz) δ 1.20 (t, J = 7.5 Hz, 3H), 2.62 (q, J = 7.5 Hz, 2H), 4.42–4.40 (m, 3H), 4.63–4.60 (m, 2H), 5.78 (d, J = 4.5 Hz, 1H), 7.28 (d, J = 8.0 Hz, 2H), 7.33 (t, J = 7.0 Hz, 1H), 7.45 (t, J = 7.5 Hz, 2H), 7.76 (d, J = 8.0 Hz, 2H), 7.85 (d, J = 7.5 Hz, 2H), 8.49 (s, 1H), 8.54 (s, 1H) ppm; 13C NMR (DMSO-d6, 125 MHz) δ 16.0, 28.4, 53.67, 53.72, 68.8, 122.6, 123.0, 125.60, 125.63, 128.3, 128.7, 129.4, 131.3, 143.9, 146.60, 146.68 ppm. ESI-MS: m/z = 375 (M + 1)+; HRMS calcd for C21H23N6O (M + H)+: 375.1928; found: 375.1932.
1-Benzyl-4-phenyl-1H-1,2,3-triazole (6a). White solid; IR (KBr): 3198, 3032, 1616, 1508, 669, 489 cm−1; 1H NMR (CDCl3, 500 MHz) δ 5.59 (s, 2H), 7.33–7.31 (m, 3H), 7.42–7.37 (m, 5H), 7.66 (s, 1H), 7.80 (d, J = 8.0 Hz, 2H) ppm; 13C NMR (CDCl3, 125 MHz) δ 54.2, 119.5, 125.7, 128.1, 128.2, 128.8, 129.2, 130.6, 134.7, 148.3 ppm. ESI-MS: m/z = 236 (M + 1)+.
1-Benzyl-4-(4-ethylphenyl)-1H-1,2,3-triazole (6b). Oil; IR (KBr): 3134, 3032, 1550, 1508, 1159, 781, 669 cm−1; 1H NMR (CDCl3, 500 MHz) δ 1.24 (t, J = 7.5 Hz, 3H), 2.66 (q, J = 7.5 Hz, 2H), 5.58 (s, 2H), 7.23 (d, J = 8.0 Hz, 2H), 7.30 (s, 1H), 7.32 (d, J = 2.0 Hz, 1H), 7.41–7.37 (m, 3H), 7.62 (s, 1H), 7.23 (d, J = 8.0 Hz, 2H) ppm; 13C NMR (CDCl3, 125 MHz) δ 28.5, 29.6, 54.1, 119.0, 125.6, 127.9, 128.1, 128.3, 128.6, 129.0, 130.8, 134.6, 148.2 ppm. ESI-MS: m/z = 264 (M + 1)+; HRMS calcd for C17H18N3 (M + H)+: 264.1495; found: 264.1501.
1-Benzyl-4-(3-fluorophenyl)-1H-1,2,3-triazole (6c). Oil; IR (KBr): 3134, 3012, 1558, 1508, 1161, 779, 669 cm−1; 1H NMR (CDCl3, 500 MHz) δ 5.59 (s, 2H), 7.41–7.29 (m, 7H), 757–7.51 (m, 2H), 7.66 (s, 1H) ppm; 13C NMR (CDCl3, 125 MHz) δ 54.2, 119.5, 125.7, 128.1, 128.2, 128.8, 129.2, 130.6, 134.7, 148.3 ppm; 13C NMR (CDCl3, 125 MHz) δ 54.3, 112.6 (d, 2JCF = 22.8 Hz), 114.9, 121.3 (d, 4JCF = 2.9 Hz), 127.1, 128.1, 128.90, 128.91, 129.2, 130.4 (d, 3JCF = 8.9 Hz), 134.6, 147.2, 163.9 ppm. ESI-MS: m/z = 254 (M + 1)+.
1-Benzyl-4-(4-chlorophenyl)-1H-1,2,3-triazole (6d). Oil; IR (KBr): 3246, 3034, 1541, 1496, 1159, 779, 669 cm−1; 1H NMR (CDCl3, 500 MHz) δ 5.58 (s, 2H), 7.33–7.31 (m, 2H), 7.40–7.36 (m, 5H), 7.64 (s, 1H), 7.73 (d, J = 8.5 Hz, 2H) ppm; 13C NMR (CDCl3, 125 MHz) δ 54.3, 119.5, 127.0, 128.1, 128.9, 129.0, 129.1, 129.2, 133.9, 134.5, 147.2 ppm. ESI-MS: m/z = 270 (M + 1)+.
1-Benzyl-4-(4-bromophenyl)-1H-1,2,3-triazole (6e). White solid; IR (KBr): 3126, 3012, 1610, 1508, 1168, 1068, 727, 648 cm−1; 1H NMR (CDCl3, 500 MHz) δ 5.54 (s, 2H), 7.10 (d, J = 8.5 Hz, 2H), 7.32–7.29 (m, 2H), 7.54 (t, J = 8.5 Hz, 3H), 7.66 (t, J = 8.5 Hz, 2H), 7.74 (s, 1H) ppm; 13C NMR (CDCl3, 125 MHz) δ 52.0, 119.6, 127.1, 127.2, 128.1, 129.0, 129.2, 130.4, 132.0, 132.2, 147.2 ppm. ESI-MS: m/z = 314 (M + 1)+.
1-Benzyl-4-propyl-1H-1,2,3-triazole (6f). Oil; IR (KBr): 3090, 3034, 1629, 1496, 636, 475 cm−1; 1H NMR (CDCl3, 500 MHz) δ 0.98 (t, J = 7.5 Hz, 3H), 1.80–1.73 (m, 2H), 2.70 (t, J = 7.0 Hz, 2H), 5.52 (s, 2H), 7.37–7.30 (m, 6H) ppm; 13C NMR (CDCl3, 125 MHz) δ 13.8, 22.2, 27.6, 52.5, 103.0, 127.9, 128.2, 128.7, 135.2, 150.2 ppm. ESI-MS: m/z = 202 (M + 1)+.
1-Benzyl-4-pentyl-1H-1,2,3-triazole (6g). White solid; IR (KBr): 3134, 3034, 1639, 1550, 696, 461 cm−1; 1H NMR (CDCl3, 500 MHz) δ 0.87 (t, J = 7.0 Hz, 3H), 1.33–1.30 (m, 4H), 1.67–1.61 (m, 2H), 2.67 (t, J = 7.5 Hz, 2H), 5.47 (s, 2H), 7.21 (s, 1H), 7.24 (d, J = 7.5 Hz, 2H), 7.36–7.27 (m, 3H) ppm; 13C NMR (CDCl3, 125 MHz) δ 14.0, 22.4, 25.7, 29.1, 31.4, 53.9, 120.6, 127.9, 128.5, 129.0, 135.1, 148.9 ppm. ESI-MS: m/z = 230 (M + 1)+.
1-(4-Methylbenzyl)-4-phenyl-1H-1,2,3-triazole (6h). Oil; IR (KBr): 3158, 3043, 1629, 1545, 678, 480 cm−1; 1H NMR (CDCl3, 500 MHz) δ 2.35 (s, 3H), 5.52 (s, 2H), 7.22–7.17 (m, 4H), 7.29 (t, J = 7.5 Hz, 1H), 7.38 (t, J = 7.0 Hz, 2H), 7.61 (s, 1H), 7.78 (d, J = 7.5 Hz, 2H) ppm; 13C NMR (CDCl3, 125 MHz) δ 21.2, 54.1, 119.4, 125.7, 128.1, 128.8, 129.5, 129.8, 130.6, 131.7, 138.8, 148.2 ppm. ESI-MS: m/z = 250 (M + 1)+.
1-(4-Bromobenzyl)-4-phenyl-1H-1,2,3-triazole (6i). White solid; IR (KBr): 3126, 3026, 1654, 1460, 696, 669, 491 cm−1; 1H NMR (CDCl3, 500 MHz) δ 5.51 (s, 2H), 7.17 (d, J = 8.0 Hz, 2H), 7.31 (t, J = 7.5 Hz, 1H), 7.39 (t, J = 7.5 Hz, 2H), 7.51 (d, J = 8.0 Hz, 2H), 7.64 (s, 1H), 7.79 (d, J = 8.5 Hz, 2H) ppm; 13C NMR (CDCl3, 125 MHz) δ 53.5, 119.4, 123.0, 125.7, 128.3, 128.9, 129.7, 130.4, 132.4, 133.7, 148.4 ppm. ESI-MS: m/z = 314 (M + 1)+.
1,4-Diphenyl-1H-1,2,3-triazole (8a). White solid; IR (KBr): 3144, 3061, 2364, 1598, 1510, 1456 cm−1; 1H NMR (CDCl3, 500 MHz) δ 7.38 (t, J = 7.5 Hz, 1H), 7.49–7.46 (m, 3H), 7.56 (t, J = 7.5 Hz, 2H), 7.80 (d, J = 7.5 Hz, 2H), 7.92 (d, J = 7.0 Hz, 2H), 8.20 (s, 1H) ppm; 13C NMR (CDCl3, 125 MHz) δ 117.6, 120.6, 125.9, 128.4, 128.8, 128.9, 129.8, 130.3, 137.1, 148.4 ppm. ESI-MS: m/z = 222 (M + 1)+.
4-(4-Methoxyphenyl)-1-phenyl-1H-1,2,3-triazole (8b). White solid; IR (KBr): 3107, 3061, 2359, 1599, 1502, 1464, 1067 cm−1; 1H NMR (CDCl3, 500 MHz) δ 3.87 (s, 3H), 7.00 (d, J = 9.0 Hz, 2H), 7.45 (t, J = 7.5 Hz, 1H), 7.55 (t, J = 7.5 Hz, 2H), 7.79 (d, J = 7.5 Hz, 2H), 7.84 (d, J = 8.5 Hz, 2H), 8.11 (s, 1H) ppm; 13C NMR (CDCl3, 125 MHz) δ 55.4, 114.4, 116.8, 120.5, 123.0, 127.2, 128.7, 129.8, 137.2, 148.3, 159.8 ppm. ESI-MS: m/z = 252 (M + 1)+.
1-Phenyl-4-(p-tolyl)-1H-1,2,3-triazole (8c). White solid; IR (KBr): 3122, 3084, 2359, 1595, 1506, 1489 cm−1; 1H NMR (CDCl3, 500 MHz) δ 2.40 (s, 3H), 7.27 (d, J = 9.5 Hz, 2H), 7.45 (t, J = 7.5 Hz, 1H), 7.55 (t, J = 7.5 Hz, 2H), 7.80 (t, J = 7.0 Hz, 4H), 8.16 (s, 1H) ppm; 13C NMR (CDCl3, 125 MHz) δ 21.3, 117.3, 120.5, 125.8, 127.8, 128.7, 129.6, 129.8, 129.8, 134.8, 138.3, 148.5 ppm. ESI-MS: m/z = 236 (M + 1)+; HRMS calcd for C15H14N3 (M + H)+: 236.2912; found: 236.2916.
4-(4-Ethylphenyl)-1-phenyl-1H-1,2,3-triazole (8d). White solid; IR (KBr): 2983, 2914, 2359, 1573, 1504, 1454 cm−1; 1H NMR (CDCl3, 500 MHz) δ 1.28 (t, J = 8.0 Hz, 3H), 2.70 (q, J = 7.5 Hz, 1H), 7.30 (d, J = 8.0 Hz, 2H), 7.45 (t, J = 7.5 Hz, 1H), 7.55 (t, J = 7.5 Hz, 2H), 7.79 (d, J = 8.0 Hz, 2H), 7.83 (d, J = 8.0 Hz, 2H), 8.16 (s, 1H) ppm; 13C NMR (CDCl3, 125 MHz) δ 15.5, 28.7, 117.3, 120.5, 125.9, 127.7, 128.4, 128.7, 129.8, 137.1, 144.7, 148.5 ppm. ESI-MS: m/z = 250 (M + 1)+.
4-(4-Butylphenyl)-1-phenyl-1H-1,2,3-triazole (8e). White solid; IR (KBr): 3113, 2965, 2365, 1568, 1510, 1458 cm−1; 1H NMR (CDCl3, 500 MHz) δ 0.95 (t, J = 7.0 Hz, 3H), 1.42–1.35 (m, 2H), 1.67–1.61 (m, 2H), 2.66 (t, J = 7.5 Hz, 2H), 7.28 (d, J = 8.0 Hz, 2H), 7.46 (t, J = 7.5 Hz, 1H), 7.55 (t, J = 7.5 Hz, 2H), 7.83–7.79 (m, 4H), 8.16 (s, 1H) ppm; 13C NMR (CDCl3, 125 MHz) δ 14.0, 22.4, 33.6, 35.5, 117.2, 120.5, 125.8, 127.6, 128.7, 129.0, 129.8, 137.2, 143.4, 148.6 ppm. ESI-MS: m/z = 278 (M + 1)+.
4-(3-Fluorophenyl)-1-phenyl-1H-1,2,3-triazole (8f). White solid; IR (KBr): 3130, 2345, 1610, 1587, 1485, 1066 cm−1; 1H NMR (CDCl3, 500 MHz) δ 7.09–7.05 (m, 1H), 7.45–7.41 (m, 1H), 7.48 (t, J = 7.0 Hz, 1H), 7.56 (t, J = 8.0 Hz, 2H), 7.64 (d, J = 9.5 Hz, 1H), 7.68 (d, J = 8.0 Hz, 1H), 7.80 (d, J = 8.0 Hz, 2H), 8.21 (s, 1H) ppm; 13C NMR (CDCl3, 125 MHz) δ 112.7, 112.9, 115.2 (d, 2JCF = 21.0 Hz), 118.0, 120.6, 121.5 (d, 4JCF = 2.8 Hz), 129.0, 129.9, 130.5 (d, 3JCF = 8.3 Hz), 137.0, 147.4, 163.2 (d, 1JCF = 244.0 Hz) ppm. ESI-MS: m/z = 240 (M + 1)+.
4-(4-Chlorophenyl)-1-phenyl-1H-1,2,3-triazole (8g). White solid; IR (KBr): 3152, 2346, 1616, 1530, 1500, 796, 542 cm−1; 1H NMR (CDCl3, 500 MHz) δ 7.44 (d, J = 8.5 Hz, 2H), 7.48 (d, J = 7.5 Hz, 1H), 7.56 (d, J = 7.5 Hz, 2H), 7.79 (d, J = 8.0 Hz, 2H), 7.86 (d, J = 8.0 Hz, 2H), 8.19 (s, 1H) ppm; 13C NMR (CDCl3, 125 MHz) δ 117.7, 120.6, 127.1, 128.78, 128.85, 128.94, 129.2, 129.8, 130.9, 147.4 ppm. ESI-MS: m/z = 256 (M + 1)+.
4-(4-Bromophenyl)-1-phenyl-1H-1,2,3-triazole (8h). White solid; IR (KBr): 3144, 2374, 1616, 1558, 1498, 538 cm−1; 1H NMR (CDCl3, 500 MHz) δ 7.48 (t, J = 7.0 Hz, 1H), 7.61–7.55 (m, 4H), 7.80–7.78 (m, 4H), 8.20 (s, 1H) ppm; 13C NMR (CDCl3, 125 MHz) δ 117.7, 120.6, 122.4, 127.4, 128.9, 129.0, 129.9, 130.9, 132.1, 147.4 ppm. ESI-MS: m/z = 300 (M + 1)+.
4-([1,1′-Biphenyl]-4-yl)-1-phenyl-1H-1,2,3-triazole (8i). White solid; IR (KBr): 3130, 3044, 2382, 1614, 1506, 1467 cm−1; 1H NMR (CDCl3, 500 MHz) δ 7.37 (t, J = 7.0 Hz, 1H), 7.47 (t, J = 7.5 Hz, 3H), 7.57 (t, J = 8.0 Hz, 2H), 7.65 (d, J = 7.5 Hz, 2H), 7.71 (d, J = 8.0 Hz, 2H), 7.81 (d, J = 8.0 Hz, 2H), 8.00 (d, J = 8.0 Hz, 2H), 8.24 (s, 1H) ppm; 13C NMR (CDCl3, 125 MHz) δ 117.6, 120.6, 126.3, 127.5, 127.6, 128.82, 128.86, 129.2, 129.8, 130.9, 137.1, 140.6, 141.2, 148.1 ppm. ESI-MS: m/z = 298 (M + 1)+.
1-Phenyl-4-(thiophen-2-yl)-1H-1,2,3-triazole (8j). Pale yellow solid; IR (KBr): 3131, 3052, 2346, 1597, 1527, 1481 cm−1; 1H NMR (CDCl3, 500 MHz) δ 7.13–7.11 (m, 1H), 7.35 (d, J = 4.0 Hz, 1H), 7.49–7.45 (m, 2H), 7.55 (t, J = 7.5 Hz, 2H), 7.78 (d, J = 8.0 Hz, 2H), 8.11 (s, 1H) ppm; 13C NMR (CDCl3, 125 MHz) δ 117.1, 120.6, 124.6, 125.4, 127.7, 128.9, 129.8, 132.5, 143.5 ppm. ESI-MS: m/z = 228 (M + 1)+.
4-Phenyl-1-(p-tolyl)-1H-1,2,3-triazole (8k). White solid; IR (KBr): 2916, 2849, 2359, 1558, 1519, 1454 cm−1; 1H NMR (CDCl3, 500 MHz) δ 2.44 (s, 3H), 7.38–7.34 (m, 3H), 7.46 (t, J = 7.5 Hz, 2H), 7.67 (d, J = 8.0 Hz, 2H), 7.91 (d, J = 7.5 Hz, 2H), 8.15 (s, 1H) ppm; 13C NMR (CDCl3, 125 MHz) δ 21.1, 117.6, 120.5, 125.9, 128.4, 128.9, 130.3, 130.4, 138.9, 148.3 ppm. ESI-MS: m/z = 236 (M + 1)+.
1-(3-Fluorophenyl)-4-phenyl-1H-1,2,3-triazole (8l). White solid; IR (KBr): 3128, 3082, 2351, 1608, 1537, 1471, 1065 cm−1; 1H NMR (CDCl3, 500 MHz) δ 7.28–7.26 (m, 2H), 7.40 (t, J = 7.0 Hz, 1H), 7.48 (t, J = 7.5 Hz, 2H), 7.54–7.52 (m, 2H), 7.90–7.89 (m, 2H), 8.14 (s, 1H) ppm; 13C NMR (CDCl3, 125 MHz) δ 105.2, 105.3, 105.37, 105.4, 117.6 (d, 2JCF = 26.6 Hz), 125.9, 128.9 (d, 3JCF = 9.6 Hz), 129.0, 129.6, 130.8, 149.1, 150.1 (d, 1JCF = 246.4 Hz) ppm. ESI-MS: m/z = 240 (M + 1)+; HRMS calcd for C14H11N3F (M + H)+: 240.0932; found: 240.0934.
1-(4-Chlorophenyl)-4-phenyl-1H-1,2,3-triazole (8m). White solid; IR (KBr): 3130, 3097, 2344, 1608, 1558, 1481, 707 cm−1; 1H NMR (CDCl3, 500 MHz) δ 7.47 (t, J = 7.5 Hz, 2H), 7.53 (d, J = 8.5 Hz, 3H), 7.75 (d, J = 8.5 Hz, 2H), 7.90 (d, J = 8.0 Hz, 2H), 8.17 (s, 1H) ppm; 13C NMR (CDCl3, 125 MHz) δ 117.4, 121.7, 125.9, 128.6, 128.8, 129.0, 130.0, 130.9, 132.3, 141.2 ppm. ESI-MS: m/z = 256 (M + 1)+.
1-Phenyl-4-propyl-1H-1,2,3-triazole (8n). Oil; IR (KBr): 3090, 958, 2343, 1599, 1501, 1465 cm−1; 1H NMR (CDCl3, 500 MHz) δ 1.02 (t, J = 7.5 Hz, 3H), 1.81–1.75 (m, 2H), 2.78 (t, J = 7.5 Hz, 2H), 7.40 (t, J = 7.5 Hz, 1H), 7.50 (t, J = 7.5 Hz, 2H), 7.69 (s, 1H), 7.72 (d, J = 8.0 Hz, 2H) ppm; 13C NMR (CDCl3, 125 MHz) δ 13.8, 22.7, 27.7, 118.9, 120.4, 128.4, 129.7, 130.9, 149.0 ppm. ESI-MS: m/z = 188 (M + 1)+.
Acknowledgements
This work was financially supported by the National Natural Science Foundation of China (No. 21272053) and the Nature Science Foundation of Hebei Province (No. H2015206393).
References
-
(a) A. Kamal, B. Shaik, V. L. Nayak, B. Nagaraju, J. S. Kapure, M. S. Malik, T. B. Shaik and B. Prasad, Bioorg. Med. Chem., 2014, 22, 5155–5167 CrossRef CAS PubMed;
(b) M. F. Mady, G. E. A. Awad and K. B. Jorgensen, Eur. J. Med. Chem., 2014, 84, 433–443 CrossRef CAS PubMed;
(c) C. Testa, M. Scrima, M. Grimaldi, A. M. D'Ursi, M. L. Dirain, N. Lubin-Germain, A. Singh, C. Haskell-Luevano, M. Chorev, P. Rovero and A. M. Papini, J. Med. Chem., 2014, 57, 9424–9434 CrossRef CAS PubMed;
(d) D. S. Huang, P. X. Zhao and D. Astruc, Coord. Chem. Rev., 2014, 272, 145–165 CrossRef CAS PubMed;
(e) H. Singh, J. Sindhu and J. M. Khurana, J. Lumin., 2015, 158, 340–350 CrossRef CAS PubMed;
(f) B. T. Heng, G. Y. Yeap, W. A. K. Mahmood, C. C. Han, H. C. Lin, S. Takano and D. Takeuchi, Liq. Cryst., 2014, 41, 1897–2191 CrossRef CAS PubMed;
(g) D. Ghosh, S. Rhodes, K. Hawkins, D. Winder, A. Atkinson, W. Ming, C. Padgett, J. Orvis, K. Aiken and S. Landge, New J. Chem., 2015, 39, 295–303 RSC.
-
(a) D. B. Ramachary, A. B. Shashank and S. Karthik, Angew. Chem., Int. Ed., 2014, 53, 10420–10424 CrossRef CAS PubMed;
(b) J. Thomas, J. John, N. Parekh and W. Dehaen, Angew. Chem., Int. Ed., 2014, 53, 10155–10159 CrossRef CAS PubMed;
(c) Z. K. Chen, Q. Q. Yan, H. Yi, Z. X. Liu, A. W. Lei and Y. H. Zhang, Chem.–Eur. J., 2014, 20, 13692–13697 CrossRef CAS PubMed;
(d) A. Ali, A. G. Correa, D. Alves, J. Zukerman-Schpector, B. Westermann, M. A. B. Ferreira and M. W. Paixao, Chem. Commun., 2014, 50, 11926–21192 RSC.
- V. V. Rostovtsev, L. G. Green, V. V. Fokin and K. B. Sharpless, Angew. Chem., Int. Ed., 2002, 41, 2596–2599 CrossRef CAS.
-
(a) D. Wang, L. Etienne, M. Echeverria, S. Moya and D. Astruc, Chem.–Eur. J., 2014, 20, 4047–4054 CrossRef CAS PubMed;
(b) R. Hudson, C. J. Li and A. Moores, Green Chem., 2012, 14, 622–624 RSC;
(c) S. B. Otvos, G. Hatoss, A. Georgiades, S. Kovacs, I. M. Mandity, Z. Novak and F. Fulop, RSC Adv., 2014, 4, 46666–46674 RSC.
-
(a) C. O. Kappe and E. Van der Eycken, Chem. Soc. Rev., 2010, 39, 1280–1290 RSC;
(b) M. M. Heravi, H. Hamidi and V. Zadsirjan, Curr. Org. Synth., 2014, 11, 647–675 CrossRef CAS;
(c) H. Sharghi, M. Hosseini-Sarvari, F. Moeini, R. Khalifeh and A. S. Beni, Helv. Chim. Acta, 2010, 93, 435–449 CrossRef CAS PubMed;
(d) G. Kumaraswamy, K. Ankamma and A. Pitchaiah, J. Org. Chem., 2007, 72, 9822–9825 CrossRef CAS PubMed;
(e) J. S. Yadav, B. V. S. Reddy, G. M. Reddy and D. N. Chary, Tetrahedron Lett., 2007, 48, 8773–8776 CrossRef CAS PubMed;
(f) C. W. Shao, X. Y. Wang, J. M. Xu, J. C. Zhao, Q. Zhang and Y. F. Hu, J. Org. Chem., 2010, 75, 7002–7005 CrossRef CAS PubMed;
(g) K. Wang, X. H. Bi, S. X. Xing, P. Q. Liao, Z. X. Fang, X. Y. Meng, Q. A. Zhang, Q. Liu and Y. Ji, Green Chem., 2011, 13, 562–565 RSC.
- A. N. Prasad, B. Thirupathi, G. Raju, R. Srinivas and B. M. Reddy, Catal. Sci. Technol., 2012, 2, 1264–1268 CAS.
- T. Boningari, A. Olmos, B. M. Reddy, J. Sommer and P. Pale, Eur. J. Org. Chem., 2010, 6338–6347 CrossRef CAS PubMed.
- F. Alonso, Y. Moglie, G. Radivoy and M. Yus, J. Org. Chem., 2011, 76, 8394–8405 CrossRef CAS PubMed.
- H. Naeimi and V. Nejadshafiee, New J. Chem., 2014, 38, 5429–5435 RSC.
- M. C. Hashjin, R. Ciyabi, M. Baharloui, G. Hosseini and H. Tavakoli, Chin. J. Chem., 2012, 30, 223–227 CrossRef PubMed.
- B. Kumar, K. H. V. Reddy, G. Satish, R. U. Kumar and Y. V. D. Nageswar, RSC Adv., 2014, 4, 60652–60657 RSC.
- M. Ghohnejad and N. Jeddi, ACS Sustainable Chem. Eng., 2014, 2, 2658–2665 CrossRef.
- X. Q. Xiong, L. Cai, Y. B. Jiang and Q. Han, ACS Sustainable Chem. Eng., 2014, 2, 765–771 CrossRef CAS.
- X. Q. Xiong and L. Cai, Catal. Sci. Technol., 2013, 3, 1301–1307 CAS.
- H. Sharghi, R. Khalifeh and M. M. Doroodmand, Adv. Synth. Catal., 2009, 351, 207–218 CrossRef CAS PubMed.
- F. Alonso, Y. Moglie, G. Radivoy and M. Yus, Adv. Synth. Catal., 2010, 352, 3208–3214 CrossRef CAS PubMed.
- R. B. N. Baig and R. S. Varma, Green Chem., 2012, 14, 625–632 RSC.
- N. Mukherjee, S. Ahammed, S. Bhadra and B. C. Ranu, Green Chem., 2013, 15, 389–397 RSC.
- L. Wang and C. Cai, Green Chem. Lett. Rev., 2010, 3, 121–125 CrossRef CAS PubMed.
- Y. H. Tsai, K. Chanda, Y. T. Chu, C. Y. Chiu and M. H. Huang, Nanoscale, 2014, 6, 8704–8870 RSC.
- F. M. Moghaddam and S. E. Ayati, RSC Adv., 2015, 5, 3894–4390 RSC.
- P. V. Chavan, K. S. Pandit, U. V. Desai, M. A. Kulkarni and P. P. Wadgaonkar, RSC Adv., 2014, 4, 42137–42146 RSC.
- X. Q. Xiong, H. X. Chen, Z. K. Tang and Y. B. Jiang, RSC Adv., 2014, 4, 9830–9837 RSC.
- A. N. Prasad, B. M. Reddy, E. Y. Jeong and S. E. Park, RSC Adv., 2014, 4, 29772–29781 RSC.
- A. S. Kumar, M. A. Reddy, M. Knorn, O. Reiser and B. Sreedhar, Eur. J. Org. Chem., 2013, 2013, 4674–4680 CrossRef CAS PubMed.
- B. Kaboudin, R. Mostafalu and T. Yokomatsu, Green Chem., 2013, 15, 2266–2274 RSC.
- S. Mohammed, A. K. Padala, B. A. Dar, B. Singh, B. Sreedhar, R. A. Vishwakarma and S. B. Bharate, Tetrahedron, 2012, 68, 8156–8162 CrossRef CAS PubMed.
-
(a) A. Domling, W. Wang and K. Wang, Chem. Rev., 2012, 112, 3083–3135 CrossRef CAS PubMed;
(b) A. Ahmed, S. Dhara, R. Singha, Y. Nuree, P. Sarkar and J. K. Ray, RSC Adv., 2014, 4, 53137–53141 RSC;
(c) M. Jida, D. Tourwe and S. Ballet, RSC Adv., 2014, 4, 38159–38163 RSC;
(d) H. Moghanian, A. Mobinikhaledi, A. G. Blackman and E. Sarough-Farahani, RSC Adv., 2014, 4, 28176–28185 RSC;
(e) A. Mondal, M. Brown and C. Mukhopadhyay, RSC Adv., 2014, 4, 36890–36895 RSC;
(f) B. Paplal, S. Nagaraju, P. Veerabhadraiah, K. Sujatha, S. Kanvah, B. V. Kumar and D. Kashinath, RSC Adv., 2014, 4, 54168–54174 RSC;
(g) M. H. Li, A. Taheri, M. Liu, S. H. Sun and Y. L. Gu, Adv. Synth. Catal., 2014, 356, 537–556 CrossRef CAS PubMed;
(h) X. J. Pan, M. H. Li and Y. L. Gu, Chem.–Asian J., 2014, 9, 268–274 CrossRef CAS PubMed.
-
(a) Y. L. Gu and F. Jerome, Chem. Soc. Rev., 2013, 42, 9550–9570 RSC;
(b) P. Liu, J. W. Hao, L. P. Mo and Z. H. Zhang, RSC Adv., 2015, 5, 48675–48704 RSC;
(c) R. Y. Guo, P. Wang, G. D. Wang, L. P. Mo and Z. H. Zhang, Tetrahedron, 2013, 69, 2056–2061 CrossRef CAS PubMed.
-
(a) M. B. Gawande, V. D. B. Bonifacio, R. Luque, P. S. Branco and R. S. Varma, Chem. Soc. Rev., 2013, 42, 5522–5551 RSC;
(b) M. B. Gawande and P. S. Branco, Green Chem., 2011, 13, 3355–3359 RSC;
(c) S. R. Kale, S. S. Kahandal, A. S. Burange, M. B. Gawande and R. V. Jayaram, Catal. Sci. Technol., 2013, 3, 2050–2056 RSC;
(d) J. P. Wan, Y. F. Lin, K. K. Hu and Y. Y. Liu, RSC Adv., 2014, 4, 20499–20505 RSC;
(e) S. Karamthulla, S. Pal, M. N. Khan and L. H. Choudhury, RSC Adv., 2014, 4, 37889–37899 RSC.
-
(a) L. Wang and F. S. Xiao, Green Chem., 2015, 17, 24–39 RSC;
(b) D. Rath, S. Rana and K. M. Parida, RSC Adv., 2014, 4, 57111–57124 RSC;
(c) S. H. Wu, C. Y. Mou and H. P. Lin, Chem. Soc. Rev., 2013, 42, 3862–3875 RSC.
-
(a) M. Pang, C. Y. Liu, W. Xia, M. Muhler and C. H. Liang, Green Chem., 2012, 14, 1272–1276 RSC;
(b) M. Masteri-Farahani and S. Abednatanzi, Appl. Catal., A, 2014, 478, 211–218 CrossRef CAS PubMed.
-
(a) P. Puthiaraj and K. Pitchumani, Green Chem., 2014, 16, 4223–4233 RSC;
(b) W. Zhang, Q. X. Wang, H. H. Wu, P. Wu and M. Y. He, Green Chem., 2014, 16, 4767–4774 RSC;
(c) S. M. Islam, K. Ghosh, A. S. Roy and R. A. Molla, RSC Adv., 2014, 4, 38986–38999 RSC;
(d) S. Itsuno and M. M. Hassan, RSC Adv., 2014, 4, 52023–52043 RSC.
-
(a) R. S. Thombal, A. P. Jadhv and V. H. Jadhav, RSC Adv., 2015, 5, 12981–12986 RSC;
(b) S. Keshipour and A. Shaabani, Appl. Organomet. Chem., 2014, 28, 116–119 CrossRef CAS PubMed;
(c) A. M. R. Galletti, C. Antonetti, M. Bertoldo and F. Piccinelli, Appl. Catal., A, 2013, 468, 95–101 CrossRef PubMed;
(d) A. Khazaei, S. Rahmati, Z. Hekmatian and S. Saeednia, J. Mol. Catal. A: Chem., 2013, 372, 160–166 CrossRef CAS PubMed.
-
(a) D. Wang and D. Astruc, Chem. Rev., 2014, 114, 6949–6985 CrossRef CAS PubMed;
(b) L. M. Rossi, N. J. S. Costa, F. P. Silva and R. Wojcieszak, Green Chem., 2014, 16, 2906–2933 RSC;
(c) R. Mrowczynski, A. Nan and J. Liebscher, RSC Adv., 2014, 4, 5927–5952 RSC;
(d) M. B. Gawande, P. S. Branco and R. S. Varma, Chem. Soc. Rev., 2013, 42, 3371–3393 RSC;
(e) K. Turcheniuk, A. V. Tarasevych, V. P. Kukhar, R. Boukherroub and S. Szunerits, Nanoscale, 2013, 5, 10729–10752 RSC;
(f) M. B. Gawande, R. Luque and R. Zboril, ChemCatChem, 2014, 6, 3312–3313 CAS.
-
(a) S. N. Shelke, S. R. Bankar, G. R. Mhaske, S. S. Kadam, D. K. Murade, S. B. Bhorkade, A. K. Rathi, N. Bundaleski, O. Teodoro, R. Zboril, R. S. Varma and M. B. Gawande, ACS Sustainable Chem. Eng., 2014, 2, 1699–1706 CrossRef CAS;
(b) S. Sa, M. B. Gawande, A. Velhinho, J. P. Veiga, N. Bundaleski, J. Trigueiro, A. Tolstogouzov, O. Teodoro, R. Zboril, R. S. Varma and P. S. Branco, Green Chem., 2014, 16, 3494–3500 RSC;
(c) M. B. Gawande, A. K. Rathi, J. Tucek, K. Safarova, N. Bundaleski, O. Teodoro, L. Kvitek, R. S. Varma and R. Zboril, Green Chem., 2014, 16, 4137–4143 RSC;
(d) A. S. Burange, S. R. Kale, R. Zboril, M. B. Gawande and R. V. Jayaram, RSC Adv., 2014, 4, 6597–6601 RSC;
(e) S. R. Kale, S. S. Kahandal, M. B. Gawande and R. V. Jayaram, RSC Adv., 2013, 3, 8184–8192 RSC;
(f) M. B. Gawande, A. K. Rathi, I. D. Nogueira, R. S. Varma and P. S. Branco, Green Chem., 2013, 15, 1895–1899 RSC;
(g) M. B. Gawande, H. Z. Guo, A. K. Rathi, P. S. Branco, Y. Z. Chen, R. S. Varma and D. L. Peng, RSC Adv., 2013, 3, 1050–1105 RSC;
(h) C. G. S. Lima, S. Silva, R. H. Goncalves, E. R. Leite, R. S. Schwab, A. G. Correa and M. W. Paixao, ChemCatChem, 2014, 6, 3455–3463 CAS;
(i) H. Ziyadi and A. Heydari, Catal. Lett., 2014, 144, 2210–2215 CrossRef CAS PubMed;
(j) A. Mobaraki, B. Movassagh and B. Karimi, Appl. Catal., A, 2014, 472, 123–133 CrossRef CAS PubMed;
(k) M. Shokouhimehr, T. Kim, S. W. Jun, K. Shin, Y. Jang, B. H. Kim, J. Kim and T. Hyeon, Appl. Catal., A, 2014, 476, 133–139 CrossRef CAS PubMed.
-
(a) Zillillah, T. A. Ngu and Z. Li, Green Chem., 2014, 16, 1202–1210 RSC;
(b) S. M. Sadeghzadeh, RSC Adv., 2014, 4, 43315–43320 RSC;
(c) K. Pradhan, S. Paul and A. R. Das, Catal. Sci. Technol., 2014, 4, 822–831 RSC;
(d) M. A. Nasseri, B. Zakerinasab and M. M. Samieadel, RSC Adv., 2014, 4, 41753–44176 RSC;
(e) M. Nasrollahzadeh, M. Maham, A. Ehsani and M. Khalaj, RSC Adv., 2014, 4, 19731–19736 RSC;
(f) H. Naeimi, Z. Rashid, A. H. Zarnani and R. Ghahremanzadeh, New J. Chem., 2014, 38, 5527–5535 RSC;
(g) H. Moghanian, A. Mobinikhaledi, A. G. Blackman and E. Sarough-Farahani, RSC Adv., 2014, 4, 28176–28185 RSC;
(h) H. Woo, K. Lee, J. C. Park and K. H. Park, New J. Chem., 2014, 38, 5626–6563 RSC;
(i) H. Mahmoudi, A. A. Jafari, S. Saeedi and H. Firouzabadi, RSC Adv., 2015, 5, 3023–3030 RSC;
(j) A. Khazaei, A. R. Moosavi-Zare, H. Afshar-Hezarkhani and V. Khakyzadeh, RSC Adv., 2014, 4, 32142–32147 RSC;
(k) X. N. Zhao, G. F. Hu, M. Tang, T. T. Shi, X. L. Guo, T. T. Li and Z. H. Zhang, RSC Adv., 2014, 4, 51089–51097 RSC.
-
(a) J. Deng, L. P. Mo, F. Y. Zhao, L. L. Hou, L. Yang and Z. H. Zhang, Green Chem., 2011, 13, 2576–2584 RSC;
(b) Y. H. Liu, J. Deng, J. W. Gao and Z. H. Zhang, Adv. Synth. Catal., 2012, 354, 441–447 CrossRef CAS PubMed;
(c) P. H. Li, B. L. Li, Z. M. An, L. P. Mo, Z. S. Cui and Z. H. Zhang, Adv. Synth. Catal., 2013, 355, 2952–2959 CrossRef CAS PubMed;
(d) F. P. Ma, P. H. Li, B. L. Li, L. P. Mo, N. Liu, H. J. Kang, Y. N. Liu and Z. H. Zhang, Appl. Catal., A, 2013, 457, 34–41 CrossRef CAS PubMed;
(e) J. Lu, X. T. Li, E. Q. Ma, L. P. Mo and Z. H. Zhang, ChemCatChem, 2014, 6, 2854–2859 CrossRef CAS PubMed;
(f) X. N. Zhao, H. C. Hu, F. J. Zhang and Z. H. Zhang, Appl. Catal., A, 2014, 482, 258–265 CrossRef CAS PubMed;
(g) P. H. Li, B. L. Li, H. C. Hu, X. N. Zhao and Z. H. Zhang, Catal. Commun., 2014, 46, 118–122 CrossRef CAS PubMed;
(h) B. L. Li, H. C. Hu, L. P. Mo and Z. H. Zhang, RSC Adv., 2014, 4, 12929–12943 RSC;
(i) B. L. Li, M. Zhang, H. C. Hu, X. Du and Z. H. Zhang, New J. Chem., 2014, 38, 2435–2442 RSC.
-
(a) Z. H. Zhang, X. N. Zhang, L. P. Mo, Y. X. Li and F. P. Ma, Green Chem., 2012, 14, 1502–1506 RSC;
(b) R. Y. Guo, Z. M. An, L. P. Mo, R. Z. Wang, H. X. Liu, S. X. Wang and Z. H. Zhang, ACS Comb. Sci., 2013, 15, 557–656 CrossRef CAS PubMed;
(c) X. T. Li, A. D. Zhao, L. P. Mo and Z. H. Zhang, RSC Adv., 2014, 4, 51580–51588 RSC;
(d) H. C. Hu, Y. H. Liu, B. L. Li, Z. S. Cui and Z. H. Zhang, RSC Adv., 2015, 5, 7720–7728 RSC;
(e) X. T. Li, Y. H. Liu, X. Liu and Z. H. Zhang, RSC Adv., 2015, 5, 25625–25633 RSC.
- C. W. Shao, G. L. Cheng, D. Y. Su, J. M. Xu, X. Y. Wang and Y. F. Hu, Adv. Synth. Catal., 2010, 352, 1587–1592 CrossRef CAS PubMed.
- X. Meng, X. Y. Xu, T. T. Gao and B. H. Chen, Eur. J. Org. Chem., 2010, 5409–5414 CrossRef CAS PubMed.
- F. Alonso, Y. Moglie, G. Radivoy and M. Yus, Org. Biomol. Chem., 2011, 9, 6385–6395 CAS.
- Z. K. Chen, Q. Q. Yan, Z. X. Liu, Y. M. Xu and Y. H. Zhang, Angew. Chem., Int. Ed., 2013, 52, 13324–13328 CrossRef CAS PubMed.
- J. Andersen, S. Bolvig and X. F. Liang, Synlett, 2005, 2941–2947 CAS.
- B. S. P. Anil Kumar, K. H. V. Reddy, K. Karnakar, G. Satish and Y. V. D. Nageswar, Tetrahedron Lett., 2015, 56, 1968–1972 CrossRef CAS PubMed.
- G. Colombano, C. Travelli, U. Galli, A. Caldarelli, M. G. Chini, P. L. Canonico, G. Sorba, G. Bifulco, G. C. Tron and A. A. Genazzani, J. Med. Chem., 2010, 53, 616–623 CrossRef CAS PubMed.
- Y. B. Cai, L. Liang, J. Zhang, H.-L. Sun and J.-L. Zhang, Dalton Trans., 2013, 42, 5390–5400 RSC.
Footnote |
† Electronic supplementary information (ESI) available. See DOI: 10.1039/c5ra09517d |
|
This journal is © The Royal Society of Chemistry 2015 |
Click here to see how this site uses Cookies. View our privacy policy here.