DOI:
10.1039/C5RA07803B
(Paper)
RSC Adv., 2015,
5, 51233-51241
Phytoprostanes in almonds: identification, quantification, and impact of cultivar and type of cultivation
Received
28th April 2015
, Accepted 1st June 2015
First published on 1st June 2015
Abstract
Recently, the relationship between oxidative stress and the phytoprostanes content in plants has been studied. In 1998, it was discovered that phytoprostanes are generated from α-linolenic acid, following a non-enzymatic pathway initiated by enhanced formation of free radicals. Almonds and other nuts have favorable contents of polyunsaturated fatty acids; one of the most-polyunsaturated fatty acids present in plants is α-linolenic acid. This study represents a first approach to the quantitative and qualitative determination of the phytoprostanes profile in 11 almond cultivars under different agronomic conditions (conventional versus ecological, rain-fed versus irrigated). In the kernels have been identified 9-F1t-phytoprostane, 9-epi-9-F1t-phytoprostane, ent-16-epi-16-F1t-phytoprostane, ent-16-F1t-phytoprostane, 9-D1t-phytoprostane, 9-epi-9-D1t-phytoprostane, 16-B1-phytoprostane, and 9-L1-phytoprostane. The total phytoprostane content was in the range of 4.0 to 23.8 ng per 100 g. F1-phytoprostanes predominated and were identified in all almond cultivars. L1-phytoprostanes were minor components while D1-phytoprostanes were only detected in cultivars ‘Colorada’ and ‘Avellanera’. The phytoprostane profile varied greatly depending on the genotype, but was also affected by factors such as the agricultural system (conventional or ecological) and irrigation. The ecological system promoted the synthesis of D1-phytoprostanes. Almonds from rain-fed trees had lower individual and total phytoprostane concentrations than those under irrigation, even though non-irrigation led to the detection of the 16-F1-phytoprostanes. Consequently, irrigation and ecological techniques applied to almonds could be considered as actions to enhance their phytoprostane content and hence their potential beneficial effects on human health.
1. Introduction
In the early 1990s, a series of prostaglandin compounds was identified as being produced in mammals, independently of the cyclooxygenase pathway, from arachidonic acid.1 These compounds were termed isoprostanes (IsoPs) because their cyclopentane structure is closely related to that of the prostaglandins, although, in contrast to prostaglandins, they are completely racemic and the characteristic lipidic side chains have a cis relationship. Nevertheless, higher plants generally do not possess arachidonic acid (C20:4 n-6, AA), but do produce α-linolenic acid (C18:3 n-3, ALA) which gives rise to oxylipins, generated spontaneously from this PUFA by a non-enzymatic pathway that is stimulated by the enhanced formation of reactive oxygen species (ROS).2 By analogy with isoprostanes, these oxylipins are denominated phytoprostanes (PhytoPs). Under normal conditions plants are continuously producing ROS, hence generating the spontaneous formation of oxidized fatty acid derivatives, but the intensity of this process increases significantly under conditions of abiotic and biotic stress.3–6
Therefore, PhytoP production is enhanced by greater exposure to ROS. Several authors have proposed that PhytoPs are not only excellent biomarkers of the oxidative degradation of plant derived food stuffs, but also are biologically active molecules because they are components of an oxidant injury sensing, archaic signaling system that induces several plant defense mechanisms.3,5,7–10 PhytoPs accumulation is a transient process, suggesting that these biomarkers are rapidly generated and metabolized in vivo.2
The field of PhytoPs was opened in 2000, and currently it is still in its infancy. We have focused this study on almonds since they are nutrient-dense foods that can be a valuable plant source of healthy lipids in the diet.11 Consumption of almonds has been associated with a decrease of the risk factors in coronary heart disease and metabolic syndrome.12 This may be related to their favorable profile of unsaturated fatty acids (LA and ALA), that are beneficial in the good balance of fatty acids omega 3 and 6. Almonds are a good dietary source of vitamin E, sterols, and flavonoids, each of which has been suggested to play a role in the promotion of health.13 Since free radicals are proposed to play a key role in the pathology of diseases such as cancer, atherosclerosis, or inflammatory diseases,14 the supply of antioxidants via the food chain is of high importance for a healthy life. Moreover, previous reports confirm that genetics, time of harvesting, source, environmental conditions, soil composition, stage of maturity, and cultivation method affect the composition of almonds.15–17 These facts make almonds a good candidate for the study of PhytoPs as excellent biomarkers of the oxidative degradation within plants.
For all these reasons, the aim of this study was the identification and quantification of the PhytoPs presents in sweet almonds (Prunus dulcis (Miller) D.A. Webb) and the elucidation of the impact of the cultivar and cultivation conditions on the generation of these compounds.
2. Materials and methods
2.1 Chemicals and reagents
PhytoPs standards including 9-F1t-PhytoP, 9-epi-9-F1t-PhytoP, ent-16-F1t-PhytoP, ent-16-epi-16-F1t-PhytoP, 9-D1t-PhytoP, 9-epi-9-D1t-PhytoP, 16-B1-PhytoP, and 9-L1-PhytoP were synthesized according to our published procedures.18–20 The d4-15-F2t-IsoP (8-isoPGF2α-d4) (containing four deuterium atoms at positions 3,3′,4, and 4′), used as an internal standard, was purchased from Cayman Chemicals (Ann Arbor, Michigan, USA). Hexane was obtained from Panreac (Castellar del Vallés, Barcelona, Spain), while bis–tris (bis (2-hydroxyethyl) amino tris(hydroxymethyl)methane) and all LC-MS grade solvents, methanol, acetonitrile, and 2,6-di-tert-butyl-4-methylphenol (BHA) were purchased from Sigma Aldrich (St. Louis, MO, USA). The SPE cartridges used were Strata cartridges (Strata X-AW, 100 mg per 3 mL), acquired from Phenomenex (Torrance, CA, USA).
2.2 Experimental design and study conditions
Three experiments were carried out in order to determine the presence, identity and quantity of PhytoPs in almonds, their variability according to the cultivar, and the impact of a conventional versus an ecological system and rain-fed versus irrigation conditions (Table 1).
Table 1 Experimental design: impact of the cultivar, cultivation method (conventional or ecological), and irrigation system on the phytoprostanes profile
Experiment 1 |
Rain-fed almond cultivars under a conventional system: comparison of 11 cultivars |
Experiment 2 |
Seven rain-fed almonds cultivars: conventional versus ecological system |
Experiment 3 |
One almond cv. under a conventional system: rain-fed versus irrigation |
All the cultivars were grown under Mediterranean condition, in the Sierra Espuña and Lorca areas (Murcia, Spain). This area has a typical semi-arid climate: in the experimental period, August 2012, August 2013, there were 1552 hours of sunshine, the ETo was 553.31 mm, and the rainfall was 189.41 mm, concentrated mainly in the autumn. All the almond cultivars were grafted on ‘Garrigues franco’ plum rootstock, at a spacing of 7 × 7 m. The harvesting was by hand, in August 2013. The three experiments were developed as described below:
a) In the first, 11 almond cultivars (cvs) were selected: ‘Planeta’, ‘Blanqueta’, ‘Guara’, ‘Progreso’, ‘Marcona’, ‘Colorada’, ‘Largueta’, ‘Garriges’, ‘Atocha’, ‘Ferraduel’, and ‘Avellanera’. All were grown under rain-fed conditions, without supplemental irrigation, and under a conventional system. In this conventional system a fertilization and phytosanitary program was applied. Soil nutrients were supplied during the months of November and December, depending on the natural rainfall. The doses of fertilizer applied (Fertilizer Units per hectare and year, F.U. per ha year) were N (30–60), P2O5 (25–50), and K2O (50–75). The phytosanitary treatment of the almond trees, performed in winter time, was based on two fungicides: paraffin oil and copper oxychloride (Cu2(OH)3Cl). The applied doses were those recommended by the commercial brand.
b) In the second experiment, the conventional and ecological systems were compared for seven almond cvs (‘Planeta’, ‘Marcona’, ‘Colorada’, ‘Atocha’ ‘Largueta’, ‘Garriges’, ‘Avellanera’), grown under rain-fed conditions. The fertilizer used for the ecological cultivation was based on 2000 kg ha−1 of manure plus l,000 L ha−1 of pig slurry, applied from March to October. The ecological phytosanitary treatment was the same as for the conventional system (paraffin oil and copper oxychloride).
c) For the third experiment, rain-fed conditions and irrigation were compared. For this, cv. ‘Garriges’ was grown in a conventional system. The irrigation dose was 4707 m3 ha−1 per year.
In all the experiments, after the harvest for each treatment, almonds (≈100
000 kg) were transported to the company COATO (Totana, Murcia) where the fruits were peeled automatically to obtain the edible kernel. For each treatment, five replicates (250 g of kernels each) were selected and sent to the laboratory to be analyzed.
2.3 Sample preparation and phytoprostane analysis
The kernels from each replicate were immediately crushed in a coffee mill to a fine powder; then, 2–3 g (fresh weight) were suspended in a solution containing 10 mL of BHA plus methanol (10
:
01 v/v), stirred for 5 min, and centrifuged (2000g for 10 min). The supernatant was extracted and filtered through a Sep-Pack C18 cartridge (Waters, Mildford, MA).
2.3.1 Sample extraction. The PhytoPs present in the samples were isolated by carrying out a dilution followed by a solid phase extraction (SPE).15 Briefly, the protocol used was as follows: 1 mL of extracted and filtered sample was dissolved in 1 mL of n-hexane.21 The solution was rediluted in 2 mL of methanol plus 2 mL of bis–tris buffer (0.02 M, HCl, pH = 7). This solution was loaded into a Strata X-AW cartridge (100 mg per 3 mL) to carry out the SPE.15,21 Prior to loading of the sample, the cartridge was conditioned with 2 mL of hexane, 2 mL of methanol, and 2 mL of MilliQ water. After sample loading, and to remove other compounds, the cartridge was washed with 2 mL of hexane, 2 mL of MilliQ water, 2 mL of a solution that contained Methanol and MilliQ water (3/1), and 2 mL of acetonitrile. The target compounds were eluted with 1 mL of methanol and dried using a SpeedVac concentrator (Savant SPD121P, Thermo Scientific, MA, USA). The dry extracts were reconstituted with 200 µL of solvents A/B (90
:
10, v/v) solvent A being a MilliQ water/0.01% (v/v) acetic acid mixture, while a solution of methanol/0.01% (v/v) acetic acid was used as solvent B. After that, the samples were sonicated for 10 min and centrifuged (10
000g for 10 min) before being filtered through a 0.45 µm filter (Millipore, MA, USA). Of each sample, 20 µL were injected and analyzed in a UHPLC-QqQ-MS/MS (Agilent Technologies, Waldbronn, Germany). Two injections per sample were performed.
2.3.2 Preparation of PhytoPs standards. Stock solutions of PhytoPs were prepared in methanol/water (50
:
50, v/v), to facilitate the ionization process in the mass spectrometer, at a concentration of 1000 nM for each compound, and were stored in Eppendorf tubes at −80 °C.
2.3.3 UHPLC-QqQ-MS/MS analyses. Separation of the PhytoPs present in the almond samples was performed using a UHPLC coupled with a 6460 triple quadrupole-MS/MS (Agilent Technologies, Waldbronn, Germany). This methodology has been previously validated by Collado-González and co-workers.15 The analytical column was a BEH C18 (2.1 × 50 mm, pore size 1.7 µm) (Waters, Milford, M.A.), inserted in a column oven at 6 °C (both, left and right). The mobile phases consisted of water/acetic acid (99.99
:
0.01, v/v) (A) and methanol/acetic acid (99.99
:
0.01, v/v) (B). The injection volume was 20 µL and elution was performed at a flow rate of 0.2 mL min−1. The linear gradient started with 60% B at 0 min, reaching 62% B at 2 min and 62.5% B at 4 min, achieving 65% B at 8 min, and returning to the initial conditions at 8.01 min. The acquisition time was 8.01 min for each sample, with a post-run of 1.5 min for the column equilibration. The analyses were performed by multiple reaction monitoring (MRM) in the negative mode. The ESI conditions and ion optics were as previously described by Collado-González et al.15 Data acquisition and processing were performed using Mass Hunter software version B.04.00 (Agilent Technologies). The quantification of PhytoPs detected in the kernels was performed using authentic standards of 9-F1t-PhytoP, 9-epi-9-F1t-PhytoP, ent-16-epi-16-F1t-PhytoP, ent-16-F1t-PhytoP, 9-D1t-PhytoP, 9-epi-9-D1t-PhytoP, 16-B1-PhytoP, and 9-L1-PhytoP. The synthetic isoprostane d4-15-F2t-IsoP (8-isoPGF2α-d4, containing four deuterium atoms at positions 3,3′,4, and 4′) was used as internal standard.
2.4 Statistical analysis
For statistical analysis, the average of five repetitions per treatment and the standard error (mean ± SE) were calculated.
3. Results
3.1 Experiment 1: identification and quantification of phytoprostanes in different almond cultivars cultivated under rain-fed conditions and in a conventional system
PhytoPs were identified on the basis of their mass spectra, considering their molecular ion (m/z 327.2, 325.2, and 307.2), their most characteristic fragmentations, and their elution order according to their retention times, as described by Collado-González et al.15 (Fig. 1). In this study, we worked with 13 almond cultivars and eight authentic standards belonging to two series (9 and 16) of the F1-, D1-, B1-, and L1-PhytoP series (see chemical and reagents section). The PhytoP profile of the almond kernels is shown in Table 2 and Fig. 1, which indicate the occurrence of 9-F1t-PhytoP, 9-epi-9-F1t-PhytoP, ent-16-epi-16-F1t-PhytoP, ent-16-F1t-PhytoP, 9-D1t-PhytoP, 9-epi-9-D1t-PhytoP, 16-B1-PhytoP, and 9-L1-PhytoP. The total analyzed content of PhytoPs was in the range of 4025 to 23
809 ng per 100 g FW. This method allowed the detection of eight PhytoPs in the almond cvs, but not all of them were found in all the cvs. The 9-series of F1t-PhytoPs was the dominant type of PhytoP and was identified in all the cvs. The 9-series of L1-PhytoPs was the minor component. There were two cvs, Colorada and Avellanera, where the 16-series of F1t-PhytoPs was not detected, whereas the 9-series of D1t-PhytoPs was only detected in these cultivars, at low concentrations (23 to 43 ng per 100 g f.w.).
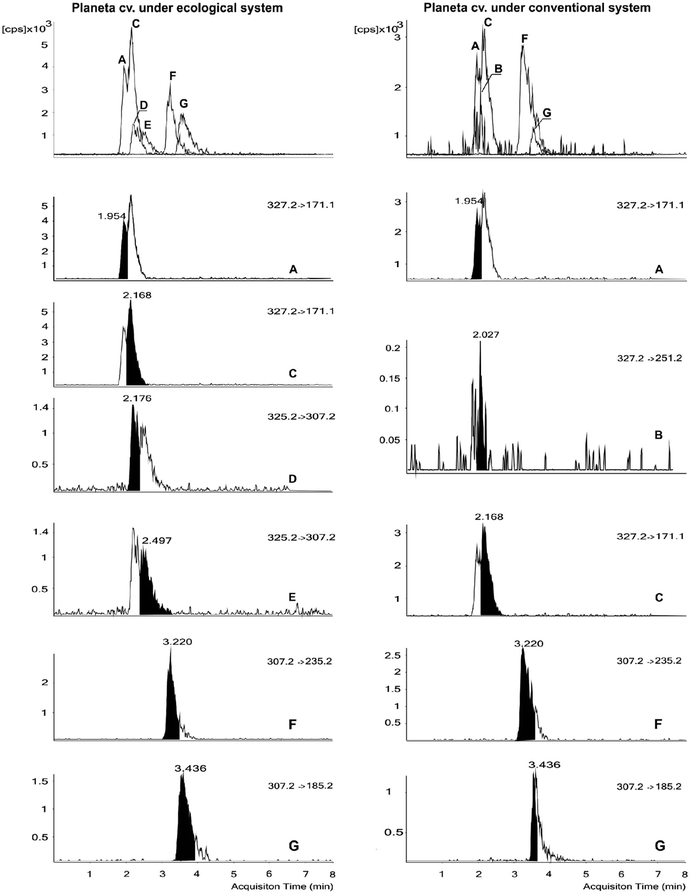 |
| Fig. 1 Comparison of representative UHPLC-MS/MS chromatograms of the phytoprostanes with their chemical structures and their MRM (multiple reaction monitoring) transitions, for their quantification in kernels from almond trees (cv. Planeta) grown in conventional and ecological systems. 9-F1t-PhytoP (A); ent-16-epi-16-F1t-PhytoP + ent-16-F1t-PhytoP (B); 9-epi-9-F1t-PhytoP (C); 9-epi-9-D1t-PhytoP (D); 9-D1t-PhytoP (E); 16-B1-PhytoP (F); 9-L1-PhytoP (G). | |
Table 2 Phytoprostanes (ng per 100 g) in different almond cultivars grown under rain-fed and conventional conditions
Cultivar |
9-F1t-PhytoP |
9-epi-9-F1t-PhytoP |
9-epi-9-D1t-PhytoP |
9-D1t-PhytoP |
ent-16-epi-16-F1t-PhytoP + ent-16-F1t-PhytoP |
16-B1-PhytoP |
9-L1-PhytoP |
Total |
Planeta |
2746.8 ± 42.7 |
2232.1 ± 63.1 |
nd |
nd |
22.9 ± 2.0 |
472.9 ± 24.0 |
159.0 ± 9.5 |
5633.6 ± 28.3 |
Blanqueta |
9623.5 ± 136.2 |
9915.6 ± 206.8 |
nd |
nd |
104.3 ± 5.1 |
3009.4 ± 84.2 |
1155.9 ± 58.2 |
23808.7 ± 98.1 |
Guara |
6682.4 ± 367.6 |
6079.1 ± 353.3 |
nd |
nd |
111.6 ± 30.0 |
1514.3 ± 205.7 |
415.8 ± 73.8 |
14803.1 ± 206.1 |
Progreso |
6257.2 ± 205.8 |
4718.3 ± 543.0 |
nd |
nd |
99.3 ± 10.1 |
1260.3 ± 3.4 |
435.0 ± 109.9 |
12770.1 ± 174.4 |
Marcona |
6524.1 ± 10.1 |
6558.3 ± 204.6 |
nd |
nd |
170.8 ± 5.0 |
1318.6 ± 27.5 |
609.1 ± 48.2 |
15180.9 ± 59.1 |
Colorada |
3394.1 ± 202.7 |
1387.4 ± 90.6 |
41.3 ± 10.1 |
22.5 ± 9.6 |
nd |
229.2 ± 48.3 |
272.0 ± 54.8 |
5346.5 ± 69.4 |
Largueta |
6748.6 ± 25.4 |
5715.3 ± 296.0 |
nd |
nd |
132.1 ± 40.9 |
377.5 ± 4.3 |
122.4 ± 14.4 |
13095.8 ± 76.2 |
Garriges |
1909.9 ± 175.7 |
1881.8 ± 381.9 |
nd |
nd |
39.5 ± 2.1 |
155.8 ± 49.3 |
38.0 ± 10.2 |
4025.0 ± 123.9 |
Atocha |
2832.1 ± 190.6 |
2728.2 ± 233.3 |
nd |
nd |
49.2 ± 13.8 |
500.2 ± 51.4 |
201.1 ± 37.2 |
6310.8 ± 105.3 |
Ferraduel |
4460.8 ± 882.3 |
5276.3 ± 727.5 |
nd |
nd |
81.8 ± 12.1 |
735.5 ± 137.7 |
280.7 ± 59.8 |
5558.8 ± 363.9 |
Avellanera |
2140.7 ± 186.6 |
1802.5 ± 65.0 |
42.9 ± 6.1 |
20.4 ± 1.6 |
nd |
285.2 ± 10.1 |
268.2 ± 3.1 |
4274.7 ± 45.4 |
The presence and concentration of every PhytoP were strongly influenced by the cv. Blanqueta, with a total concentration of 23
809 ng per 100 g, followed by Marcona, Guara, Largueta, and Progreso (12
770 to 15
180 ng pert 100 g), showed the highest level of PhytoPs, in particular for PhytoPs of the F1- and B1- types. In contrast, Garriges was the cv. with the lowest concentration of total PhytoPs (4025 ng per 100 g).
3.2 Experiment 2: comparison of conventional and ecological systems in eight almond cultivars grown under rain-fed conditions
In seven cultivars assessed also in the first experiment, the same PhytoPs were detected (Table 3). The great differences between the conventional and ecological systems were the change of PhytoPs concentrations and the detection of new PhytoPs (Fig. 1), namely the 9-series of D1-PhytoPs in cv. ‘Planeta’ and the 16-series of F1t-PhytoPs in ‘Colorada’ which were detected under ecological conditions.
Table 3 Phytoprostanes (ng per 100 g) in different almond cultivars grow under rain-fed and conventional (C) or ecological (E) conditions
Cultivar |
9-F1t-PhytoP |
9-epi-9-F1t-PhytoP |
9-epi-9-D1t-PhytoP |
9-D1t-PhytoP |
ent-16-epi-16-F1t-PhytoP + ent-16-F1t-PhytoP |
16-B1-PhytoP |
9-L1-PhytoP |
Total |
Planeta |
C |
2746.8 ± 42.7 |
2232.1 ± 60.1 |
— |
— |
22.9 ± 2.0 |
472.9 ± 24.0 |
158.9 ± 9.5 |
5633.6 ± 28.3 |
E |
4050.8 ± 172.0 |
4011.5 ± 347.3 |
151.3 ± 24.9 |
41.4 ± 7.8 |
— |
417.6 ± 52.6 |
435.8 ± 48.1 |
9108.3 ± 108.8 |
Marcona |
C |
6524.1 ± 10.1 |
6558.3 ± 204.6 |
— |
— |
170.8 ± 5.0 |
1318.6 ± 27.5 |
609.1 ± 48.3 |
15180.9 ± 59.1 |
E |
4652.8 ± 522.7 |
5527.3 ± 670.8 |
— |
— |
85.0 ± 18.0 |
343.2 ± 51.3 |
125.5 ± 14.5 |
10733.6 ± 255.4 |
Colorada |
C |
3394.1 ± 202.7 |
2232.1 ± 90.6 |
41.3 ± 10.1 |
22.5 ± 9.6 |
— |
229.2 ± 48.3 |
272.0 ± 54.7 |
5346.5 ± 69.4 |
E |
3397.0 ± 69.0 |
2660.9 ± 602.9 |
— |
— |
51.0 ± 5.2 |
543.4 ± 207.5 |
191.4 ± 62.0 |
6843.7 ± 189.3 |
Largueta |
C |
6748.6 ± 78.4 |
5715.3 ± 296.0 |
— |
— |
132.1 ± 40.9 |
377.5 ± 4.3 |
122.4 ± 14.4 |
13095.8 ± 76.2 |
E |
3627.1 ± 25.4 |
3105.3 ± 259.8 |
— |
— |
79.4 ± 11.6 |
642.7 ± 50.8 |
244.1 ± 33.3 |
7698.5 ± 76.2 |
Garriges |
C |
1909.9 ± 898.0 |
1881.8 ± 381.3 |
— |
— |
39.5 ± 2.1 |
155.8 ± 49.34 |
38.0 ± 10.2 |
4025.0 ± 123.9 |
E |
4316.3 ± 175.7 |
3694.1 ± 429.7 |
— |
— |
65.4 ± 0.7 |
181.5 ± 0.7 |
55.9 ± 0.5 |
8313.2 ± 121.5 |
Atocha |
C |
2832.1 ± 513.5 |
2728.2 ± 233.3 |
— |
— |
49.2 ± 13.8 |
500.5 ± 51.4 |
201.1 ± 37.2 |
6310.8 ± 105.3 |
E |
3699.3 ± 190.6 |
4234.4 ± 250.1 |
— |
— |
48.1 ± 9.3 |
603.5 ± 26.4 |
215.9 ± 21.1 |
8801.3 ± 99.5 |
Avellanera |
C |
2140.7 ± 35.8 |
1802.5 ± 65.0 |
42.9 ± 6.1 |
20.4 ± 1.6 |
— |
285.2 ± 10.1 |
268.2 ± 3.1 |
4274.7 ± 45.4 |
E |
3643.6 ± 186.6 |
2050.1 ± 130.6 |
38.5 ± 6.4 |
14.7 ± 1.3 |
— |
256.8 ± 82.2 |
308.9 ± 89.2 |
6312.5 ± 82.7 |
Concerning the concentration changes, as happened in the first experiment, the 9-series of F1t-PhytoPs represented the dominant PhytoPs. In general, the type of agricultural system produced a higher or lower total PhytoPs concentration depending on the almond cultivar. For example, total PhytoPs were higher in ‘Marcona’ and ‘Largueta’ when these trees were grown under the conventional system. However, ‘Planeta’, ‘Colorada’, ‘Atocha’, ‘Garriges’, and ‘Avellanera’ showed higher PhytoPs concentrations under the ecological system. The highest total PhytoPs concentration was found in ‘Marcona’ under the conventional system (15.2 ng per 100 g); this cultivar also had the highest value under the ecological system (10.7 ng per 100 g). In general, adding together all the PhytoPs from the different cvs, the ecological system produced an increase in PhytoPs versus the conventional system (57.8 vs. 53.9 ng per 100 g).
Concerning the detection of new PhytoPs, the ecological system led to the synthesis of the 9-series of D1t-PhytoPs in cv. ‘Planeta’ (Fig. 1). It must be remembered that in the first experiment, under the conventional system, these PhytoPs were detected only in ‘Colorada’ and ‘Avellanera’; whereas ‘Avellanera’ also synthesized these PhytoPs under the ecological system, in ‘Colorada’ they were not detected. In addition, the 16-series of F1t-PhytoPs was detected in ‘Colorada’ under ecological conditions but not under the conventional system. However, this group of compounds was not detected in cv. ‘Avellanera’, for either system.
3.3 Experiment 3: comparison of rain-fed conditions and irrigation under the conventional system, for cv. ‘Garriges’
As happened in experiment 2, two differences in concentration and PhytoP detection were found between the rain-fed and irrigated systems. Kernels from rain-fed almond trees had the lowest individual and total PhytoPs concentrations, but the scarcity of water led to the detection of the 16-series of F1t-PhytoPs, which was not found in the kernels from irrigated almond trees (Table 4). The concentration of total PhytoPs in almonds from rain-fed trees was 4025 ± 118 ng per 100 g, versus 5153 ± 112 ng per 100 g under irrigated conditions. These concentrations were significant in from B1- and L1-PhytoP types.
Table 4 Phytoprostanes (ng per 100 g) in cultivar ‘Garriges’ grown under rain-fed or irrigation conditions and conventional system
Water conditions |
9-F1t-PhytoP |
9-epi-9-F1t-PhytoP |
9-epi-9-D1t-PhytoP |
9-D1t-PhytoP |
ent-16-epi-16-F1t-PhytoP + ent-16-F1t-PhytoP |
16-B1-PhytoP |
9-L1-PhytoP |
Total |
Irrigation |
1978.1 ± 122.2 |
2867.8 ± 302.6 |
— |
— |
— |
227.5 ± 13.9 |
79.3 ± 9.6 |
5152.7 ± 112.1 |
Rain-fed |
1909.9 ± 175.7 |
1881.8 ± 381.3 |
— |
— |
39.5 ± 2.1 |
155.8 ± 19.3 |
38.0 ± 10.2 |
4025.1 ± 117.7 |
4. Discussion
To the best of our knowledge, no information has been published before on the content of PhytoPs in almonds. In previous studies, GC-MS was used to measure the concentrations of several classes of PhytoPs in tissues of various plant species for instance, in tomato leaves, peppermint leaves, lime tree flowers, birch pollen, birch leaves, and valerian root2,7 where the concentration ranged from 43 to 1380 ng g−1 DW. In addition, using this method, several classes of PhytoPs have also been measured in edible oils (linkernel, soybean, olive, walnut, rape kernel, grape) and their concentration was around 1057 ng mL−.22 Other studies have also detected them in cell cultures of different types of plants such as Nicotiana tabacum (Solanaceae), Glycine max (Fabaceae), Rauvolfia serpentina (Apocynaceae), Agrostis tenuis (Poaceae), Salix alba, Arabidopsis thaliana, Tilia cordata, and Betula pendula in the range of 11 to 131 ng g−1 DW.5,23 It is important to highlight that this method, used in these studies, was not able to quantify individual PhytoPs but rather the types of PhytoPs. In addition, the method used previously has a drawback: it is very slow, since the PhytoPs must be subjected to derivatization reactions previous to the final analysis. Therefore, in order to solve this handicap, several studies have been carried out recently with a new method which uses individual, synthetic standards and UHPLC-MS/MS.15 This new method showed that olive oil and sunflower oil had a total PhytoPs concentration of between 15 and 39 ng mL−1 and around 298 ng mL−1, respectively,15 while algae from several species had a total PhytoPs content that oscillated between 6 and 1381 ng per 100 g of dry algae.24 Also, in raw ‘Manzanilla de Sevilla’ green table olive fruits, the total PhytoPs content was 581–999 ng per 100 g, increasing to 5888–8786 ng per 100 g f.w. when the olives were conserved in the “Spanish” style.25 In this current work, the total PhytoPs content in the almond kernels was in the range of 4.0 to 23.8 ng per 100 g (Tables 2–4) higher than in dry algae, raw olive fruits, and olive oil and in the range of refined sunflower oil and table olives conserved in the “Spanish” style. This indicates that the oxidative stress (OS) suffered by the almonds was similar to that to which the plant products were subjected in their industrial processing. This could be due to the use of fertilizers and the phytosanitary treatment used in the cultivation of the different varieties of almond.
Concerning the PhytoP series, the 9-series of F1t-PhytoPs dominated in this study and was identified in all the almond cvs. The F1-PhytoPs series has been shown to occur predominately esterified in plant lipids, and plant membranes are the storage site of this type of PhytoP showing that it is in the first line of defense of the plant.26 The 9-series of L1-PhytoPs was a minor component when the 9-series of D1t-PhytoPs was not detected (only found in cvs Colorada and Avellanera). Collado-González et al.25 and Barbosa et al.24 also found that the 9-series of F1t-PhytoPs was the dominant PhytoPs class in the green table olive ‘Manzanilla de Sevilla’ and in edible macroalgae. Although in olive oils the dominant PhytoP class was the 9-series of D1-PhytoPs,15 all of these results agree with those of Karg et al.,22 who reported that in all the oils studied either D1-PhytoPs or F1-PhytoPs were the dominant PhytoP class, the B1-PhytoPs class being a minor component. However, in refined sunflower oil the major PhytoP class was the 16-series of B1-PhytoPs. This could be due to the harsh treatment during the refining of sunflower oil, which includes a dramatic temperature treatment and could induce more cross oxidation reactions of ALA at 100 °C or 240 °C.15
The results obtained in our study show that the qualitative and quantitative PhytoP profiles varied greatly among cultivars from the same species. For example, the total PhytoPs content of cv. Blanqueta was about 4.5 fold higher than that of Garriges, when both grew in a rain-fed, conventional system (Table 2). This agrees with Barbosa et al.,24 who reported that PhytoPs were widely distributed among 24 macroalgae species (brown, green, and red), varying greatly between the three phyla and even between species from the same genus. Collado-González et al.15 also found differences in the PhytoPs profiles of 0.8° extra virgin olive oil, 0.4° olive oil, and refined sunflower oil.
Another interesting finding, in cvs ‘Colorada’ and ‘Avellanera’ under the same growing conditions, was the detection of the 9-series of D1t-PhytoPs and the absence of the 16-series of F1t-PhytoPs which was found in the rest of the cvs. This variation in the qualitative and quantitative PhytoP profiles also depended on the agricultural system or irrigation used (Fig. 1 and Table 3 and 4). The ecological system promoted the synthesis of the 9-series of D1t-PhytoPs in ‘Planeta’ (Fig. 1) and the 16-series of F1t-PhytoPs in ‘Colorada’ under ecological conditions but not under the conventional system. Some cvs showed a higher total PhytoP content under conventional cultivation while others did so in the ecological system. This could be ascribed to the decisive effect of the cultivar, agricultural system, and/or interaction between these two factors, which can make these almonds prone to autoxidation. The PhytoP content and composition depended primarily on the genotype but also were affected by factors such as the agricultural system (conventional or ecological). Barbosa et al.24 observed, in macroalgae, large variations in the individual and total PhytoP contents which they attributed to the availability of ALA, along with inter and/or intra specific variations and other environmental factors. Indeed, almonds are rich sources of lipids (25 to 66 g per 100 g f.w.), oleic and linoleic acids accounting for about 90% of the total lipids, with very low saturated fatty acid levels (<10%) and very low concentrations of ALA (<0.1%).27 Other minor components characterized in almonds include tocopherols (about 450 µg g−1 oil), sterols (2200 µg g−1), and squalene (95 µg g−1).28,29 However, the chemical composition of almonds varies tremendously within the species, according to environmental variability, geographical origin, temperature, and irrigation.30–34 Exposure to these diverse abiotic factors has an influence on PUFA levels in almonds. Therefore, considering that the only requirement for PhytoP formation is the presence of ALA and molecular oxygen, the variability observed in the PhytoP signature could be explained by the magnitude of the effect of external factors, such as the climatic condition of the year, which probably depends on the genetic background of each cultivar showing that this phenotypic variability has a genetic basis.4,24,35,36 However, Karg et al.22 did not find a correlation between the ALA and PhytoP contents. Therefore, this genetic difference could be due to difference in lipase activity and/or membrane lipids stability, since the PhytoPs are generated by free radical catalyzed oxidation of esterified linolenate in membranes in situ and can be released preformed by lipases.2,26
Concerning the irrigation factor, the rain-fed almond trees showed lower individual and total PhytoP concentrations than those under irrigation, even though the rain-only treatment gave rise to the detection of the 16-series of F1-PhytoPs which was not found in irrigated almonds (Table 4). However, Collado-González et al.25 recently reported that the total PhytoP content in raw olive fruits increased as a result of regulated deficit irrigation, probably due to the fact that one of the inevitable consequences of drought stress is enhanced ROS production in the different cellular compartments. One consequence of this enhanced ROS formation in plants is the formation of an array of lipid peroxidation products, including structural congeners of jasmonates, the PhytoPs.5 However, in this sense, the average olive fruit yield was not affected by water restriction, while in our experiment irrigation increased the almond yield, from 400 kg ha−1 (rain-fed) to 1000 kg ha−1, as other authors have previously reported.37,38 Collado-González et al.25 irrigated olive trees but applied a pronounced restriction of irrigation during pit hardening. In our case, the rain-fed almond trees could be adapted to this common, no-irrigation situation, resulting in a low enhancement of ROS formation. In addition, the α-tocopherol content seems to be the most important contributor to both the radical scavenging activity and the almond oxidative stability.33 Tocopherols are believed to have a diversity of physiological, biological, and biochemical functions, mainly due to their action as antioxidants but also by acting as membrane stabilizers.39 Since there is an inverse relationship between watering and oil content (the oil content in ‘Garriges’ increased from 48.6% under irrigation to 56.1% when rain-fed),40 the lower PhytoP content in the kernels of rain-fed almond trees could be explained as the secondary substrate to be oxidized being α-tocopherol, if this is the most important one and the most abundant under rain-fed conditions.
5. Conclusion
This study represents a first analysis of the quantitative and qualitative PhytoP profile in almond cvs under different agronomic conditions. The total PhytoP content in almond kernels was in the range of 4.0 to 23.8 ng per 100 g. The F1-PhytoPs predominated and were identified in all the almond cvs. The PhytoP profile varied greatly according to the genotype, but also was affected by factors such as the agricultural system (conventional or ecological) and irrigation.
6. Abbreviations
ALA | α-Linolenic acid |
AA | Arachidonic acid |
BHA | 2,6-Di-tert-butyl-4-methylphenol |
ETo | Annual reference evapotranspiration from a hypothetical grass reference crop with specific characteristics, without water shortage |
GC-MS | Gas chromatography coupled to mass spectrometry |
IS | Internal standard |
MRM | Multiple reaction monitoring |
OS | Oxidative stress |
PG | Prostaglandin |
PhytoP | Phytoprostane |
PUFA | Polyunsaturated fatty acid |
RSD | Relative standard deviation |
ROS | Reactive oxygen species |
SPE | Solid-phase extraction |
UHPLC-QqQ-MS/MS | Ultra-high performance liquid chromatography coupled to triple quadrupole mass spectrometry |
Acknowledgements
The authors are grateful to the COATO company (Totana, Murcia, Spain) for supplying all the almond cultivars, to the Institute of Plant Biotechnology of the UPCT for the use of equipment, and to MICINN (CICYT AGL2010-19201-C04-02-AGR and AGL2013-23690). JC-G was funded by an FPI grant.
References
- J. D. Morrow, T. A. Minton and L. J. Roberts, 2nd The F2-isoprostane, 8-epi-prostaglandin F2 alpha, a potent agonist of the vascular thromboxane/endoperoxide receptor, is a platelet thromboxane/endoperoxide receptor antagonist, Prostaglandins, 1992, 44, 155–163 CrossRef CAS.
- R. Imbusch and M. J. Mueller, Formation of isoprostane F2-like compounds (phytoprostanes F1) from α-linolenic acid in plants, Free Radical Biol. Med., 2000, 28, 720–726 CrossRef CAS.
- M. Zoeller, N. Stingl, M. Krischke, A. Fekete, F. Waller, S. Berger and M. J. Mueller, Lipid profiling of the arabidopsis hypersensitive response reveals specific lipid peroxidation and fragmentation processes: biogenesis of pimelic and azelaic acid, J. Plant Physiol., 2012, 160, 365–378 CrossRef CAS PubMed.
- M. H. Cruz de Carvalho, Drought stress and reactive oxygen species: Production, scavenging and signaling, Plant Signaling Behav., 2008, 3, 156–165 CrossRef.
- I. Thoma, C. Loeffler, A. Sinha, M. Gupta, M. Krischke, B. Steffan, T. Roitsch and M. J. Mueller, Cyclopentenone isoprostanes induced by reactive oxygen species trigger defense gene activation and phytoalexin accumulation in plants, Plant J., 2003, 34, 363–375 CrossRef CAS.
- E. E. Farmer and M. J. Mueller, ROS-Mediated lipid peroxidation and res-activated signaling, Annu. Rev. Plant Biol., 2013, 64, 429–450 CrossRef CAS PubMed.
- R. Imbusch and M. J. Mueller, Analysis of oxidative stress and wound-inducible dinor isoprostanes F(1) (phytoprostanes F(1)) in plants, J. Plant Physiol., 2000, 124, 1293–1303 CrossRef CAS PubMed.
- M. J. Mueller, Radically novel prostaglandins in animals and plants: the isoprostanes, Chem. Biol., 1998, 5, R323–R333 CrossRef CAS.
- N. A. Eckardt, Oxylipin signaling in plant stress responses, Plant Cell, 2008, 20, 495–497 CrossRef CAS PubMed.
- I. Thoma, M. Krischke, C. Loeffler and M. J. Mueller, The isoprostanoid pathway in plants, Chem. Phys. Lipids, 2004, 128, 135–148 CrossRef CAS PubMed.
- J. C. King, J. Blumberg, L. Ingwersen, M. Jenab and K. L. Tucker, Tree nuts and peanuts as components of a healthy diet, J. Nutr., 2008, 138, 1736S–1740S CAS.
- P. M. Kris‐Etherton, K. D. Hecker and A. E. Binkoski, Polyunsaturated fatty acids and cardiovascular health, Nutr. Rev., 2004, 62, 414–426 CrossRef PubMed.
- S. Ahrens, M. Venkatachalam, A. M. Mistry, K. Lapsley and S. K. Sathe, Almond (Prunus dulcis L.) protein quality, Plant Foods Hum. Nutr., 2005, 60, 123–128 CrossRef CAS PubMed.
- A. Scalbert and G. Williamson, Dietary intake and bioavailability of polyphenols, J. Nutr., 2000, 130, 2073S–2085S CAS.
- J. Collado-González, S. Medina, T. Durand, A. Guy, J. M. Galano, A. Torrecillas, F. Ferreres and A. Gil-Izquierdo, New UHPLC-QqQ-MS/MS method for quantitative and qualitative determination of free phytoprostanes in foodstuffs of commercial olive and sunflower oils, Food Chem., 2015, 178, 212–220 CrossRef PubMed.
- F. Lavedrine, A. Ravel, A. Villet, V. Ducros and J. Alary, Mineral composition of two walnut cultivars originating in France and California, Food Chem., 2000, 68, 347–351 CrossRef CAS.
- J. Parcerisa, M. Rafecas, A. I. Castellote, R. Codony, A. Farrán, J. Garcia, C. González, A. López, A. Romero and J. Boatella, Influence of variety and geographical origin on the lipid fraction of hazelnuts (Corylus avellana L.) from Spain: (III) oil stability, tocopherol content and some mineral contents (Mn, Fe, Cu), Food Chem., 1995, 53, 71–74 CrossRef CAS.
- E. Pinot, A. Guy, A. Fournial, L. Balas, J. C. Rossi and T. Durand, Total synthesis of the four enantiomerically pure diasteroisomers of the phytoprostanes E1type II and of the 15-E2t-isoprostanes, J. Org. Chem., 2008, 73, 3063–3069 CrossRef CAS PubMed.
- S. El Fangour, A. Guy, V. Despres, J. P. Vidal, J. C. Rossi and T. Durand, Total syntheses of the eight diastereoisomers of the syn-Anti-syn phytoprostanes F1 types I and II, J. Org. Chem., 2004, 69, 2498–2503 CrossRef CAS PubMed.
- S. El Fangour, A. Guy, J. P. Vidal, J. C. Rossi and T. Durand, A flexible synthesis of the phytoprostanes B1 type I and II, J. Org. Chem., 2005, 70, 989–997 CrossRef CAS PubMed.
- S. Medina, R. Dominguez-Perles, J. I. Gil, F. Ferreres, C. Garcia-Viguera, J. M. Martínez-Sanz and A. Gil Izquierdo, An ultra-pressure liquid chromatography/triple quadrupole tandem mass spectrometry method for the analysis of 13 eicosanoids in human urine and quantitative 24 hour values in healthy volunteers in a controlled constant diet, Rapid Commun. Mass Spectrom., 2012, 26, 1249–1257 CrossRef CAS PubMed.
- K. Karg, V. Dirsch, A. M. Vollmar, J. L. Cracowski, F. Laporte and M. J. Mueller, Biologically active oxidized lipids (phytoprostanes) in the plant diet and parenteral lipid nutrition, Free Radical Res., 2007, 41, 25–37 CrossRef CAS PubMed.
- S. Parchmann and M. J. Mueller, Evidence for the formation of dinor isoprostanes E1 from alpha-linolenic acid in plants, J. Biol. Chem., 1998, 273, 32650–32655 CrossRef CAS PubMed.
- M. Barbosa, J. Collado-González, P. B. Andrade, F. Ferreres, P. Valentão, J. M. Galano, T. Durand and A. Gil-Izquierdo, Non-enzymatic α-linolenic acid derivatives from the sea: macroalgae as novel sources of phytoprostanes, J. Agric. Food Chem., 2015 Search PubMed , in press.
- J. Collado-González, T. Durand, F. Ferreres, S. Medina, A. Torrecillas and Á. Gil-Izquierdo, Phytoprostanes, Lipid Technol., 2015, 27(5) DOI:10.1002/lite.201500020.
- T. Durand, V. Bultel-Poncé, A. Guy, S. Berger, M. J. Mueller and J. M. Galano, New bioactive oxylipins formed by non-enzymatic free-radical-catalyzed pathways: the phytoprostanes, Lipids, 2009, 44, 875–888 CrossRef CAS PubMed.
- S. Yada, K. Lapsley and G. Huang, A review of composition studies of cultivated almonds: Macronutrients and micronutrients, J. Food Compos. Anal., 2011, 24, 469–480 CrossRef CAS PubMed.
- M. Kornsteiner, K. H. Wagner and I. Elmadfa, Tocopherols and total phenolics in 10 different nut types, Food Chem., 2006, 98, 381–387 CrossRef CAS PubMed.
- C. M. López-Ortiz, S. Prats-Moya, A. B. Sanahuja, S. E. Maestre-Pérez, N. Grané-Teruel and M. L. Martín-Carratalá, Comparative study of tocopherol homologue content in four almond oil cultivars during two consecutive years, J. Food Compos. Anal., 2008, 21, 144–151 CrossRef PubMed.
- O. Kodad, J. M. Alonso, M. T. Espiau, G. Estopañán and T. Juan, Chemometric characterization of almond germplasm: compositional aspects involved in quality and breeding, J. Am. Soc. Hortic. Sci., 2011, 136, 273–281 CAS.
- O. Kodad, G. Estopañán, T. Juan, J. Alonso, M. Espiau and R. Socias i Compan, Oil content, fatty acid composition and tocopherol concentration in the Spanish almond genebank collection, Sci. Hortic., 2014, 177, 99–107 CrossRef CAS PubMed.
- O. Kodad, I. Socias, R. Company, M. Prats and M. Loópez Ortiz, Variability in tocopherol concentrations in almond oil and its use as a selection criterion in almond breeding, J. Hortic. Sci. Biotechnol., 2006, 81, 501–507 Search PubMed.
- D. Maestri, M. Martínez, R. Bodoira, Y. Rossi, A. Oviedo, P. Pierantozzi and M. Torres, Variability in almond oil chemical traits from traditional cultivars and native genetic resources from Argentina, Food Chem., 2015, 170, 55–61 CrossRef CAS PubMed.
- S. Yada, G. Huang and K. Lapsley, Natural variability in the nutrient composition of California-grown almonds, J. Food Compos. Anal., 2013, 30, 80–85 CrossRef CAS PubMed.
- A. Cutignano, N. Lamari, E. Manzo, G. Cimino and A. Fontana, Lipoxygenase products in marine diatoms: a concise analytical method to explore the functional potential of oxylipins1, J. Phycol., 2011, 47, 233–243 CrossRef CAS PubMed.
- C. Loeffler, S. Berger, A. Guy, T. Durand, G. Bringmann, M. Dreyer, U. Von Rad, J. Durner and M. J. Mueller, B1-phytoprostanes trigger plant defense and detoxification responses, Plant Physiol., 2005, 137, 328–340 CrossRef CAS PubMed.
- G. Egea, M. M. González-Real, A. Baille, P. A. Nortes, P. Sánchez-Bel and R. Domingo, The effects of contrasted deficit irrigation strategies on the fruit growth and kernel quality of mature almond trees, Agric. Water Manage., 2009, 96, 1605–1614 CrossRef PubMed.
- G. Egea, P. A. Nortes, M. M. González-Real, A. Baille and R. Domingo, Agronomic response and water productivity of almond trees under contrasted deficit irrigation regimes, Agric. Water Manage., 2010, 97, 171–181 CrossRef PubMed.
- A. Azzi and A. Stocker, Vitamin E: non-antioxidant roles, Prog. Lipid Res., 2000, 39, 231–255 CrossRef CAS.
- F. Romojaro and T. Berenguer, L'influence de la varieté et de l'irrigation sur la teneur en huile des fruits de l'amandier, in Proceedings of the V GREMPA seminar on pistachios and almonds, Options mediterranees, Sfax, Tunisia, Serie Etudes, 1984, pp. 185–188 Search PubMed.
|
This journal is © The Royal Society of Chemistry 2015 |
Click here to see how this site uses Cookies. View our privacy policy here.