DOI:
10.1039/C5RA06210A
(Paper)
RSC Adv., 2015,
5, 51020-51026
Design, synthesis and biological evaluation of soluble 2,5-diketopiperazines derivatives as potential antifouling agents†
Received
8th April 2015
, Accepted 3rd June 2015
First published on 3rd June 2015
Abstract
A small library of soluble 2,5-diketopiperazine derivatives were designed, synthesized and evaluated as antifouling agents against two species of marine organisms: Balanus amphitrite and Bugula neritina by larval attachment assay. The results showed that different derivatives had different antifouling activities. Among these compounds, 3a (EC50 = 2.5 μg mL−1), 3b (EC50 = 3.2 μg mL−1) and 3d (EC50 = 1.6 μg mL−1) had strong activities, and 2d (EC50 = 20.5 μg mL−1), 3l (EC50 = 25.0 μg mL−1) and 3m (EC50 = 18.5 μg mL−1) had moderate activities against Balanus amphitrite, but other compounds had no activities. Differently, compounds 3d (EC50 = 3.1 μg mL−1) and 3i (EC50 = 2.3 μg mL−1) exhibited strong activities, and 3a (EC50 = 11.3 μg mL−1) and 3c (EC50 = 17.2 μg mL−1) showed moderate activities against Bugula neritina, whereas other compounds had no activities. All of the active compounds had stronger antifouling activities than those of the natural product cyclo-(L-Phe-L-Pro). Compound 3d could be a new template molecule for further development as an antifouling agent because of its strong inhibitory activities against both species of fouling organisms with low or no toxicity to the environment.
1. Introduction
It is well known that biofouling has a seriously negative influence on marine-related activities, and a number of effective metal-based (such as copper(I) compounds and organotins) polymer antifouling coatings have been used to inhibit the attachment of marine organisms (e.g. Barnacle, Bugula neritina, Mussel, and Seaweed) for a long time.1 However due to their high toxicity to the environment, they were banned or reduced in use by IMO (International Maritime Organization) in recent years,2,3 thus new methods for developing environmentally friendly antifoulants needs to be explored urgently. Although a few papers2,4 recently reviewed that natural products showed patency as antifoulants with low or even no harm to the environment, it might yet be a dilemma because of their difficult availability.5 Fortunately, this problem might be solved via the synthesis or optimization of the active natural products which are used as the lead compounds.6–10
2,5-Diketopiperazine derivatives (Fig. 1) are not only a class of naturally occurring privileged structures from fungi, bacteria, the plant kingdom, and mammals, but they also have several characteristics that make them attractive scaffolds for drug discovery.11,12 Several previous articles showed that cyclo-(L-Phe-L-Pro) (Fig. 1) or its homologues which were isolated from marine-derived fungi had moderate activities to inhibit the settlement of barnacles,13,14 and some other modified 2,5-diketopiperazine derivatives (Fig. 1) also showed effective antifouling activities to these sessile marine organisms.15 These results indicate that the small molecule 2,5-diketopiperazine will be a suitable scaffold for further development in search for high potential antifouling agents. On the other hand, 2,5-diketopiperazine derivatives which have no other substituent on the amide nitrogen atoms except the hydrogen atoms, especially for those with their aromatic groups substituted at 3 and/or 6-positions on its skeleton, show poor solubility because of the existence of intermolecular hydrogen bonds and π–π stacking,16,17 thus result in negative impacts on their antifouling activity.18 Therefore, in the present paper, a small library of soluble 2,5-diketopiperazine derivatives, with the hydrogen atoms on the amides replaced by an acetyl group or/and an allyl group, were designed and synthesized (Fig. 2) and their antifouling activities against two species of marine fouling organisms Balanus amphitrite and Bugular neritina, were evaluated aiming to discover more effective 2,5-diketopiperazine derivatives that can be used as environmentally friendly antifouling agents.
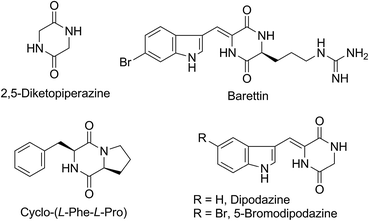 |
| Fig. 1 2,5-Diketopiperazine and its marine natural products or synthetic derivatives. | |
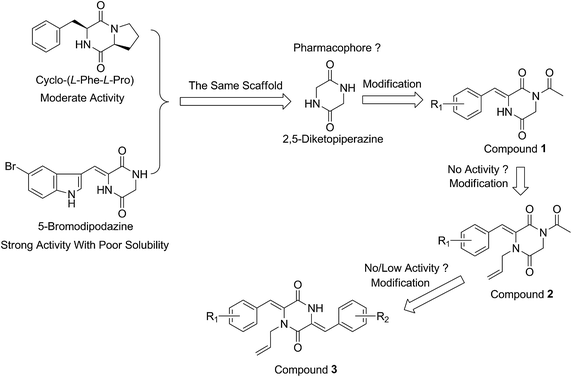 |
| Fig. 2 Designing of 2,5-diketopiperazine derivatives used as antifouling agents. | |
2. Results and discussion
2.1. Synthesis of 2,5-diketopiperazine derivatives
According to our previous study,16 the synthesis of 2,5-diketopiperazine derivatives was as follows: 1,4-diacetyl-2,5-diketopiperazine and aromatic aldehydes were stirred with Cs2CO3 in N,N-dimethylformamide (DMF) at room temperature to yield compound 1, which was then kept together with allyl bromide and K2CO3 in DMF to get compound 2, and the final compound 3 was obtained by mixing compound 2, aromatic aldehydes, and Cs2CO3 in DMF at atmosphere until the reaction was completed (Scheme 1). Among all the derivatives, compounds 1a–b, 1d, 2a–b, 2d, 3a–b, 3e, and 3h–i were synthesized in our previous article16 and were screened directly to their antifouling activities, and other compounds were synthesized for the first time in the present paper. Compound 1 can be easily dissolved in dimethylsulfoxide (DMSO) and DMF, but has low solubility in ethyl acetate (AcOEt), dichloromethane (DCM), chloroform, methanol (MeOH), ethanol (EtOH), etc. Compounds 2 and 3 can be dissolved in all of the above solvents.
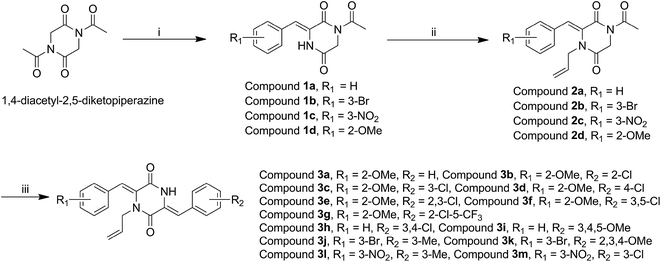 |
| Scheme 1 Synthesis of 2,5-diketopiperazine derivatives. Regents and conditions: (i). 1,4-diacetyl-2,5-diketopiperazine (1.5 equiv.), aromatic aldehyde (1.0 equiv.), Cs2CO3 (1.5 equiv.), DMF, rt; (ii). allyl bromide (1.2 equiv.), K2CO3 (2.0 equiv.), DMF, rt; (iii). aromatic aldehyde (1.2 equiv.), Cs2CO3 (1.5 equiv.), DMF, rt. | |
2.2. Antifouling activity
2.2.1. Anti-attachment activity of 2,5-diketopiperazine derivatives against Balanus amphitrite. Although a few researchers suggested that an antifouling compound with an EC50 value less than 5.0 μg mL−1 could be considered as a candidate,4 in this study, each sample with a concentration of 25.0 μg mL−1, was preliminary screened in anti-larval settlement assays.19,20 As a consequence of the experiments, only these compounds which had obvious inhibitory activities at this concentration would be selected for further studies. Since the 2,5-diketopiperazine derivatives with no substituent on the amide nitrogen atoms usually have poor solubility, four derivatives were designed and synthesized by introducing some aromatic aldehydes with different electrical properties at 3-positions and an acetyl group at 1-position on the 2,5-diketopiperazine scaffold. Among these derivatives, compound 1a contains a phenyl (Ph) group, 1b contains a 3-BrPh group, 1c contains an electron-withdrawing 3-NO2Ph group, and 1d contains an electron-donating 2-OMePh group, respectively. The antifouling results showed that these derivatives had no activity to inhibit the larval attachment of Balanus amphitrite (Table 1). So another four derivatives (2a, 2b, 2c, and 2d) with an allyl group on the other nitrogen atom were synthesized, and the anti-attachment assays showed that compounds 2a, 2b, and 2c still had no inhibitory activities, whereas surprisingly, compound 2d (EC50 = 20.5 μg mL−1) displayed a little stronger activity than that of cyclo-(L-Phe-L-Pro) (EC50 = 68.6 μg mL−1) (see ref. 13 and 14), which indicated that 2-OMe might be an optimal moiety and beneficial to its antifouling ability. As the chloride atom is common in a large number of secondary metabolites and plays an important role in the bioactivity,21,22 so compound 2d was selected as a template molecule, and other aromatic aldehydes possessing one or two chloride atoms were chosen to be another substituting unit on 6-position of the 2,5-diketopiperazine scaffold, thus six soluble 2,5-diketopiperazine derivatives (3b–g), combined with one derivative (3a) modified by a benzaldehyde group, were designed and synthesized. Their antifouling results against Balanus amphitrite are shown in Table 1. From Table 1, we could see that compound 3a (EC50 = 2.5 μg mL−1) had strong activity, which was the same as to compounds 3b (EC50 = 3.2 μg mL−1) and 3d (EC50 = 1.6 μg mL−1), and there were no larval death even at the concentration of 25.0 μg mL−1 and the lowest LC50/EC50 ratio of these three compounds was higher than 5.0, indicating that these derivatives were non-toxic or low-toxic.19 While compounds 3c with a 3-ClPh group, 3e with a 3,4-ClPh group, 3f with a 3,5-ClPh group, and 3g with a 2-Cl-5-CF3Ph group had no activity, which implied the number of chloride atoms and the substitutive position on the phenyl ring were both important to their antifouling activities.23
Table 1 Anti-settlement activities of the synthetic 2,5-diketopiperazine derivatives and compound cyclo-(L-Phe-L-Pro) against Balanus amphitrite
Compounds |
Balanus amphitrite |
EC50a (μg mL−1) |
LC50b (μg mL−1) |
LC50/EC50 |
EC50: the concentration of a compound that inhibited larval attachment by 50% in comparison with the control. LC50: the concentration that caused 50% larval mortality in testing wells in comparison with the control. —: undetectable. Ref. 13 and 14. The concentrations (EC50 values) are denoted as the means ± SD (for n = 3). |
Cyclo-(L-Phe-L-Pro) |
>25 |
>25 |
—c |
68.6 |
115 |
= 1.7d |
1a |
>25 |
>25 |
— |
1b |
>25 |
>25 |
— |
1c |
>25 |
>25 |
— |
1d |
>25 |
>25 |
— |
2a |
>25 |
>25 |
— |
2b |
>25 |
>25 |
— |
2c |
>25 |
>25 |
— |
2d |
20.5 ± 1.8e |
>25 |
>1.2 |
3a |
2.5 ± 0.03 |
>25 |
>10.0 |
3b |
3.2 ± 0.4 |
>25 |
>7.8 |
3c |
>25 |
>25 |
— |
3d |
1.6 ± 0.01 |
>25 |
>15.6 |
3e |
>25 |
>25 |
— |
3f |
>25 |
>25 |
— |
3g |
>25 |
>25 |
— |
3h |
>25 |
>25 |
— |
3i |
>25 |
>25 |
— |
3j |
>25 |
>25 |
— |
3k |
>25 |
>25 |
— |
3l |
25.0 ± 2.5 |
>25 |
= 1.0 |
3m |
18.5 ± 1.3 |
>25 |
>1.4 |
In order to obtain more information of antifouling abilities about other substituted aromatic aldehyde, another six soluble derivatives (3h–m) were also synthesized, and their antifouling abilities to inhibit the larval attachment of Balanus amphitrite were determined, and the results showed that compounds 3h–k had no activities except 3l and 3m, which had moderate antifouling activities with the EC50 values of 25.0 and 18.5 μg mL−1, respectively (Table 1). These results demonstrated that 3-NO2Ph might be another effective group used as a derived molecule in exploring antifouling agents, nevertheless, in comparison to the former derivatives which contained one 2-OMePh group, their antifouling effectiveness is weaker.
2.2.2. Anti-settlement activity of 2,5-diketopiperazine derivatives against Bugula neritina. The antifouling abilities to inhibit the settlement of Bugula neritina by the above synthesized compounds were also investigated and the results are listed in Table 2. Unlike their inhibition of the larval settlement of Balanus amphitrite, compound 3d (EC50 = 3.1 μg mL−1) had strong abilities to inhibit the attachment of Bugula neritina, however compound 3b had no activity. We were pleased to find that compound 3i exhibited strong activity with an EC50 value of 2.3 μg mL−1, and compounds 3a (EC50 = 11.3 μg mL−1) and 3c (EC50 = 17.2 μg mL−1) showed moderate activity against the attachment of Bugula neritin. Other compounds had no activity at a concentration of 25 μg mL−1. Similar to the toxicity of compounds 3a, 3b and 3d against Balanus amphitrite, there were no Bugula neritina death and the lowest LC50/EC50 ratio was more than 5.0 which indicated that compounds 3d and 3i were non-toxic or low-toxic.19 These results revealed that different marine organism species might show different sensitivities to their inhibitors and the activity and toxicity of the active derivatives might be combined in order to achieve better antifouling performance.
Table 2 Anti-settlement activities of the synthetic 2,5-diketopiperazine derivatives and compound cyclo-(L-Phe-L-Pro) against Bugula neritina
Compounds |
Bugula neritina |
EC50a (μg mL−1) |
LC50b (μg mL−1) |
LC50/EC50 |
EC50: the concentration of a compound that inhibited larval attachment by 50% in comparison with the control. LC50: the concentration that caused 50% larval mortality in testing wells in comparison with the control. —: undetectable. Ref. 13. The concentrations (EC50 values) are denoted as the means ± SD (for n = 3). |
Cyclo-(L-Phe-L-Pro) |
>25 |
>25 |
—c |
70.4 |
>200 |
>2.8d |
1a |
>25 |
>25 |
— |
1b |
>25 |
>25 |
— |
1c |
>25 |
>25 |
— |
1d |
>25 |
>25 |
— |
2a |
>25 |
>25 |
— |
2b |
>25 |
>25 |
— |
2c |
>25 |
>25 |
— |
2d |
>25 |
>25 |
— |
3a |
11.3 ± 0.9e |
>25 |
>2.2 |
3b |
>25 |
>25 |
— |
3c |
17.2 ± 0.8 |
>25 |
>1.5 |
3d |
3.1 ± 0.5 |
>25 |
>8.1 |
3e |
>25 |
>25 |
— |
3f |
>25 |
>25 |
— |
3g |
>25 |
>25 |
— |
3h |
>25 |
>25 |
— |
3i |
2.3 ± 0.1 |
>25 |
>10.9 |
3j |
>25 |
>25 |
— |
3k |
>25 |
>25 |
— |
3l |
>25 |
>25 |
— |
3m |
>25 |
>25 |
— |
2.3. Compound 3d hold promise as environmentally friendly antifouling agent
In a more intuitive comparison study, the EC50 values of the active synthesized compounds and cyclo-(L-Phe-L-Pro) to inhibit the larval attachment of Balanus amphitrite and Bugula neritina are shown in Fig. 3 and 4. From Fig. 3 and 4, it was found that all the active synthesized compounds had stronger inhibitory activity than that of cyclo-(L-Phe-L-Pro). These might be due to the higher liposolubility of the derivatives than that of cyclo-(L-Phe-L-Pro), thus resulted in the penetration of the former through tissues and reached the target more easily.15,24 Among all the active compounds, compound 3d had strong antifouling activities against both of the marine organisms as well as with low or no toxicity, and would be a new chemical template for the further development as environmentally friendly antifouling agent.
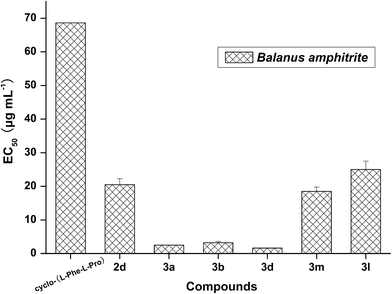 |
| Fig. 3 A comparison for the effect of the active derivatives against Balanus amphitrite. EC50 values of compounds 2d, 3a, 3b, 3d, 3m, 3l, and cyclo-(L-Phe-L-Pro) against Balanus amphitrite were 20.5 ± 1.8, 2.5 ± 0.03, 3.2 ± 0.4, 1.6 ± 0.01, 18.5 ± 1.3, 25.0 ± 2.5, and 68.6 (ref. 13 and 14) (μg mL−1), respectively, and the concentrations (EC50 values) are denoted as the means ± SD (for n = 3). | |
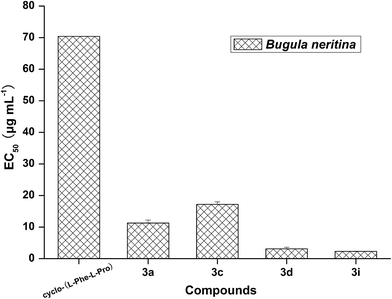 |
| Fig. 4 A comparison for the effect of the active derivatives against Bugula neritina. EC50 values of compounds 3a, 3c, 3d, 3i, and cyclo-(L-Phe-L-Pro) against Bugula neritina were 11.3 ± 0.9, 17.2 ± 0.8, 3.1 ± 0.5, 2.3 ± 0.1, and 70.4 (ref. 13) (μg mL−1), respectively, and the concentrations (EC50 values) are denoted as the means ± SD (for n = 3). | |
3. Conclusions
Inspired by the structure–activity relationships of the natural product cyclo-(L-Phe-L-Pro) and the synthesized compound 5-bromodipodazine, a small library of soluble 2,5-diketopiperazine derivatives were designed, synthesized and evaluated for their antifouling activities against Balanus amphitrite and Bugular neritina. The results showed that three compounds (3a, 3b, and 3d) had strong activities against Balanus amphitrite, and two compounds (3d and 3i) exhibited strong activities against Bugula neritina, in which compound 3d had strong activities against both of the marine organisms, and all these active compounds were low-toxic or non-toxic. These results confirmed that 2,5-diketopiperazine is a useful scaffold in search for effective antifouling agents, meanwhile, they also offer an example to illustrate that it is feasible that natural products or their optimized derivatives can indeed be used as antifouling candidates with low or no toxicity to the environment. Furthermore, compound 3d may be a new template molecule for further development as antifouling agents.
4. Experimental section
4.1. Chemistry
Melting points (m.p.) were determined by using a SRSO-ptiMelt automated melting point instrument without correction. The NMR spectra were recorded on a Bruker AC 500 NMR spectrometer with TMS as an internal standard. HR-ESI-MS data were measured on AQUITY UPLC/Q-TOF mass spectrometer. IR spectra were recorded on a Shimadzu IR Affinity-1 fourier transform infrared spectrometer. Materials for column chromatography were silica gel (200–300 mesh, Qingdao Marine Chemical Factory, Qingdao, China). All solvents were analytical grade. DMF was dried in CaH2 and distilled. 1,4-diacetyl-2,5-diketopiperazine was purchased from Sigma Aldrich, and others from Aladdin. All chemicals were used without further purification. Compounds 1a–b, 1d, 2a–b, 2d, 3b–c, 3e, and 3h–i were synthesized and characterized according to our previous article,16 and other compounds were synthesized as follows:
4.1.1. Synthesis of compound 1-acetyl-3-(3-nitrobenzylidene)piperazine-2,5-dione (1c). Cs2CO3 (1.5 equiv., 0.51 mmol, 166.0 mg) was added into the solution containing 1,4-diacetyl-2,5-diketopiperazine (1.5 equiv., 0.51 mol, 100.0 mg) and 3-nitrobenzaldehyde (1.0 equiv., 0.34 mmol, 51.0 mg) in dry DMF (2 mL), and the mixture was stirred at room temperature for about 5 hours. After the reaction was completed, the mixture was poured into crashed ice and the solid was filtered, washed with water for three times and dried. The target compound 1c was obtained as white solids with a yield of 82%. m.p. 197–200 °C, FT-IR (neat, cm−1): 3176, 1689, 1637, 1525, 1348, 1261, 1207, 796, 729; 1H NMR (500 MHz, DMSO-d6) δ = 10.72 (s, 1H, N–H), 8.37 (s, 1H, Ar-H), 8.17 (d, J = 10.0 Hz, 1H, Ar-H), 7.94 (d, J = 10.0 Hz, 1H, Ar-H), 7.70 (t, J = 10.0 Hz, 1H, Ar-H), 7.03 (s, 1H,
CH–H), 4.38 (s, 2H, –CH2–), 2.51 (s, 3H, –CH3); 13C NMR (125 MHz, DMSO-d6) δ = 171.92, 164.34, 160.97, 147.90, 136.14, 134.79, 130.01, 129.02, 123.90, 122.84, 115, 54, 45.87, 26.83; HRMS (ESI) (m/z) [M + H]+ for (C13H12N3O5) calculated 290.0771 found 290.0767; [M + Na]+ for (C13H11N3NaO5) calculated 312.0591 found 312.0590.
4.1.2. Synthesis of compound 1-acetyl-4-allyl-3-(3-nitrobenzylidene)piperazine-2,5-dione (2c). K2CO3 (2 equiv., 97 mg) was added into the solution containing compound 1c (1 equiv., 100 mg, 0.35 mmol) and allyl bromide (1.2 equiv., 0.42 mmol, 0.35 mL) in dry DMF (2 mL), and the mixture was stirred at room temperature overnight. After the reaction was completed, the mixture was poured into crashed ice and the solid was filtered, washed with water for three times and purified by silica gel chromatography. The target compound 2c was obtained as white solids with a yield of 55%. m.p. 80–83 °C, FT-IR (neat, cm−1): 1691, 1627, 1531, 1350, 1286, 1263, 1184; 1H NMR (500 MHz, CDCl3) δ = 8.23 (d, J = 10.0 Hz, 1H, Ar-H), 8.17 (s, 1H, Ar-H), 7.67–7.61 (m, 2H, Ar-H), 7.30 (s, 1H,
C–H), 5.54–5.46 (m, 1H,
C–H), 5.03 (d, J = 10.0 Hz, 1H,
CH–H), 4.69 (d, J = 20.0 Hz, 1H,
CH–H), 4.56 (s, 2H, –CH2–), 4.07 (d, J = 10.0 Hz, 2H, –CH2–), 2.63 (s, 3H, –CH3); 13C NMR (125 MHz, CDCl3) δ = 171.17, 164.54, 163.47, 148.29, 134.88, 134.55, 131.64, 130.67, 129.80, 124.13, 124.00, 122.98, 119.16, 46.74, 45.19, 26.72 ppm; HRMS (ESI) (m/z) [M + H]+ for (C16H16N3O5) calculated 330.1084 found 330.1082; [M + Na]+ for (C16H15N3NaO5) calculated 352.0904 found 352.0907.
4.1.3. General procedure for synthesis of compounds 3a, 3d, 3f–g, 3j–m. Cs2CO3 (1.5 equiv.) was added into the solution containing compound 2 (1.0 equiv., 50.0 mg) and aromatic aldehyde (1.2 equiv.) in dry DMF (1.5 mL), and the mixture was stirred at room temperature for about 5 hours. After the reaction was completed, the mixture was slowly poured into water and extracted with AcOEt for three times, and the organic layer was dried, filtered, and purified with silica gel column to afford the target compounds.
4.1.3.1. 1-Allyl-3-benzylidene-6-(2-methoxybenzylidene)piperazine-2,5-dione (3a). According to the general procedure, compound 3a was synthesized as a yellow solid with a yield of 56.3%. m.p. 68–80 °C, FT-IR (neat, cm−1): 3232, 1676, 1618, 1487, 1452, 1379, 1354, 1246, 1022, 752; 1H NMR (500 MHz, CDCl3) δ = 8.11 (s, 1H, N–H), 7.45–7.43 (m, 4H, Ar-H), 7.35 (t, J = 10.0 Hz, 2H, Ar-H), 7.32 (s, 1H, = C–H), 7.22 (d, J = 10.0 Hz, 1H, Ar-H), 7.06 (s, 1H,
C–H), 6.98 (t, J = 10.0 Hz, 1H,
C–H), 6.92 (d, J = 10.0 Hz, 1H, Ar-H), 5.55–5.51 (m, 1H,
C–H), 5.00 (d, J = 10.0 Hz, 1H,
CH–H), 4.76 (d, J = 20.0 Hz, 1H,
CH–H), 4.24 (d, J = 10.0 Hz, 2H, –CH2–), 3.85 (s, 3H, –OCH3); 13C NMR (125 MHz, CDCl3) δ = 160.04, 159.17, 157.30, 132.98, 131.41, 130.51, 130.34, 129.31, 128.67, 128.50, 128.28, 126.10, 122.78, 120.18, 118.33, 118.02, 117.17, 110.59, 55.42, 47.35 ppm; HRMS (ESI) (m/z) [M + H]+ for (C22H21N2O3) calculated 361.1547 found 361.1551; [M + Na]+ for (C22H20N2NaO3) calculated 383.1366 found 383.1371.
4.1.3.2. 1-Allyl-3-(4-chlorobenzylidene)-6-(2-methoxybenzylidene)piperazine-2,5-dione (3d). According to the general procedure, compound 3d was synthesized as a yellow solid with a yield of 62.3%. m.p. 135–136 °C, FT-IR (neat, cm−1): 3197, 1681, 1668, 1616, 1489, 1384, 1355, 1323, 1247, 1016, 752; 1H NMR (500 MHz, CDCl3) δ = 8.02 (s, 1H, N–H), 7.42 (d, J = 10.0 Hz, 2H, Ar-H), 7.38–7.36 (m, 3H, Ar-H), 7.34 (s, 1H,
C–H), 7.22 (d, J = 10.0 Hz, 1H, Ar-H), 6.98 (t, J = 10.0 Hz, 1H, Ar-H, 1H,
C–H), 6.93 (d, J = 5.0 Hz, 1H, Ar-H), 5.57–5.51 (m, 1H,
C–H), 5.01 (d, J = 10.0 Hz, 1H,
CH–H), 4.77 (d, J = 15.0 Hz, 1H,
CH–H), 4.23 (s, J = 5.0 Hz, 2H, –CH2–), 3.86 (s, 3H, –OCH3); 13C NMR (125 MHz, CDCl3) δ = 160.35, 159.04, 157.36, 134.56, 131.42, 131.33, 130.66, 130.37, 129.93, 129.53, 128.12, 126.46, 122.67, 120.23, 118.75, 118.19, 116.01, 110.64, 55.48, 47.41 ppm; HRMS (ESI) (m/z) [M + H]+ for (C22H20ClN2O3) calculated 395.1157 found 395.1153; [M + Na]+ for (C22H19ClN2NaO3) calculated 417.0976 found 417.0972.
4.1.3.3. 1-Allyl-3-(3,5-dichlorobenzylidene)-6-(2-methoxybenzylidene)piperazine-2,5-dione (3f). According to the general procedure, compound 3f was synthesized as a yellow solid with a yield of 32.7%. m.p. 186–188 °C, FT-IR (neat, cm−1): 3179, 1682, 1666, 1622, 1456, 1355, 1338, 1242, 1022, 754; 1H NMR (500 MHz, CDCl3) δ = 8.33 (s, 1H, N–H), 7.37 (t, J = 10.0 Hz, 1H, Ar-H), 7.31 (s, 2H, Ar-H), 7.29 (s, 1H, Ar-H; 1H,
C–H), 7.21 (d, J = 5.0 Hz, 1H, Ar-H), 6.99 (t, J = 10.0 Hz, 1H, Ar-H), 6.93 (d, J = 10.0 Hz, 1H, Ar-H), 6.90 (s, 1H,
C–H), 5.58–5.50 (m, 1H,
C–H), 5.01 (d, J = 10.0 Hz, 1H,
CH–H), 4.76 (d, J = 15.0 Hz, 1H,
CH–H), 4.23 (d, J = 10.0 Hz, 2H, –CH2–), 3.86 (s, 3H, –OCH3); 13C NMR (125 MHz, CDCl3) δ = 160.34, 158.57, 157.40, 136.07, 135.95, 131.21, 130.79, 130.37, 128.55, 128.01, 127.85, 126.79, 122.56, 120.28, 119.31, 118.31, 113.99, 110.68, 55.51, 47.49 ppm; HRMS (ESI) (m/z) [M + H]+ for (C22H19Cl2N2O3) calculated 429.0767 found 429.0767; [M + Na]+ for (C22H18Cl2N2NaO3) calculated 451.0587 found 451.0586.
4.1.3.4. 1-Allyl-3-(2-chloro-5-(trifluoromethyl)benzylidene)-6-(2-methoxybenzylidene)piperazine-2,5-dione (3g). According to the general procedure, compound 3g was synthesized as a yellow solid with a yield of 47.9%. m.p. 137–138 °C, FT-IR (neat, cm−1): 3180, 1682, 1620, 1489, 1386, 1352, 1321, 1246, 1132, 1122, 1080, 758, 748; 1H NMR (500 MHz, CDCl3) δ = 8.15 (s, 1H, N–H), 7.69 (s, 1H, Ar-H), 7.61 (d, J = 5.0 Hz, 1H, Ar-H), 7.53 (d, J = 5.0 Hz, 1H, Ar-H), 7.37 (t, J = 5.0 Hz, 1H, Ar-H), 7.25 (s, 1H,
C–H), 7.21 (d, J = 5.0 Hz, 1H, Ar-H), 7.08 (s, 1H, = C–H), 6.99 (t, J = 5.0 Hz, 1H, Ar-H), 6.93 (d, J = 10.0 Hz, 1H, Ar-H), 5.58–5.51 (m, 1H,
C–H), 5.01 (d, J = 10.0 Hz, 1H,
CH–H), 4.76 (d, J = 15.0 Hz, 1H,
CH–H), 4.26 (d, J = 5.0 Hz, 2H, –CH2–), 3.87 (s, 3H, –OCH3); 13C NMR (125 MHz, CDCl3) δ = 160.12, 158.26, 157.36, 138.14, 132.56, 131.21, 130.97, 130.77, 130.41, 130.02, 129.76, 128.67, 127.77, 126.47 (q, JC–F = 3.75 Hz, –CF3), 122.63, 120.27, 119.37, 118.38, 112.32, 110.68, 55.50, 47.43 ppm; HRMS (ESI) (m/z) [M + H]+ for (C23H19ClF3N2O3) calculated 463.1031 found 463.1037; [M + Na]+ for (C23H18ClF3N2NaO3) calculated 485.0850 found 485.0856.
4.1.3.5. 1-Allyl-6-(3-bromobenzylidene)-3-(3-methylbenzylidene)piperazine-2,5-dione (3j). According to the general procedure, compound 3j was synthesized as a yellow solid with a yield of 56.0%. m.p. 111–113 °C, FT-IR (neat, cm−1): 3197, 1680, 1614, 1456, 1353, 1332, 1238, 923, 777; 1H NMR (500 MHz, CDCl3) δ = 8.17 (s, 1H, N–H), 7.48 (d, J = 10.0 Hz, 1H, Ar-H), 7.44 (s, 1H, Ar-H), 7.33 (t, J = 10.0 Hz, 1H, Ar-H), 7.27 (t, J = 5.0 Hz, 1H, Ar-H), 7.23 (d, J = 5.0 Hz, 3H, Ar-H), 7.16 (d, J = 5.0 Hz, 1H, Ar-H, 1H,
C–H), 7.06 (s, 1H,
CH–H), 5.57–5.50 (m, 1H,
C–H), 5.04 (d, J = 10.0 Hz, 1H,
CH–H), 4.78 (d, J = 15.0 Hz, 1H,
CH–H), 4.26 (d, J = 5.0 Hz, 2H, –CH2–), 2.38 (s, 3H, –CH3); 13C NMR (125 MHz, CDCl3) δ = 159.50, 159.35, 139.20, 136.06, 132.68, 132.01, 131.69, 131.22, 129.89, 129.77, 129.27, 129.24, 129.08, 127.82, 125.58, 125.52, 122.52, 119.86, 118.44, 118.22, 48.05, 21.43 ppm; pm; HRMS (ESI) (m/z) [M + H]+ for (C22H20BrN2O2) calculated 423.0703 found 423.0709; [M + Na]+ for (C22H19BrN2NaO2) calculated 445.0522 found 445.0525.
4.1.3.6. 1-Allyl-6-(3-bromobenzylidene)-3-(2,3,4-trimethoxybenzylidene)piperazine-2,5-dione (3k). According to the general procedure, compound 3k was synthesized as a yellow solid with a yield of 51.7%. m.p. 146–148 °C, FT-IR (neat, cm−1): 3230, 1680, 1665, 1614, 1525, 1348, 1330, 1236, 1024, 921, 777, 680; 1H NMR (500 MHz, CDCl3) δ = 9.34 (s, 1H, N–H), 7.45 (d, J = 5.0 Hz, 1H, Ar-H), 7.42 (s, 1H, Ar-H), 7.25 (d, J = 5.0 Hz, 1H, Ar-H), 7.22 (d, J = 5.0 Hz, 1H, Ar-H), 7.16 (s, 1H,
C–H), 7.04 (d, J = 10.0 Hz, 1H, Ar-H), 6.95 (s, 1H,
C–H), 6.75 (d, J = 10.0 Hz, 1H, Ar-H), 5.59–5.52 (m, 1H,
C–H), 5.04 (d, J = 10.0 Hz, 1H,
CH–H), 4.79 (d, J = 15.0 Hz, 1H,
CH–H), 4.26 (d, J = 5.0 Hz, 2H, –CH2–), 3.92 (s, 3H, –OCH3), 3.90 (s, 6H, –OCH3); 13C NMR (125 MHz, CDCl3) δ = 160.01, 159.24, 154.74, 150.71, 142.79, 136.30, 131.98, 131.49, 131.43, 129.83, 129.71, 127.83, 126.72, 124.74, 122.44, 120.02, 119.29, 118.10, 115.44, 108.43, 61.93, 61.22, 56.10, 48.12 ppm; pm; HRMS (ESI) (m/z) [M + H]+ for (C24H24BrN2O5) calculated 499.0863 found 499.0870; [M + Na]+ for (C24H23BrN2NaO5) calculated 521.0683 found 521.0690.
4.1.3.7. 1-Allyl-3-(3-methylbenzylidene)-6-(3-nitrobenzylidene)piperazine-2,5-dione (3l). According to the general procedure, compound 3l was synthesized as a yellow solid with a yield of 62.0%. m.p. 145–146 °C, FT-IR (neat, cm−1): 3219, 1682, 1620, 1494, 1465, 1410, 1373, 1280, 1097, 906, 729; 1H NMR (500 MHz, CDCl3) δ = 8.21 (d, J = 10.0 Hz, 1H, Ar-H), 8.15 (s, 2H, Ar-H), 7.64–7.58 (m, 2H, Ar-H), 7.35 (t, J = 10.0 Hz, 1H, Ar-H), 7.23 (s, 1H, Ar-H; 1H,
C–H), 7.19 (d, J = 10.0 Hz, 1H, Ar-H), 7.10 (s, 1H,
C–H), 5.55–5.47 (m, 1H,
C–H), 5.03 (d, J = 10.0 Hz, 1H,
CH–H), 4.73 (d, J = 20.0 Hz, 1H,
CH–H), 4.25 (d, J = 5.0 Hz, 2H, –CH2–), 2.39 (s, 3H, –CH3); 13C NMR (125 MHz, CDCl3) δ = 159.17, 158.98, 148.07, 139.33, 135.89, 134.89, 132.57, 131.01, 130.33, 129.94, 129.41, 129.37, 129.08, 125.56, 125.25, 123.98, 123.28, 118.96, 118.31, 118.20, 48.12, 21.45 ppm; HRMS (ESI) (m/z) [M + H]+ for (C22H20N3O4) calculated 390.1448 found 390.1455; [M + Na]+ for (C22H19N3NaO4) calculated 412.1268 found 412.1278.
4.1.3.8. 1-Allyl-3-(3-chlorobenzylidene)-6-(3-nitrobenzylidene)piperazine-2,5-dione (3m). According to the general procedure, compound 3m was synthesized as a yellow solid with a yield of 48.6%. m.p. 172–173 °C, FT-IR (neat, cm−1): 3174, 1682, 1666, 1616, 1526, 1375, 1343, 1318, 731, 690; 1H NMR (500 MHz, CDCl3) δ = 8.40 (s, 1H, N–H), 8.22 (d, J = 10.0 Hz, 1H, Ar-H), 8.15 (s, 1H, Ar-H), 7.63–7.59 (m, 2H, Ar-H), 7.43 (s, 1H, Ar-H), 7.39 (t, J = 10.0 Hz, 1H, Ar-H), 7.32 (t, J = 10.0 Hz, 2H, Ar-H), 7.22 (s, 1H,
C–H), 7.05 (s, 1H,
C–H), 5.53–5.47 (m, 1H,
C–H), 5.04 (d, J = 10.0 Hz, 1H,
CH–H), 4.73 (d, J = 20.0 Hz, 1H,
CH–H), 4.23 (d, J = 5.0 Hz, 2H, –CH2–); 13C NMR (125 MHz, CDCl3) δ = 159.23, 158.85, 148.09, 135.74, 135.37, 134.88, 134.47, 130.87, 130.65, 130.07, 129.48, 129.01, 128.50, 126.74, 126.38, 123.98, 123.39, 118.73, 118.45, 117.03, 48.18 ppm; HRMS (ESI) (m/z) [M + H]+ for (C21H17ClN3O4) calculated 410.0902 found 410.0903; [M + Na]+ for (C21H16ClN3NaO4) calculated 432.0722 found 432.0722.
4.2. Anti-larval settlement assay
Adults of Balanus amphitrite were collected from Pak Sha Wan, Hong Kong (22°19′N, 114°16′E) while adults of Bugula neritina were collected from submerged rafts at fish farms in Yung Shue O, Hong Kong (22°24′N, 114°21′E). The settlement assays were carried out according to the method described by a previous study:20 Compounds dissolved in DMSO were diluted to a final concentration of 25.0 μg mL−1 with filtered seawater (FSW) for a preliminary anti-larval settlement activity test. The synthetic compounds were serial diluted with FSW to 25.0, 12.5, 6.25, 3.125, and 1.06 μg mL−1 for further bioassay. About 15–20 competent larvae were gently transferred into each well containing 1 mL of the testing solution, which was replaced by 0.1% DMSO in FSW in the negative controls. The plates for Balanus amphitrite were incubated for 48 h at 25 °C while those for Bugula neritina were incubated for 24 h at 25 °C. The effects of the compounds on larval settlement were determined by observing the plates under a dissecting microscope to check for (1) settled larvae, (2) swimming larvae, and (3) dead larvae. The number of settled larvae was expressed as a percentage of the total number of larvae per well.
EC50 was calculated as the concentration at which 50% of the larval population was inhibited from settling, compared with that in the negative control while LC50 was calculated as the concentration at which 50% of the larval population died. Each compound was tested using three different batches of larvae to determine the mean and standard deviation (SD) of the EC50 and LC50 values.
Conflict of interests
The authors declare no conflict of interests.
Acknowledgements
This work was supported by a grant from National Natural Science Foundation of China (21402218), the foundation of the innovation team of Zhongshan City, the National Key Basic Research Program of China (973 Project, 2011CB915503), the National High Technology Research and Development Program (863 Program, 2012AA092104), the Strategic Priority Research Program of the Chinese Academy of Sciences (XDA11030403), Guangdong Marine Economic Development and Innovation of Regional Demonstration Project (GD2012-D01-001).
References and notes
- G. D. Bixler and B. Bhushan, Philos. Trans. R. Soc., A, 2012, 370, 2381–2417 CrossRef CAS PubMed.
- N. Fusetani, Nat. Prod. Rep., 2011, 28, 400–410 RSC.
- Y. T. Cui, S. L. Teo, W. Leong and C. L. Chai, Int. J. Mol. Sci., 2014, 15, 9255–9284 CrossRef CAS PubMed.
- P. Y. Qian, Y. Xu and N. Fusetani, Biofouling, 2010, 26, 223–234 CrossRef CAS PubMed.
- C. M. Kirschner and A. B. Brennan, Annu. Rev. Mater. Res., 2012, 42, 211–229 CrossRef CAS PubMed.
- P. Y. Qian, Z. Li, Y. Xu, Y. Li and N. Fusetani, Biofouling, 2015, 31, 101–122 CrossRef CAS PubMed.
- V. Piazza, I. Dragic, K. Sepcic, M. Faimali, F. Garaventa, T. Turk and S. Berne, Mar. Drugs, 2014, 12, 1959–1976 CrossRef PubMed.
- D. Blihoghe, E. Manzo, A. Villela, A. Cutignano, G. Picariello, M. Faimali and A. Fontana, Biofouling, 2011, 27, 99–109 CrossRef CAS PubMed.
- S. Kantheti, R. Narayan and K. V. S. N. Raju, RSC Adv., 2015, 5, 3687–3708 RSC.
- M. S. Majik, S. Tilvi, S. Mascarenhas, V. Kumar, A. Chatterjee and M. Banerjee, RSC Adv., 2014, 4, 28259–28264 RSC.
- Y. Wang, P. Wang, H. Ma and W. Zhu, Expert Opin. Ther. Pat., 2013, 23, 1415–1433 CrossRef CAS PubMed.
- A. D. Borthwick, Chem. Rev., 2012, 112, 3641–3716 CrossRef CAS PubMed.
- S.-H. Qi, Y. Xu, H.-R. Xiong, P.-Y. Qian and S. Zhang, World J. Microbiol. Biotechnol., 2009, 25, 399–406 CrossRef CAS.
- X. Li, S. Dobretsov, Y. Xu, X. Xiao, O. S. Hung and P. Y. Qian, Biofouling, 2006, 22, 201–208 CrossRef CAS PubMed.
- M. Sjogren, A. L. Johnson, E. Hedner, M. Dahlstrom, U. Goransson, H. Shirani, J. Bergman, P. R. Jonsson and L. Bohlin, Peptides, 2006, 27, 2058–2064 CrossRef PubMed.
- S. R. Liao, X. C. Qin, D. Li, Z. C. Tu, J. S. Li, X. F. Zhou, J. F. Wang, B. Yang, X. P. Lin, J. Liu, X. W. Yang and Y. H. Liu, Eur. J. Med. Chem., 2014, 83, 236–244 CrossRef CAS PubMed.
- S. Ando, A. L. Grote and K. Koide, J. Org. Chem., 2011, 76, 1155–1158 CrossRef CAS PubMed.
- W. A. Loughlin, R. L. Marshall, A. Carreiro and K. E. Elson, Bioorg. Med. Chem. Lett., 2000, 10, 91–94 CrossRef CAS.
- X. J. Zhou, Z. Zhang, Y. Xu, C. L. Jin, H. P. He, X. J. Hao and P. Y. Qian, Biofouling, 2009, 25, 69–76 CrossRef CAS PubMed.
- Y. X. Li, F. Y. Zhang, Y. Xu, K. Matsumura, Z. Han, L. L. Liu, W. H. Lin, Y. X. Jia and P. Y. Qian, Biofouling, 2012, 28, 857–864 CrossRef CAS PubMed.
- B. G. Wang, J. B. Gloer, N. Y. Ji and J. C. Zhao, Chem. Rev., 2013, 113, 3632–3685 CrossRef CAS PubMed.
- M. T. Cabrita, C. Vale and A. P. Rauter, Mar. Drugs, 2010, 8, 2301–2317 CrossRef CAS PubMed.
- B. M. Xi, Z. Z. Jiang, J. W. Zou, P. Z. Ni and W. H. Chen, Bioorg. Med. Chem., 2011, 19, 783–788 CrossRef CAS PubMed.
- Y. Xu, H. P. He, S. Schulz, X. Liu, N. Fusetani, H. R. Xiong, X. Xiao and P. Y. Qian, Bioresour. Technol., 2010, 101, 1331–1336 CrossRef CAS PubMed.
Footnote |
† Electronic supplementary information (ESI) available. See DOI: 10.1039/c5ra06210a |
|
This journal is © The Royal Society of Chemistry 2015 |
Click here to see how this site uses Cookies. View our privacy policy here.