DOI:
10.1039/C5RA04795A
(Paper)
RSC Adv., 2015,
5, 30826-30832
Facile “on water” domino reactions for the expedient synthesis of 2H-thiopyrano[2,3-b]quinolines†
Received
8th December 2014
, Accepted 23rd March 2015
First published on 23rd March 2015
Abstract
A facile synthesis of novel 3-nitro-2-aryl-2H-thiopyrano[2,3-b]quinolines from the domino reactions of 2-mercaptoquinoline-3-carbaldehyde and substituted β-nitrostyrenes in the presence of triethylamine (TEA) in water is described. This “on water” protocol proceeds with high atom economy through the creation of one C–S and one C–C bond presumably via a Michael addition-intramolecular aldol-dehydration domino sequence.
Introduction
Domino reactions involving two or more reactants lead to the expedient assembly of molecules of high structural complexity in a convergent and synthetically efficient manner. These processes occurring in one pot avoid the isolation and purification of intermediates, maximize the yield of the final product, minimize the waste of solvent and chromatographic stationary phases and enhance the greenness of the transformations.1 Consequently, domino reactions have become an attractive tool in combinatorial synthesis2 and drug development programs.3
Another important facet of synthetic organic chemistry pertains to the efficient access of natural products, pharmaceuticals, agrochemicals etc. in green solvents viz. water, ionic liquids etc. In particular, water as solvent for reactions offers unique advantages such as abundant availability, environment-friendliness and safety.4 Further, the lipophilicity of water renders the facile segregation of the product. In addition, water facilitates many organic reactions, in particular, when the reactants are initially emulsionated in water and the transition states better solvated by hydrogen bonds with unsymmetrically H-bonded water molecules than the reactants. These reactions known as “on water” processes result in enhancements of rate and product yield compared to that in organic solvents which are usually toxic or unsafe. Moreover, water as a reaction medium facilitates unique solvation modes and assembly processes furnishing selectivities and reactivities that are often difficult to realize in organic solvents.5
Numerous natural and synthetic analogues comprising quinoline ring system display interesting biological activities such as antiviral,6 antitumor,7 antibacterial,8 anti-inflammatory,9 antiasthmatic,10 antiproliferative,11 antimalarial,12 anti allergenic,13 antifungal,14 MCH1R antagonist15 and potent HIV-integrase inhibition.16 Among them, thiopyrano[2,3-b]quinoline exhibits remarkable biological activities such as antioxidant17 and mGlu 1 receptor activity.18 (Fig. 1).
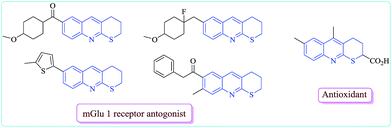 |
| Fig. 1 Selected examples of biologically active functionalized thiopyrano [2,3-b]quinolines. | |
Synthetic methods for the construction thiapyrano[2,3-b]quinolines include the reactions of (i) 2-mercapto-4-methylquinoline and maleic anhydride in the presence of nanostructured-TiO2,17 (ii) 2-(3-methylbut-2-enylthio)quinoline-3-carbaldehyde and anilines in the presence of silica gel supported InCl3 in CH3CN,19 (iii) 2-arylthioquinolin-3-carbaldehydes and activated olefins through intramolecular Friedel–Crafts reaction of Morita–Baylis–Hillman adducts in the presence of acid in dichloromethane,20 (iv) 2-methylthio-3-(2-bromobenzoyl)quinoline in the presence of AIBN via radical cyclization in toluene,21 (v) 7-substituted 2-allylsulfanyl-3-hydroximinomethylquinolines in the presence of aqueous NaOCl in CHCl3,22 (vi) 3-formyl-2-mercaptoquinoline with ethyl acetoacetate/diethyl malonate/ethyl cyanoacetate in the presence of piperidine in CH3CN,23 (vii) 3-formyl-2-mercaptoquinoline with chloroacetyl chloride in DMF,24 (viii) 3-formyl-2-mercaptoquinoline and phenoxyacetic acid in the presence of triethylamine and acetic anhydride,25 (ix) 3-formyl-2-mercaptoquinoline with acetyl chloride in the presence of sodium methoxide,26 (x) 3-formyl-2-mercaptoquinoline, malononitrile and 4-methylquinoline-2-thiol in the presence of anhydrous ammonium hydroxide,27 (xi) 2-mercaptoquinoline-3-carbaldehyde and citral in the presence of EDDA in xylene,28 (xii) α,β-unsaturated aldehyde and 2-mercaptoquinoline-3-carbaldehyde in the presence of chiral organocatalysts in CHCl3 (ref. 29) and (xiii) acrylonitrile and 2-mercaptoquinoline-3-carbaldehyde in the presence of triethylamine in DMF.30 These approaches, however, are associated with one or more drawbacks such as corrosive reagents, moderate yield, toxic solvents, expensive catalysts, lack of convergence and tedious work up.
These considerations in conjunction with the biological relevance of thiapyrano[2,3-b]quinoline ring system prompted us to develop an atom-efficient synthesis as a part of our ongoing research programme on the construction biologically significant novel heterocycles through domino31 and/or green transformations32 and report the results in this manuscript. In the present study, we have evolved an environmentally benign one-pot two-component domino synthesis of novel 3-nitro-2-aryl-2H-thiopyrano[2,3-b]quinolines from the reaction of 2-mercaptoquinoline-3-carbaldehyde 1 and substituted β-nitrostyrenes 2 in the presence of triethylamine (TEA) (25 mol%) in water (Scheme 1).
 |
| Scheme 1 Synthesis of 2H-thiopyrano[2,3-b]quinolines 3. | |
Results and discussion
We began our study with the optimization of the model two-component reaction between equimolecular amounts of 2-mercaptoquinoline-3-carbaldehyde and β-nitrostyrene employing different solvents, bases and temperature and the results are summarized in Table 1. It was found that when water was used as the reaction medium in the presence of Na2CO3, the yield of the product was very low (Table 1, Entry 16). On the other hand, this reaction in water in the presence of TEA furnished a higher yield of the product in shorter reaction time than in other solvents (Table 1, Entries 9–14). Consequently, we have performed all subsequent reactions in water. A study of the effect of temperature on the reaction outcome shows that the yield of the product 3e increases from 63 to 93% with an increase in temperature from 40 to 100 °C (Table 1, Entries 1–4) respectively. The optimal catalyst load investigated with 25, 50, 75 and 100 mol% of TEA on the model reaction discloses that 25 mol% of TEA is sufficient to obtain an excellent yield of 94% of 3e (Table 1, Entry 7). Hence all reactions were performed in water at 100 °C with 25 mol% of triethylamine. That this reaction proceeds via “on water” protocol is evident from the fact that (i) initially the mercaptoquinolinecarbaldehyde and β-nitrostyrenes are not soluble in water, (ii) upon addition of TEA, the former partially goes into solution during the progress of the reaction and (iii) the yield of the product is maximum in water as solvent. One great advantage associated with this protocol is that the isolation and purification of the product could be achieved by simply filtering the product and washing with cold ethanol. This protocol has an added advantage as column chromatographic purification has been avoided in workup.
Table 1 Optimization of the reaction conditions for the synthesis of 3e
Entry |
Base (100 mol%) |
Solvent |
Time (h) |
Temp (°C) |
Yield of 3ee (%) |
75 mol% used. 50 mol% used. 25 mol% used. These reactions performed at reflux conditions. Isolated yield after washing with cold ethanol. |
1 |
TEA |
Water |
8.0 |
rt |
63 |
2 |
TEA |
Water |
4.0 |
40 |
72 |
3 |
TEA |
Water |
3.5 |
70 |
89 |
4 |
TEA |
Water |
2.0 |
—d |
93 |
5 |
TEAa |
Water |
2.1 |
—d |
92 |
6 |
TEAb |
Water |
2.4 |
—d |
93 |
7 |
TEAc |
Water |
3.0 |
—d |
94 |
9 |
TEA |
EtOH |
4.0 |
—d |
84 |
10 |
TEA |
MeOH |
3.0 |
—d |
75 |
11 |
TEA |
DCM |
4.3 |
—d |
70 |
12 |
TEA |
THF |
3.5 |
—d |
62 |
13 |
TEA |
CH3CN |
4.5 |
—d |
59 |
14 |
TEA |
DMF |
3.0 |
—d |
57 |
15 |
K2CO3 |
Water |
5.0 |
—d |
64 |
16 |
Na2CO3 |
Water |
6.5 |
—d |
34 |
17 |
DABCO |
Water |
6.0 |
—d |
49 |
18 |
DBU |
Water |
4.0 |
—d |
55 |
19 |
DMAP |
Water |
4.5 |
—d |
61 |
20 |
Pyridine |
Water |
4.0 |
—d |
58 |
Typically, the reaction was performed with a mixture of 2-mercaptoquinoline-3-carbaldehyde 1 (1.0 mmol) and substituted β-nitrostyrene 2 (1.0 mmol) in the presence of TEA (0.25 mol) in water (10 ml) under heating to reflux at 100 °C for 3–4 h. After completion of the reaction (TLC), the resulting crude product was purified by washing with cold ethanol, which afforded a library of 3-nitro-2-aryl-2H-thiopyrano[2,3-b]quinolines 3 in 85–96% yields (Table 2). This domino reaction proceeded well with electron-releasing as well as electron-withdrawing group, sterically hindered aryl rings and heteroaromatic nitro olefins as well. Moreover, the reaction proceeds well with quinoline part bearing electron releasing groups such as –Me and –OMe, while the presence of substituents like –Cl and –Br led to a mixture of products.
Table 2 Synthesis of 2H-thiopyrano[2,3-b]quinolines 3
Entry |
Comp. |
R |
Ar |
Time (h) |
Yield of 3a (%) |
Isolated yield after washing with cold ethanol. |
1 |
3a |
H |
4-FC6H4 |
4.0 |
87 |
2 |
3b |
H |
4-ClC6H4 |
3.3 |
89 |
3 |
3c |
H |
4-BrC6H4 |
3.5 |
92 |
4 |
3d |
H |
4-CF3C6H4 |
4.0 |
85 |
5 |
3e |
H |
C6H5 |
3.0 |
94 |
6 |
3f |
H |
4-MeC6H4 |
3.0 |
95 |
7 |
3g |
H |
4-EtC6H4 |
3.0 |
93 |
8 |
3h |
H |
4-ButC6H4 |
3.0 |
92 |
9 |
3i |
H |
4-MeOC6H4 |
3.0 |
96 |
10 |
3j |
H |
2-FC6H4 |
4.0 |
90 |
11 |
3k |
H |
2-MeOC6H4 |
3.0 |
94 |
12 |
3l |
H |
3-FC6H4 |
3.0 |
92 |
13 |
3m |
H |
3-MeOC6H4 |
3.0 |
95 |
14 |
3n |
H |
1-Naphthyl |
3.0 |
91 |
15 |
3o |
H |
2-Thienyl |
3.0 |
94 |
16 |
3p |
Me |
4-ClC6H4 |
3.0 |
90 |
17 |
3q |
Me |
4-MeC6H4 |
3.0 |
93 |
18 |
3r |
OMe |
4-MeC6H4 |
3.0 |
91 |
Finally, the TEA in the aqueous filtrate after the reaction was over could also be removed by our previously reported procedure by passing the solution through polymer-bound acid resin, Indion 790 to ensure the purity of water ultimately let into the environment thereby obviating pollution.32c Further, this transformation is characterized by remarkable synthetic efficiency affording the product with innocuous by-product, water.
The structure of 3-nitro-2-aryl-2H-thiopyrano[2,3-b]quinolines 3 was deduced from one- and two-dimensional NMR spectroscopic data as detailed for 3f as a representative example (Video ESI†). Finally, the structure of the product was also confirmed by an X-ray crystallographic study34 of a single crystal of 3c (Fig. 2).
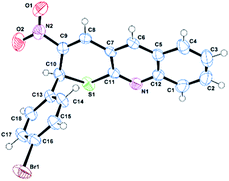 |
| Fig. 2 ORTEP diagram for 3c. | |
A plausible mechanism for the formation of 3-nitro-2-aryl-2H-thiopyrano[2,3-b]quinolines is depicted in Scheme 2.
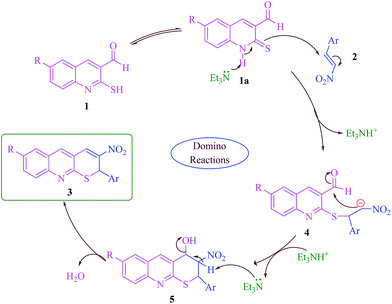 |
| Scheme 2 Probable domino mechanistic pathway leading to the formation of 3. | |
Presumably, this transformation is presumably triggered by the Michael addition of the thiolate anion of 2-mercaptoquinoline-3-carbaldehyde, generated from the reaction of 2-mercaptoquinoline-3-carbaldehyde 1 with base, to β-nitrostyrenes 2 affording intermediate 4. Subsequent intramolecular aldol reaction of 4 and concomitant elimination of water molecule furnishes 3-nitro-2-aryl-2H-thiopyrano[2,3-b]quinolines 3. Overall, this is a two-component domino transformation via the formation of one C–S and one C–C bond.
Conclusion
In conclusion, we have developed an eco-friendly two-component “on water” domino protocol for the facile synthesis of novel 3-nitro-2-aryl-2H-thiopyrano[2,3-b]quinolines in good yields from simple, readily available starting materials. This protocol, following group-assisted-purification chemistry (GAP Chemistry)33 obviating traditional column chromatographic and recrystallization steps, occurs with the formation of a C–S and a C–C bond in a one pot operation.
Experimental section
General information
Melting points were measured in open capillary tubes and are uncorrected. The 1H-NMR, 13C-NMR, DEPT, H,H-COSY, C,H-COSY and HMBC spectra were recorded on a Bruker (Avance) 300 MHz NMR instrument using TMS as internal standard and CDCl3 as solvent. Standard Bruker software was used throughout. Chemical shifts are given in parts per million (δ-scale) and the coupling constants are given in Hertz. Silica gel-G plates (Merck) were used for TLC analysis with a mixture of petroleum ether (60–80 °C) and ethyl acetate as eluent. Mass spectra were recorded in LCQ Fleet mass spectrometer, Thermo Fisher Instruments Limited, US. Electrospray ionisation mass spectrometry (ESI-MS) analysis was performed in the positive ion mode on a liquid chromatography ion trap.
General procedure for the synthesis of 3-nitro-2-aryl-2H-thiopyrano[2,3-b]quinolines (3a–r)
A mixture of 2-mercaptoquinoline-3-carbaldehyde (1.0 mmol) and substituted β-nitrostyrenes (1.0 mmol) in the presence of triethylamine (TEA) (0.25 mmol) in water (10 ml) was heated to reflux at 100 °C for 3–4 h. After completion of the reaction (TLC), the resulting crude product was purified by washing with cold ethanol, which afforded a library of 3-nitro-2-aryl-2H-thiopyrano[2,3-b]quinolines 3 in excellent yields.
2-(4-Fluorophenyl)-3-nitro-2H-thiopyrano[2,3-b]quinoline 3a. Yellow solid. Yield: 87%; mp. 167–168 °C; 1H NMR (300 MHz, CDCl3) δH: 5.77 (s, 1H), 6.89–6.96 (m, 2H), 7.20–7.26 (m, 2H), 7.52–7.57 (m, 1H), 7.77 (td, J = 8.4, 1.2 Hz, 1H), 7.84 (d, J = 8.1 Hz, 1H), 7.95 (d, J = 8.4 Hz, 1H), 8.20 (s, 1H), 8.31 (s, 1H); 13C NMR (75 MHz, CDCl3) δC: 41.5, 116.0 (2JC,F = 21.7 Hz), 121.9, 126.1, 127.0, 128.1 (3JC,F = 8.4 Hz), 128.4, 128.5, 130.9, 132.5, 136.0 (4JC,F = 3.3 Hz), 139.4, 145.2, 149.4, 155.1, 162.6 (1JC,F = 246.6 Hz); ESI-MS: m/z. Calcd: 338.05; found: 339.14 (M+).
2-(4-Chlorophenyl)-3-nitro-2H-thiopyrano[2,3-b]quinoline 3b. Pale yellow solid. Yield: 89%; mp. 163–164 °C; 1H NMR (300 MHz, CDCl3) δH: 5.75 (s, 1H), 7.17–7.23 (m, 4H), 7.53–7.58 (m, 1H), 7.77 (td, J = 8.4, 1.2 Hz, 1H), 7.84 (d, J = 8.1 Hz, 1H), 7.94 (d, J = 8.7 Hz, 1H), 8.21 (s, 1H), 8.32 (s, 1H); 13C NMR (75 MHz, CDCl3) δC: 41.6, 121.9, 126.1, 127.1, 127.3, 127.7, 128.5, 129.3, 131.1, 132.6, 134.5, 138.6, 139.5, 145.0, 149.4, 155.0; ESI-MS: m/z. Calcd: 354.02; found: 355.19 (M+).
2-(4-Bromophenyl)-3-nitro-2H-thiopyrano[2,3-b]quinoline 3c. Pale yellow solid. Yield: 92%; mp. 198–199 °C; 1H NMR (300 MHz, CDCl3) δH: 5.74 (s, 1H), 7.13 (d, J = 8.4 Hz, 2H), 7.35–7.38 (m, 2H), 7.53–7.58 (m, 1H), 7.76 (td, J = 7.8, 1.3 Hz, 1H), 7.85 (d, J = 8.4 Hz, 1H), 7.95 (d, J = 8.4 Hz, 1H), 8.21 (s, 1H), 8.32 (s, 1H); 13C NMR (75 MHz, CDCl3) δC: 41.7, 121.9, 122.7, 126.1, 127.1, 128.0, 128.5, 128.5, 131.1, 132.3, 132.6, 139.1, 139.5, 144.9, 149.4, 154.9; ESI-MS: m/z. Calcd: 397.97; found: 399.06 (M+), 401.12 (M + 3).
3-Nitro-2-(4-(trifluoromethyl)phenyl)-2H-thiopyrano[2,3-b]quinoline 3d. Yellow solid. Yield: 85%; mp. 160–161 °C; 1H NMR (300 MHz, CDCl3) δH: 5.82 (s, 1H), 7.38 (d, J = 8.1 Hz, 2H), 7.50–7.57 (m, 3H), 7.77 (t, J = 7.6 Hz, 1H), 7.84 (d, J = 7.8 Hz, 1H), 7.94 (d, J = 8.4 Hz, 1H), 8.29 (s, 1H), 8.35 (s, 1H) 13C NMR (75 MHz, CDCl3) δC: 41.8, 121.8, 126.1, 126.2, 126.7, 127.2, 128.5, 128.6, 130.7 (2JC,F = 32.9 Hz), 131.5, 132.7, 139.7, 143.8, 144.5, 149.4, 154.7; ESI-MS: m/z. Calcd: 388.05; found: 389.20 (M+).
3-Nitro-2-phenyl-2H-thiopyrano[2,3-b]quinoline 3e. Pale yellow solid. Yield: 94%; mp. 206–207 °C; 1H NMR (300 MHz, CDCl3) δH: 5.78 (s, 1H), 7.21–7.24 (m, 5H), 7.50–7.56 (m, 1H), 7.75 (td, J = 8.4, 1.2 Hz, 1H), 7.83 (d, J = 8.1 Hz, 1H), 7.93 (d, J = 8.4 Hz, 1H), 8.19 (s, 1H), 8.31 (s, 1H); 13C NMR (75 MHz, CDCl3) δC: 42.2, 122.2, 126.4, 126.3, 126.9, 128.4, 128.5, 128.6, 129.1, 130.9, 132.4, 139.3, 140.0, 145.3, 149.3, 155.5; ESI-MS: m/z. Calcd: 320.06; found: 321.21 (M+).
3-Nitro-2-(p-tolyl)-2H-thiopyrano[2,3-b]quinoline 3f. Yellow solid. Yield: 95%; mp. 214–215 °C; 1H NMR (300 MHz, CDCl3) δH: 2.26 (s, 3H), 5.76 (s, 1H), 7.03 (d, J = 8.4 Hz, 2H), 7.13 (d, J = 8.1 Hz, 2H), 7.53 (t, J = 7.5 Hz, 1H), 7.75 (td, J = 7.8, 1.5 Hz, 1H), 7.83 (d, J = 8.4 Hz, 1H), 7.93 (d, J = 8.7 Hz, 1H), 8.18 (s, 1H), 8.30 (s, 1H); 13C NMR (75 MHz, CDCl3) δC: 21.0, 42.1, 122.2, 126.2, 126.3, 126.8, 128.4, 128.5, 129.8, 130.5, 132.2, 137.2, 138.5, 139.0, 145.7, 149.4, 155.7; ESI-MS: m/z. Calcd: 334.08; found: 335.18 (M+).
2-(4-Ethylphenyl)-3-nitro-2H-thiopyrano[2,3-b]quinoline 3g. Pale yellow solid. Yield: 93%; mp. 217–218 °C; 1H NMR (300 MHz, CDCl3) δH: 1.14 (t, J = 7.5 Hz, 3H), 2.55 (q, J = 7.6 Hz, 2H), 5.76 (s, 1H), 7.05 (d, J = 8.1 Hz, 2H), 7.15 (d, J = 8.4 Hz, 2H), 7.50–7.55 (m, 1H), 7.75 (td, J = 7.7, 1.4 Hz, 1H), 7.83 (d, J = 7.8 Hz, 1H), 7.93 (d, J = 8.4 Hz, 1H), 8.18 (s, 1H), 8.30 (s, 1H); 13C NMR (75 MHz, CDCl3) δC: 15.3, 28.4, 42.0, 122.2, 126.1, 126.2, 126.9, 128.4, 128.5, 128.6, 130.6, 132.3, 137.3, 139.2, 144.8, 145.5, 149.3, 155.6; ESI-MS: m/z. Calcd: 348.09; found: 349.22 (M+).
2-(4-(tert-Butyl)phenyl)-3-nitro-2H-thiopyrano[2,3-b]quinoline 3h. Pale yellow solid. Yield: 92%; mp. 252–253 °C; 1H NMR (300 MHz, CDCl3) δH: 1.22 (s, 9H), 5.77 (s, 1H), 7.16 (d, J = 8.4 Hz, 2H), 7.24 (d, J = 8.4 Hz, 2H), 7.53 (t, J = 7.5 Hz, 1H), 7.73–7.78 (m, 1H), 7.84 (d, J = 8.1 Hz, 1H), 7.94 (d, J = 8.4 Hz, 1H), 8.19 (s, 1H), 8.31 (s, 1H); 13C NMR (75 MHz, CDCl3) δC: 31.1, 34.5, 41.9, 122.3, 125.9, 126.0, 126.1, 126.9, 128.4, 128.5, 130.6, 132.3, 136.9, 139.1, 145.6, 149.3, 151.6, 155.6; ESI-MS: m/z. Calcd: 376.12; found: 377.21 (M+).
2-(4-Methoxyphenyl)-3-nitro-2H-thiopyrano[2,3-b]quinoline 3i. Yellow solid. Yield: 96%; mp. 208–209 °C; 1H NMR (300 MHz, CDCl3) δH: 3.72 (s, 3H), 5.75 (s, 1H), 6.73–6.76 (m, 2H), 7.15–7.18 (m, 2H), 7.51–7.56 (m, 1H), 7.76 (td, J = 7.7, 1.4 Hz, 1H), 7.83 (d, J = 8.1 Hz, 1H), 7.94 (d, J = 8.4 Hz, 1H), 8.19 (s, 1H), 8.28 (s, 1H); 13C NMR (75 MHz, CDCl3) δC: 41.8, 55.2, 114.4, 122.2, 126.1, 126.9, 127.6, 128.4, 128.5, 130.4, 132.2, 132.3, 145.6, 149.3, 155.6, 159.7; ESI-MS: m/z. Calcd: 350.07; found: 351.21 (M+).
2-(2-Fluorophenyl)-3-nitro-2H-thiopyrano[2,3-b]quinoline 3j. Pale yellow solid. Yield: 90%; mp. 216–217 °C; 1H NMR (300 MHz, CDCl3) δH: 6.10 (s, 1H), 6.94 (t, J = 7.5 Hz, 1H), 7.01–7.11 (m, 2H), 7.19–7.24 (m, 1H) 7.54 (t, J = 7.5 Hz, 1H), 7.73–7.78 (m, 1H), 7.84 (d, J = 7.8 Hz, 1H), 7.93 (d, J = 8.4 Hz, 1H), 8.21 (s, 1H), 8.40 (s, 1H); 13C NMR (75 MHz, CDCl3) δC: 35.9 (3JC,F = 3.7 Hz), 116.3 (2JC,F = 21.2 Hz), 122.0, 124.5 (3JC,F = 3.5 Hz), 126.2, 127.0, 127.1 (4JC,F = 2.7 Hz), 127.3, 128.5, 130.3 (3JC,F = 8.3 Hz), 132.0, 132.4, 139.4, 143.8, 149.4, 155.4, 159.2 (1JC,F = 248.2 Hz); ESI-MS: m/z. Calcd: 338.05; found: 339.18 (M+).
2-(2-Methoxyphenyl)-3-nitro-2H-thiopyrano[2,3-b]quinoline 3k. Yellow solid. Yield: 94%; mp. 199–200 °C; 1H NMR (300 MHz, CDCl3) δH:3.86, (s, 3H), 6.19 (s, 1H), 6.72 (t, J = 7.5 Hz, 1H), 6.89 (d, J = 8.4 Hz, 1H), 6.94 (dd, J = 7.5, 1.5 Hz, 1H), 7.17–7.23 (m, 1H), 7.51 (t, J = 7.5 Hz, 1H), 7.72 (td, J = 7.7, 1.3 Hz, 1H), 7.82 (d, J = 8.1 Hz, 1H), 7.89 (d, J = 8.4 Hz, 1H), 8.18 (s, 1H), 8.40 (s, 1H); 13C NMR (75 MHz, CDCl3) δC: 36.4, 55.6, 111.3, 120.5, 122.5, 126.0, 126.1, 126.7, 127.4, 128.3, 128.4, 129.7, 132.0, 132.1, 139.0, 144.4, 149.2, 155.7, 156.7; ESI-MS: m/z. Calcd: 350.07; found: 351.18 (M+).
2-(3-Fluorophenyl)-3-nitro-2H-thiopyrano[2,3-b]quinoline 3l. Yellow solid. Yield: 92%; mp. 210–211 °C; 1H NMR (300 MHz, CDCl3) δH: 5.77 (s, 1H), 6.94 (d, J = 9.3 Hz, 2H), 7.05 (d, J = 7.8 Hz, 1H), 7.19–7.23 (m, 1H), 7.55 (t, J = 7.5 Hz, 1H), 7.75 (m, 1H), 7.85 (d, J = 8.1 Hz, 1H), 7.95 (d, J = 8.1 Hz, 1H), 8.22 (s, 1H), 8.34 (s, 1H); 13C NMR (75 MHz, CDCl3) δC: 39.3, 111.5 (2JC,F = 22.3 Hz), 113.4 (2JC,F = 20.8 Hz), 120.3 (4JC,F = 2.8 Hz), 120.4, 124.4, 125.1, 126.1, 127.3, 129.2 (3JC,F = 8.1 Hz), 130.6, 130.7, 138.9, 141.6 (3JC,F = 6.7 Hz), 142.4, 147.1, 153.0, 160.7 (1JC,F = 241.0 Hz); ESI-MS: m/z. Calcd: 338.05; found: 339.2 (M+).
2-(3-Methoxyphenyl)-3-nitro-2H-thiopyrano[2,3-b]quinoline 3m. Pale yellow solid. Yield: 95%; mp. 152–153 °C; 1H NMR (300 MHz, CDCl3) δH: 3.71 (s, 3H), 5.75 (s, 1H), 6.74–6.83 (m, 3H), 7.15 (t, J = 7.6 Hz, 1H), 7.53 (t, J = 7.5 Hz, 1H), 7.72–7.78 (m, 1H), 7.82 (d, J = 8.1 Hz, 1H), 7.93 (d, J = 8.7 Hz, 1H), 8.18 (s, 1H), 8.32 (s, 1H); 13C NMR (75 MHz, CDCl3) δC: 42.1, 55.2, 112.5, 113.5, 118.5, 122.1, 126.1, 126.9, 128.4, 128.5, 130.2, 131.0, 132.3, 139.3, 141.4, 145.1, 149.3, 155.4, 159.9; ESI-MS: m/z. Calcd: 350.07; found: 351.20 (M+).
2-(Naphthalen-1-yl)-3-nitro-2H-thiopyrano[2,3-b]quinoline 3n. Yellow solid. Yield: 91%; mp. 287–288 °C; 1H NMR (300 MHz, CDCl3) δH: 6.62 (s, 1H), 7.14–7.22 (m, 2H), 7.49–7.59 (m, 2H), 7.66–7.75 (m, 3H), 7.86 (t, J = 7.9 Hz, 3H), 8.20 (d, J = 8.7 Hz, 1H), 8.26 (s, 1H), 8.55 (s, 1H); 13C NMR (75 MHz, CDCl3) δC: 37.9, 122.2, 122.7, 123.2, 125.0, 126.1, 126.2, 127.0, 127.1, 128.5, 129.1, 129.2, 129.4, 132.3, 132.4, 134.2, 134.5, 139.5, 144.7, 149.3, 155.5; ESI-MS: m/z. Calcd: 370.08; found: 371.19 (M+).
3-Nitro-2-(thiophen-2-yl)-2H-thiopyrano[2,3-b]quinoline 3o. Green solid. Yield: 94%; mp. 201–202 °C; 1H NMR (300 MHz, CDCl3) δH: 6.06 (s, 1H), 6.82 (dd, J = 5.1, 3.6 Hz, 1H), 6.95 (dt, J = 3.6, 1.0 Hz, 1H), 7.11 (dd, J = 5.1, 1.2 Hz, 1H), 7.55 (ddd, J = 8.1, 6.9, 1.2 Hz, 1H), 7.78 (ddd, J = 8.4, 6.9, 1.5 Hz, 1H), 7.84 (d, J = 8.1 Hz, 1H), 7.98 (d, J = 8.1 Hz, 1H), 8.22 (s, 1H), 8.25 (s, 1H); 13C NMR (75 MHz, CDCl3) δC: 37.6, 122.1, 125.5, 125.9, 126.2, 127.0, 127.1, 128.5, 130.4, 132.5, 139.5, 143.0, 145.5, 149.4, 155.0, 166.1; ESI-MS: m/z. Calcd: 326.02; found: 327.14 (M+).
2-(4-Chlorophenyl)-7-methyl-3-nitro-2H-thiopyrano[2,3-b]quinoline 3p. Yellow solid. Yield: 90% mp. 251–252; 1H NMR (300 MHz, CDCl3) δH: 2.54 (s, 3H), 5.73 (s, 1H), 7.16–7.22 (m, 4H), 7.59–7.61 (m, 2H), 7.84 (d, J = 9.3 Hz, 1H), 8.11 (s, 1H), 8.31 (s, 1H); 13C NMR (75 MHz, CDCl3) δC: 21.4, 41.5, 121.8, 126.2, 127.3, 127.7, 128.1, 129.2, 131.3, 134.4, 134.8, 137.2, 138.6, 138.9, 144.8, 148.0, 153.7; ESI-MS: m/z. Calcd: 368.04; found: 369.06 (M+).
7-Methyl-3-nitro-2-(p-tolyl)-2H-thiopyrano[2,3-b]quinoline 3q. Light yellow solid. Yield: 93% mp. 238–239; 1H NMR (300 MHz, CDCl3) δH: 2.25 (s, 3H), 2.53 (s, 3H), 5.74 (s, 1H), 7.02 (d, J = 8.1 Hz, 2H), 7.12 (d, J = 7.8 Hz, 2H), 7.56–7.58 (m, 2H), 7.83 (d, J = 9.0 Hz, 1H), 8.09 (s, 1H), 8.28 (s, 1H); 13C NMR (75 MHz, CDCl3) δC: 21.0, 21.4, 41.9, 122.1, 126.2, 127.3, 128.1, 129.7, 130.9, 134.6, 137.0, 137.1, 138.4, 138.6, 145.3, 148.0, 154.3; ESI-MS: m/z. Calcd: 348.09; found: 349.12 (M+).
7-Methoxy-3-nitro-2-(p-tolyl)-2H-thiopyrano[2,3-b]quinoline 3r. Orange solid. Yield: 91% mp. 197–198; 1H NMR (300 MHz, CDCl3) δH: 2.25 (s, 3H), 3.94 (s, 3H), 5.72 (s, 1H), 7.00–7.13 (m, 5H), 7.39 (dd, J = 9.1, 2.8 Hz, 1H), 7.83 (d, J = 9.3 Hz, 1H), 8.01 (s, 1H), 8.27 (s, 1H); 13C NMR (75 MHz, CDCl3) δC: 21.0, 41.8, 55.6, 105.8, 122.4, 124.8, 126.1, 127.1, 129.7, 129.8, 130.8, 137.0, 137.9, 138.4, 145.5, 152.2, 157.9; ESI-MS: m/z. Calcd: 364.09; found: 365.08 (M+).
Acknowledgements
S.P. thanks Department of Science and Technology, New Delhi for Major Research Project SR/S1/OC-50/2011. S.P. also thanks the UGC, New Delhi for the award of BSR Faculty Fellowship.
Notes and references
- For reviews on domino reactions, see:
(a) L. F. Tietze, Chem. Rev., 1996, 96, 15 CrossRef PubMed;
(b) H. Pellissier, Chem. Rev., 2013, 113, 442 CrossRef CAS PubMed;
(c) For selected recent examples of domino reactions, see, L. F. Tietze, G. Brasche and K. Gericke, Domino Reactions in Organic Synthesis, Wiley-VCH, Weinheim, Germany, 2006, ISBN: 3-527-29060-5 Search PubMed;
(d) A. Padwa and S. K. Bur, Tetrahedron, 2007, 63, 5341 CrossRef CAS PubMed;
(e) L. F. Tietze and N. Rackelmann, Pure Appl. Chem., 2004, 76, 1967 CrossRef CAS;
(f) P. Gunasekaran, K. Balamurugan, S. Sivakumar, S. Perumal, J. C. Menéndez and A. I. Almansour, Green Chem., 2012, 14, 750 RSC;
(g) N. Ma, B. Jiang, G. Zhang, S.-J. Tu, W. Wever and G. Li, Green Chem., 2010, 12, 1357 RSC.
- For selected recent example of combinatorial synthesis, see:
(a) L. F. Tietze and M. E. Lieb, Curr. Opin. Chem. Biol., 1998, 2, 363 CrossRef CAS;
(b) F. Vögtle, J. E. Stoddart and M. Shibasaki, Stimulating Concepts in Chemistry, Wiley-VCH, Weinheim, 2000, ISBN: 3527299785 Search PubMed;
(c) L. F. Tietze, N. Rackelmann and G. Sekar, Angew. Chem., Int. Ed., 2003, 42, 4254 CrossRef CAS PubMed.
- L. F. Tietze and A. Modi, Med. Res. Rev., 2000, 20, 304 CrossRef CAS.
- For recent reviews on organic reactions in water medium, see:
(a) C.-J. Li, Chem. Rev., 1993, 93, 2023 CrossRef CAS;
(b) U. M. Lindstrom, Chem. Rev., 2002, 102, 2751 CrossRef PubMedFor recent books for organic reactions in water medium, see,
(c) C. J. Li and T. H. Chan, Comprehensive Organic Reactions in Aqueous Media, John Wiley and Sons, New York, 2007, ISBN 9780471761207 Search PubMed;
(d) P. A. Grieco, Organic Synthesis in Water, Springer, 1998, ISBN: 9780751404104 Search PubMed;
(e) V. K. Ahluwalia and R. S. Varma, Green Solvents for Organic Synthesis, Alpha Science International, Abingdon, UK, 2009, ISBN: 1842655205 Search PubMedFor selected recent examples of organic reaction in water medium, see,
(f) Y. Yang, L. Tang, S. Zhang, X. Guo, Z. Zha and Z. Wang, Green Chem., 2014, 16, 4106 RSC;
(g) P. Wagner, M. Bollenbach, C. Doebelin, F. Bihel, J.-J. Bourguignon, C. Salome and M. Schmitt, Green Chem., 2014, 16, 4170 RSC;
(h) F. Zhang, X. Wu, C. Liang, X. Li, Z. Wang and H. Li, Green Chem., 2014, 16, 3768 RSC;
(i) Y. Sawama, K. Morita, T. Yamada, S. Nagata, Y. Yabe, Y. Monguchi and H. Sajiki, Green Chem., 2014, 16, 3439 RSC;
(j) F. Chen, M. Lei and L. Hu, Green Chem., 2014, 16, 2472 RSC;
(k) M. A. Nasser, M. Salimi and A. A. Esmaeili, RSC Adv., 2014, 4, 61193 RSC.
-
(a) For recent examples on the effect of water in organic reactions, S. Narayan, J. Muldoon, M. G. Finn, V. V. Fokin, H. C. Kolb and K. B. Sharpless, Angew. Chem., Int. Ed., 2005, 44, 3275 CrossRef CAS PubMed;
(b) A. Chanda and V. V. Fokin, Chem. Rev., 2009, 109, 725 CrossRef CAS PubMed;
(c) R. N. Butler and A. G. Coyne, Chem. Rev., 2010, 110, 6302 CrossRef CAS PubMed.
- Antiviral activity: M. N. Zemtsova, A. V. Zimichev, P. L. Trakhtenberg, R. S. Belen'kaya and E. I. Boreko, Pharm. Chem. J., 2008, 42, 571 CrossRef CAS PubMed.
- Antitumor activity:
(a) A. Beauchard, A. Jaunet, L. Murillo, B. Baldeyrou, A. Lansiaux, J. R. Cherouvrier, L. Domon, L. Picot, C. Bailly, T. Besson and V. Thiery, Eur. J. Med. Chem., 2009, 44, 3858 CrossRef CAS PubMed;
(b) A. Bolognese, G. Correale, M. Manfra, A. Esposito, E. Novellino and A. Lavecchia, J. Med. Chem., 2008, 51, 8148 CrossRef CAS PubMed;
(c) J. A. Spicer, S. A. Gamage, G. J. Atwell, G. J. Finlay, B. C. Baguley and W. A. Denny, J. Med. Chem., 1997, 40, 1919 CrossRef CAS PubMed.
- Antibacterial activity: M. Kidwai, K. R. Bhushan, P. Sapra, R. K. Saxena and R. Gupta, Bioorg. Med. Chem., 2000, 8, 69 CrossRef CAS.
- Anti-inflammatory activity:
(a) R. D. Dillard, D. E. Pavey and D. N. Benslay, J. Med. Chem., 1973, 16, 251 CrossRef CAS;
(b) B. J. Mulchin, C. G. Newton, J. W. Baty, C. H. Grasso, W. J. Martin, M. C. Walton, E. M. Dangerfield, C. H. Plunkett, M. V. Berridge, J. L. Harper, M. S. M. Timmer and B. L. Stocker, Bioorg. Med. Chem., 2010, 18, 3238 CrossRef CAS PubMed.
- Antiasthmatic activity: D. Dubé, M. Blouin, C. Brideau, C. Chan, C. Desmarais, D. Ethier, J. P. Falgueyret, R. W. Friesen, M. Girard, Y. Girard, J. Guay, P. Tagari and R. N. Young, Bioorg. Med. Chem. Lett., 1998, 8, 1255 CrossRef.
- Antiproliferative activity:
(a) C.-H. Tseng, Y.-L. Chen, K.-Y. Chung, C.-M. Cheng, C.-H. Wang and C.-C. Tzeng, Bioorg. Med. Chem., 2009, 17, 7465 CrossRef CAS PubMed;
(b) C.-M. Lu, Y.-L. Chen, H.-L. Chen, C.-A. Chen, P.-J. Lu, C.-N. Yang and C.-C. Tzeng, Bioorg. Med. Chem., 2010, 18, 1948 CrossRef CAS PubMed.
- Antimalarial activity:
(a) K. Kaur, M. Jain, R. P. Reddy and R. Jain, Eur. J. Med. Chem., 2010, 45, 3245 CrossRef CAS PubMed;
(b) H. Shiraki, M. P. Kozar, V. Mlelndez, T. H. Hudson, C. Ohrt, A. J. Magill and A. J. Lin, J. Med. Chem., 2011, 54, 131 CrossRef CAS PubMed.
- Antiallergenic activity: T. H. Althuis, S. B. Khadin, L. J. Czuba, P. F. Moore and H. J. Hess, J. Med. Chem., 1980, 23, 262 CrossRef CAS.
- J. B. Jiang and D. Isaacson, Antifungal activity, US Pat. 4656274, 1987.
- MCH1R antagonist:
(a) T. Ulven, P. B. Little, J.-M. Receveur, T. M. Frimurer, Ø. Rist, P. K. Nørregaard and T. Hçgberg, Bioorg. Med. Chem. Lett., 2006, 16, 1070 CrossRef CAS PubMed;
(b) T. Ulven, T. M. Frimurer, J.-M. Receveur, P. B. Little, Ø. Rist, P. Nørregaard and T. Hçgberg, J. Med. Chem., 2005, 48, 5684 CrossRef CAS PubMed;
(c) R. Arienzo, D. E. Clark, S. Cramp, S. Daly, H. J. Dyke, P. Lockey, D. Norman, A. G. Roach, K. Stuttle, M. Tomlinson, M. Wong and S. P. Wren, Bioorg. Med. Chem. Lett., 2004, 14, 4099 CrossRef CAS PubMed.
- Potent HIV-integrace inhibition activity:
(a) J. Polanski, H. Niedbala, R. Musiol, B. Podeszwa, D. Tabak, A. Palka, A. Mencel, J. F. Mouscadet and M. Le Bret, Lett. Drug Des. Discovery, 2007, 4, 99 CrossRef CAS;
(b) J. Polanski, H. Niedbala, R. Musiol, B. Podeszwa, D. Tabak, A. Palka, A. Mencel, J. Finster, J. F. Mouscadet and M. Le Bret, Lett. Drug Des. Discovery, 2006, 3, 175 CrossRef CAS.
- Antioxidant activity: H. R. P. Naik, H. S. B. Naik, T. R. R. Naik, P. J. Bindu, H. R. Naika, T. Aravinda and D. S. Lamani, Med. Chem., 2009, 5, 148 CrossRef CAS.
- A. S. J. Lesage, F. P. Bischoff, C. G. M. Janssen and H. Lavreysen, mGlu 1 receptor activity, PCT Int. Appl. WO 2003082350, 2003.
- E. Ramesh, T. K. S. Vidhya and R. Raghunathan, Tetrahedron Lett., 2008, 49, 2810 CrossRef CAS PubMed.
- W. Zhong, W. Ma and Y. Liu, Tetrahedron, 2011, 67, 3509 CrossRef CAS PubMed.
- P. K. Mahata, C. Venkatesh, U. K. Syam Kumar, H. Ila and H. Junjappa, J. Org. Chem., 2003, 68, 3966 CrossRef CAS PubMed.
- B. Prabhuswamy and S. Y. Ambekar, Synth. Commun., 1999, 29, 3477 CrossRef CAS.
- B. P. Nandeshwarappa, D. B. Aruna Kumar, M. N. Kumaraswamy, Y. S. Ravi Kumar, H. S. B. Naik and K. M. Mahadevan, Phosphorus, Sulfur Silicon Relat. Elem., 2006, 181, 1545 CrossRef CAS.
- B. M. Kiran, B. P. Nandeshwarappa, V. P. Vaidya and K. M. Mahadevan, Phosphorus, Sulfur Silicon Relat. Elem., 2007, 182, 969 CrossRef CAS.
- M. Raghavendra, H. S. B. Naik and B. S. Sherigara, Phosphorus, Sulfur Silicon Relat. Elem., 2008, 183, 1229 CrossRef CAS.
- H. R. P. Naik, H. S. B. Naik, T. R. R. Naik, T. Aravinda, D. S. Lamani and H. Raja Naika, Phosphorus, Sulfur Silicon Relat. Elem., 2009, 184, 2583 CrossRef CAS.
- H. R. P. Naik, H. S. B. Naik, T. R. R. Naik, D. S. Lamani and T. Aravinda, Phosphorus, Sulfur Silicon Relat. Elem., 2010, 185, 663 CrossRef CAS.
- N. J. Parmar, H. A. Barad, B. M. Labana, R. Kant and V. K. Gupta, RSC Adv., 2013, 3, 20719 RSC.
- L. Wu, Y. Wang, H. Song, L. Tang, Z. Zhou and C. Tang, Chem.–Asian J., 2013, 8, 2204 CrossRef CAS PubMed.
- B. Singh, A. Chandra, M. Asthana and R. M. Singh, Tetrahedron Lett., 2012, 53, 3242 CrossRef CAS PubMed.
- For selected recent examples of domino transformations, see:
(a) S. Vivek Kumar and S. Perumal, Tetrahedron Lett., 2014, 55, 3761 CrossRef CAS PubMed;
(b) P. Gunasekaran, S. Indumathi and S. Perumal, RSC Adv., 2013, 3, 8318 RSC;
(c) S. Muthusaravanan, B. Devi Bala and S. Perumal, Tetrahedron Lett., 2013, 54, 5302 CrossRef CAS PubMed;
(d) P. Gunasekaran, P. Prasanna, S. Perumal and A. I. Almansour, Tetrahedron Lett., 2013, 54, 3248 CrossRef CAS PubMed;
(e) P. Prasanna, S. Vivek Kumar, P. Gunasekaran and S. Perumal, Tetrahedron Lett., 2013, 54, 3740 CrossRef CAS PubMed;
(f) S. Indumathi, S. Perumal and J. C. Menéndez, J. Org. Chem., 2010, 75, 472 CrossRef CAS PubMed.
- For selected recent examples of green transformations, see:
(a) S. Muthusaravanan, C. Sasi kumar, B. Devi Bala and S. Perumal, Green Chem., 2014, 16, 1297 RSC;
(b) P. Prasanna, S. Perumal and J. C. Menéndez, Green Chem., 2013, 15, 1292 RSC;
(c) S. Indumathi, S. Perumal and N. Anbananthan, Green Chem., 2012, 14, 3361 RSC;
(d) B. Devi Bala, S. Michael Rajesh and S. Perumal, Green Chem., 2012, 14, 2484 RSC;
(e) S. Michael Rajesh, B. Devi Bala, S. Perumal and J. C. Menéndez, Green Chem., 2011, 13, 3248 RSC;
(f) S. Vivek Kumar, P. Prasanna and S. Perumal, Tetrahedron Lett., 2013, 54, 6651 CrossRef CAS PubMed.
- For selected examples of GAP chemistry, see:
(a) P. Kaur, S. Pindi, W. Wever, T. Rajale and G. Li, Chem. Commun., 2010, 46, 4330 RSC;
(b) P. Kaur, S. Pindi, W. Wever, T. Rajale and G. Li, J. Org. Chem., 2010, 75, 5144 CrossRef CAS PubMed;
(c) P. Kaur, W. Wever, S. Pindi, R. Milles, P. Gu, M. Shi and G. Li, Green Chem., 2011, 13, 1288 RSC;
(d) Y. Zou, Y. Hu, H. Liu and D. Q. Shi, ACS Comb. Sci., 2012, 14, 38 CrossRef CAS PubMed;
(e) H.-Y. Wang and D.-Q. Shi, ACS Comb. Sci., 2013, 15, 261 CrossRef CAS PubMed;
(f) H. Wang, X. Liu, X. Feng, Z. Huang and D. Shi, Green Chem., 2013, 15, 3307 RSC;
(g) J.-J. Zhang, X. Feng, X.-C. Liu, Z.-B. Huang and D.-Q. Shi, Mol. Diversity, 2014, 18, 727 CrossRef CAS PubMed;
(h) M. Chennapuram, N. R. Emmadi, C. Bingi, J. B. Nanubolu and K. Atmakur, Green Chem., 2014, 16, 3237 RSC;
(i) J. Liu, H.-R. Zhang, X.-R. Lin, S.-J. Yan and J. Lin, RSC Adv., 2014, 4, 27582 RSC.
- ESI.†.
Footnote |
† Electronic supplementary information (ESI) available: Copies of 1H and 13C NMR spectra. CCDC 991643. For ESI and crystallographic data in CIF or other electronic format see DOI: 10.1039/c5ra04795a |
|
This journal is © The Royal Society of Chemistry 2015 |