DOI:
10.1039/C5RA02368H
(Paper)
RSC Adv., 2015,
5, 27867-27873
Switchable electrochromic devices based on disubstituted bipyridinium derivatives†
Received
6th February 2015
, Accepted 9th March 2015
First published on 9th March 2015
Abstract
Gel switchable electrochromic devices based on disubstituted bipyridinium derivatives have been developed. The type of substituent from the 4,4′-bipyridinium cation and counter-ions can influence the reversibility and switching of colours of these electrochromic systems. Three switching colours (yellow, blue and red or violet) can be obtained for iodide salts due to dimer formation. In the case of bistrifluoromethanesulfonylimide [NTf2] salts only two reversible colour switches (transparent and blue) were observed. Complementary electrochromic performance including chromatic contrast (ΔT%); transition times between the bleached and coloured states; colouration efficiencies (CE), colour coordinates (CIE L*a*b*) and preliminary cycling stability studies of the devices have been performed. The higher coloration efficiency is observed in the case of [(C10)2bpy][NTf2]2 (186.9 cm2 C−1), which is similar to other bipyridinium systems already reported in the literature.
Introduction
In the past few years, electrochromic materials1 have gained increasing interest due to their practical applications in devices such as electrochromic windows,2 displays,3,4 anti-glare mirrors,5,6 eye-glasses,7 solar attenuated windows,8,9 flexible electrochromic devices,10 among others.
Bipyridinium derivative salts constitute an attractive family of organic compounds, presenting a large range of applications in liquid crystals,11–17 coordination polymers,18–21 antimicrobial agents,22–24 herbicides,25,26 redox indicators27 as well as functional organic electrochromic materials.28–31 In particular, 4,4′-bipyridinium salts, also known as viologens, showed a very good electrochromic contrast, switching speed, stability and inherent high turnover.32–35 In solution, viologen (V) salts exhibit three redox states: dication (V2+), a radical cation (V+˙, blue) and a neutral compound (V0), owing to them undergoing two successive electron-transfer reactions.36
Viologen radical cations dimerise (spin–pair) in aqueous solutions provoking a colour change between blue (monomer) and red (dimer).37,38 At the same time, it can be observed by UV-Vis spectroscopy a mixture of both species (radical cation and dimer). Several publications reported that the anodic oxidation process is not very fast for the dimer.39,40
Scheme 1A, illustrates symmetric electrochromic devices based on viologens switching from transparent to blue. These types of devices are easy to construct because the electrochrom is incorporated in the gel electrolyte and no film deposition is required. In scheme 1B a typical voltammogram of these kinds of devices is shown. The voltammogram is symmetric as consequence of the potential alternates between two identical electrodes. Assuming that the device is submitted to an increasing potential from 0 to +2 V, no current or colour change is observed up to ca. +1.3 V. After this potential, an increasing of anodic current takes place accompanied by the appearance of a blue colour in one of the faces of the device.41 If the electrolyte is transparent the device as a whole exhibits a blue colour. However, it is possible to make the electrolyte opaque and white by addition of a specific pigment such as titanium dioxide. In this case, one face is blue and the other only slightly blue.42 When the potential is reversed a cathodic current is observed. At 0 V a state equivalent to the open circuit is reached designated in Scheme 1 as “silent state”. Starting from 0 to −2 V the same occurs symmetrically, now in the other face of the device. No evidence for the formation of the neutral and colourless viologen species have been achieved.
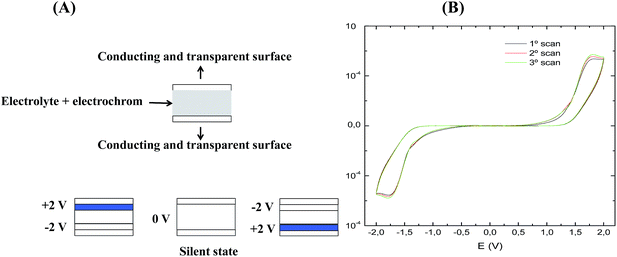 |
| Scheme 1 (A) Symmetric electrochromic device with the electrochrom incorporated in the gel electrolyte. The oxidized form is transparent or pale yellow, while the reduced one is blue as occurring in many viologens. (B) Typical voltammogram of these electrochromic devices containing a bipyridinium salt. | |
A third switching state can be observed in the case of viologens due to the formation of the dimer, as indicated in eqn (1).
Several research groups have been reported that the switching colours of the bipyridinium salts are function of the nitrogen substituent of dication and counter anions.1,8,9,18–21,28–31,43–45 In this context, our group described the development of electrochromic ionic liquids based on symmetric and non-symmetric disubstituted alkyl- and oxo-bipyridinium cations combined with iodide or bromide, bis(trifluoromethanesulfonyl)imide [NTf2] and docusate [AOT] as anions.46,47 The most promising electrochromic bipyridinium ionic liquids have been tested in efficient liquid and solid state electrochromic devices using an adequate electrolyte.46,47 Previously, two classes of intrinsically electrochromic ionic liquids based on ethylenediaminotetraacetic (EDTA) metal complexes ([Cation][Co(EDTA)])48 and vanadate oxide([Cation][VO3])49 were also reported.
|
MV+˙ ⇌ MV+˙ − MV+˙ (equilibrium monomer − dimer)
| (1) |
Herein, we present switchable electrochromic devices based on symmetrical disubstituted bipyridinium salts combined with iodide and [NTf2] anions. Fig. 1 illustrates the symmetric disubstituted bipyridinium salts tested in solid electrochromic devices. According to the selection of functional side chain groups (n-alkyl or ether) of the cation, as well as, the type of anion, different contrast colours and reversible processes of the prepared electrochromic devices can be achieved.
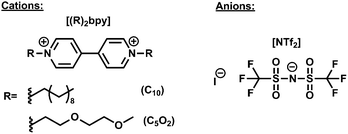 |
| Fig. 1 Selected symmetric disubstituted bipyridinium salts used to build electrochromic devices. | |
Results and discussion
Electrochromic devices
Preparation of electrochromic devices. The electrochromic devices consisted of two PET-ITO layers separated by a thickness of 0.23 mm spacer using a double sided adhesive tape (as presented in Scheme 1A).The conductive face of PET-ITO layer (electroactive area of 2 × 1.5 cm2) was coated with disubstituted bipyridinium salts (≈0.07 M) dissolved in adequate gel electrolyte.46 The devices were sealed with another PET-ITO layer, where the conductive face is in contact with the gel (composed by electrochromic material and the electrolyte).
Iodide based on bipyridinium salts. The salts [(C10)2bpy]I2 and [(C5O2)2bpy]I2 were tested in electrochromic devices. In the case of [(C10)2bpy]I2, the voltammogram is shown in Fig. 2, as well as the switch of colours between different redox states. In this case, peculiar two switches of colours between yellow to blue (characteristic radical cation formation, V+˙) and blue to red (attributed to dimer formation, V+˙ − V+˙) were observed. During the first cycle in the anodic scan (from 0 to +2 V) no electrochemical response occurs until ca. +1.5 V, where the blue colour of the radical cation (first electron transfer of disubstituted bipyridinium salts) is detected. Nevertheless after reach +2 V the red dimer is clearly observed. This dimer is thermally formed, the reason for that is when the potential is hold at +1.5 V it starts to appear slowly. In the anodic back scan the oxidation occurs firstly for the radical cation, followed by the dimer (after ca. +0.5 V). These oxidation processes corresponds to a switch of colour back to yellow (initial colour, V2+). Identical behavior takes place in the other face of the device, due to its symmetry. This redox process is fully reversible during three cycles as observed in Fig. 2. In summary, the oxidation of the dimer is more difficult than the one of the radical cation. This behavior can be explained considering that the dimer is stabilized by the covalent bond involving the two unpaired electrons as indicated in Scheme 2.
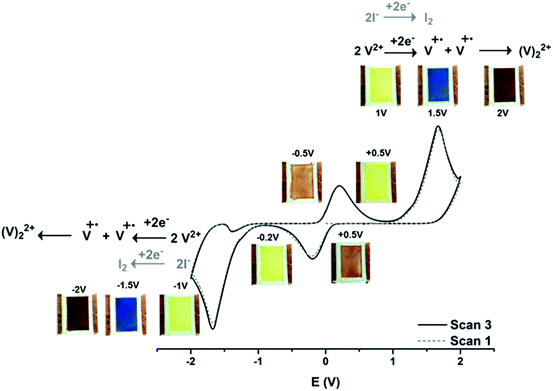 |
| Fig. 2 Voltammogram of [(C10)2bpy]I2 in electrochromic device with respective switch of colours at scan range between 0/+2/−2/0 at 20 mV s−1. | |
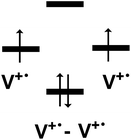 |
| Scheme 2 Representation of the molecular orbital diagram for the viologen dimer (V+˙ − V+˙) from the radical cations (V+˙). | |
In Scheme 3 the kinetic analysis of the system is shown. During the oxidation process, route 1 (radical cation oxidation process) occurs before route 2 (dimer oxidation process).
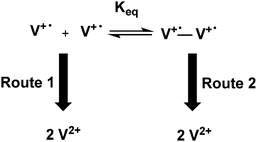 |
| Scheme 3 Kinetic representation of the oxidation process in the device. | |
Consequently, the blue colour disappears before the red. This red colour only vanishes when a sufficiently high potential, to oxidize the dimer, is reached. The proposed oxidation process in the device can be possible if the dimer equilibrium process is slower than route 1 and 2, which it is a reasonable assumption in gel state.
Symmetric electrochromic device containing [(C5O2)2bpy]I2 showed a complete reversible electrochromic behavior (see ESI†) and the switch of colours are between yellow to blue (radical cation, V+˙) and from blue to violet (dimer, V+˙ − V+˙) as indicated in Fig. 3. Starting from initial state (V2+, yellow colour, 0 V) and applying +1.5 V, the blue colour appears, followed the formation of the dimer (V+˙ − V+˙, violet colour, +2 V). When the current is reversed the colour changes back to blue by oxidation of the radical cation. At 0 V the colour return to the original state (V2+, yellow colour) by oxidation of the dimer. This redox process is fully reversible during three cycles (see ESI†).
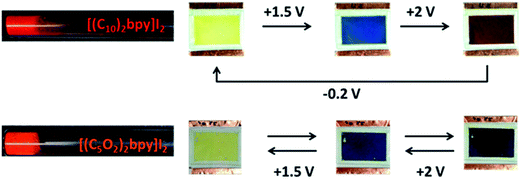 |
| Fig. 3 Switch of colours of the different symmetrical disubstituted bipyridinium salts combined with iodide. | |
Bis(trifluorometanesulfonyl)imide, [NTf2] based on bipyridinium salts. An anion exchange from halides to [NTf2] can modify some thermal and physical properties of the final salts, such as their melting points, thermal stabilities and solubilities. [(C10)2bpy][NTf2]2 and [(C5O2)2bpy][NTf2]2 were also tested in electrochromic devices.46,47Fig. 4 illustrates the switch of colour of these salts [(C10)2bpy][NTf2]2 and [(C5O2)2bpy][NTf2]2 from transparent (V2+) to blue (slight intensity). The appearance of the blue colour can be explained by the reduction of the dication to radical cation. When the potential is increased to +2 V, the blue colour became more intense, probably due to an increase on radical cation concentration. Besides that, the radical cation concentration increases with the increase on the number of cycles and allowing the dimerisation process. Therefore, on the 3rd cycle an oxidation peak (ca. 0 V) corresponding to the oxidation of the dimer appears on the voltammogram (see ESI†).
 |
| Fig. 4 Switch of colours of the different symmetrical disubstituted bipyridinium salts combined with bis(trifluorometanesulfonyl)imide. | |
Electrochromic performance
Chromatic contrast and coloration efficiency of the devices. Some electrochromic performance parameters such as chromatic contrast (ΔT, %), transition times between the bleached and coloured states as well as the coloration efficiency (CE) is presented in Table 1 for all the switchable electrochromic devices.
Table 1 Electrochromic performance (chromatic contrast, transition time and coloration efficiency) of the devicesa
Salts |
Eapp (V) |
ΔT (%) |
t90 [t70] (s) |
CE (cm2 C−1) [λ, nm] |
Eapp: applied electrical potential (in volts); ΔT: transmittance contrast; t90 or t70: time taken for the transmittance change by 90 or 70% of the total difference between the bleached and coloured states (in seconds); CE: coloration efficiency (in cm2 C−1); λ: wavelength of reduced coloured species (in nanometers). |
[(C10)2bpy]I2 |
1.8 |
72.7 |
12.8 [6.5] |
102.6 [550] |
1.4 |
45.3 |
53.3 [33.0] |
45.9 [605] |
[(C10)2bpy][NTf2]2 |
2 |
66.9 |
109.0 [46.0] |
186.9 [605] |
[(C5O2)2bpy]I2 |
1.8 |
71.3 |
9.7 [4.9] |
100.5 [521] |
[(C5O2)2bpy][NTf2]2 |
2 |
51.1 |
93.0 [37.0] |
95.3 [605] |
In general, the introduction of oxygen in the bipyridinium cation scaffold ([(C5O2)2bpy]) seems to reduce transmittance contrasts and coloration efficiencies of the devices. The higher coloration efficiency is observed in the case of [(C10)2bpy][NTf2]2 (186.9 cm2 C−1). The examples based on iodide bipyridinium salts showed faster transition times between the bleached and coloured states of the devices comparing with NTf2 examples.
Similar electrochromic performances of the devices have been described in the literature for other bipyridinium salts as described in detail above. In particular, the well-known electrochromic devices based on methylviologen is reported with 176 cm2 C−1.45 Recently, Ho and collaborators50 reported a new complementary electrochromic device (ECD) based on poly(butyl viologen) (PBV) and Prussian blue (PB) confined to the electrode surfaces. This new PBV–PB ECD system containing a solid-state electrolyte showed good optical contrast with a coloration efficiency of ca. 163 cm2 C−1 at 650 nm. The transmittance of the ECD at 650 nm changed from 73% (bleached) to 8% (darkened), with an applied potential of 1.7 V across the two electrodes. Fitzmaurice and collaborators51 described the construction of an electrochromic window based on a modified transparent nanostructured metal oxide film (TiO2) supported on conducting glass. This nanostructured TiO2 film adsorption of a monolayer of the redox chromophore bis-(2-phosphonoethyl)-4,4-bipyridinium dichloride. The authors reported 170 cm2 C−1 at 608 nm as colouration efficiency of this device. Pozo-Gonzalo et al.52 reported two materials based on viologen (1,10-diethyl-4,40-bipyridinium diperchlorate; 1,10-dioctadecyl-4,40-bipyridinium diperchlorate) where the maximum transparency of the device was 80% in the bleach state and a coloration efficiency (CE) of 136.6 cm2 C−1.
Colour coordinates of the devices. Table 2 summarizes the colour space coordinates (L*a*b) of each electrochromic devices. In general, L*a*b colour space is defined as a colour-opponent space with dimension L for lightness and a and b for the colour-opponent dimensions, based on nonlinearly compressed coordinates. The three coordinates of CIE L*a*b* represent the lightness of the color (L* = 0 yields black and L* = 100 indicates diffuse white; specular white may be higher), its position between red/magenta and green (a*, negative values indicate green while positive values indicate red/magenta) and its position between yellow and blue (b*, negative values indicate blue and positive values indicate yellow).
Table 2 Colour space coordinates (CIE L*a*b*) of the devicesa
Salts |
Eapp (V) |
L* |
a* |
b* |
Eapp: applied electrical potential (in volts); L: represents lightness; a: positive values indicate amounts of red/magenta while negative values indicate amounts of green; b: yellow is positive and blue is negative. |
[(C10)2bpy]I2 |
0 |
84 |
−7.9 |
18.7 |
−1.4 |
69.9 |
−10.5 |
−0.6 |
−1.8 |
22.1 |
38.6 |
−4.5 |
[(C10)2bpy][NTf2]2 |
0 |
90.7 |
−3.0 |
5.5 |
−2 |
73.0 |
−6.7 |
−17.5 |
[(C5O2)2bpy]I2 |
0 |
90.4 |
−9.2 |
30.3 |
−1.4 |
44.3 |
20.2 |
−4.4 |
−2 |
2.5 |
30.7 |
−6.8 |
[(C5O2)2bpy][NTf2]2 |
0 |
90.1 |
−2.8 |
4.7 |
−2 |
44.0 |
23.0 |
−35.9 |
In our case, all values were determined using spectroelectrochemistry experiments (see ESI†) between 0 to +2.0 V and 0 to −2.0 V.
Stability of the devices. Preliminary cycling stability studies of the devices were performed in the cases of [(C10)2bpy]I2 and [(C5O2)2bpy][NTf2]2. These studies indicate a modest stability performance in the case of iodide based bipyridinium salt with around 20% of decrease in the absorbance between 1st and 100th cycles. However, NTf2 based bipyridinium salt showed a good cycling stability during 70 cycles (cycling stability figures are included in ESI†).
Experimental
Materials and characterization
All solvents were used as supplied, acetonitrile was purchased from J.T. Baker, and methanol was purchased from Carlo Erba Reagents. Dichloromethane and acetone were purchased from Sigma-Aldrich.
4,4′-Bipyridine (98%), 2-(2-methoxyethoxy)ethanol, lithium bis(trifluoromethanesulfonyl)imide were purchased from Sigma-Aldrich. 2-(2-methoxyethoxy)ethyl iodide were prepared in two steps according previous reported synthetic method.53
General synthesis of symmetric bipyridinium salts
The salt [(C10)2bpy]I2 was recovered from the crude mixture by recrystallization of the monocation salt using acetone. The desired salt was dried in vacuum. The salt [(C5O2)bpy]I2 was prepared directly by nucleophilic substitution. To a solution of 4,4′-bipyridine (1 equiv.) in acetonitrile was added slowly 1-iodo-2-(2-methoxyethoxy)ethane in excess (3 equiv.). The reaction was vigoursly stirred in reflux 24 to 48 hours. The final product was precipitated with diethyl ether after cooling process. Then, the solid was washed with diethyl ether and dried in vacuum during 24 h.46,47
Anionic exchange of symmetric bipyridinium salts
Symmetric bipyridinium di-iodide salts previously prepared were dissolved in water (or methanol) and lithium bis(trifluoromethanesulfonyl)imide (2.3 equiv.) was added. After addition, the reaction was vigoursly stirred at least 24 hours at room temperature (or 60 °C in the case of docusate anion). Normally, the final product was recovered by filtration or suitable extraction with water/dichloromethane. In the case of extracted product, the organic layer was dried over anhydrous MgSO4, filtered and the solvent was evaporated to give the desired pure product. In order to eliminate any residual inorganic salts, all final NTf2 salts were completely washed with water. The presence of halides was tested by addition of aqueous solution of AgNO3.
Electrochemical studies
Electrochemical studies were performed on an Autolab PGSTAT 12 potentiostat/galvanostat, controlled with GPES software version 4.9 (Eco-Chemie), using the PET-ITO layers as electrodes.
Spectroelectrochemistry studies
Spectroelectrochemistry studies were performed using UV-Vis-NIR spectrophotometer Varian Cary 5000. All spectra were included in ESI† (for 350 or 400 to 700 nm of selected wavelength; NIR bands were not observed). The potential were controlled with a device potentiostat/galvanostat Model 20 Autolab. The device was placed in the spectrophotometer compartment perpendicularly to the light beam. The potentiostat/galvanostat applied an electric potential appropriated and previous selected potentials from CV, and the spectrophotometer registered the absorbance in the UV-Vis-NIR and then at the wavelengths selected for each experiment within the range of the equipment. Cycling stability tests were also performed in the same setup.
Conclusions
The selected symmetric bipyridinium dications and their adequate combinations with different counter-ions can influence significantly the colour contrast intensity and electrochromic reversibility. In this work, alkyl or ether symmetric disubstituted bipyridinium salts were tested as electrochromic devices. According to use of iodide or [NTf2] anions can be possible to achieve a reversible switch between two or three states respectively. In the case of iodide bipyridinium salts, the switching of colours is between yellow to blue corresponding to radical cation step and from blue to red (alkylbipyridinium) or violet (oxobipyridinium) attributed to dimer formation. Interestingly, the electrochromic device based on [(C10)2bpy]I2 showed that the oxidation of the dimer was more difficult than the one of the radical cation because the dimer is stabilized by the covalent bond involving the two unpaired electrons. The blue colour (radical cation oxidation process) disappears before the red (dimer oxidation process), which only disappears when a sufficiently high potential to oxidize the dimer is reached. Contrarily, the electrochromic device based on [(C5O2)bpy]I2 presented a fully reversible electrochromism between yellow, blue (radical cation) and violet (dimer). In the case of NTf2 based bipyridinium salts only the conventional switch between transparent to blue was observed. Electrochromic performances indicate a similar chromatic contrast (ΔT%) as well as colouration efficiencies (CE) of our devices comparing with some bipyridinium derivatives from the literature. In general, it seems that the introduction of oxygen in the bipyridinium cation scaffolds contribute to lower transition times between the bleached and coloured states and colouration efficiencies. Similar colouration efficiencies have been reported in the literature comparing with our best result (186.9 cm2 C−1 for the case of [(C10)2bpy][NTf2]2).
Taking advantage of this bipyridinium electrochromic behaviour and the possibility to improve the preliminary cycling stability results, it is possible to develop switchable and efficient electrochromic materials for application in different surfaces (e.g. glass or PET) with good reversibility as well as colour contrast.
Acknowledgements
The authors would like to thank for FCT-MCTES (Projects PTDC/CTM-NAN/120658/2010, PTDC/CTM/103664/2008 and PEst-C/EQB/LA0006/2013) and Solchemar Company. The NMR spectrometers are part of The National NMR Facility, supported by Fundação para a Ciência e a Tecnologia (RECI/BBB-BQB/0230/2012).
Notes and references
- R. J. Mortimer, Chem. Soc. Rev., 1997, 26, 147 RSC.
- G. A. Niklasson and C. G. Granqvist, J. Mater. Chem., 2007, 17, 127 RSC.
- R. J. Mortimer, A. L. Dyer and J. R. Reynolds, Displays, 2006, 27, 2 CrossRef CAS PubMed.
- X. J. Wang, W. M. Lau and K. Y. Wong, Appl. Phys. Lett., 2005, 87(113502), 1 Search PubMed.
- D. R. Rosseinsky and R. J. Mortimer, Adv. Mater., 2001, 13, 783 CrossRef CAS.
- N. R. Lyman and A. Agrawal, in: Large Area Chromogenics: Materials and devices for Transmittance Control, ed. C. M. Lampert and C. G. Granqvist, SPIE Optical Engineering Press, Bellingham, Washington, USA, 1990, p. 46 Search PubMed.
- C. Ma, M. Taya and C. Xu, Polym. Eng. Sci., 2008, 48, 2224 CAS.
- A. L. Dyer, C. R. G. Grenier and J. R. Reynolds, Adv. Funct. Mater., 2007, 17, 1480 CrossRef CAS.
- C. Pozo-Gonzalo, D. Mecerreyes, J. A. Pomposo, M. Salsamendi, R. Marcilla, H. Grande, R. Vergaz, D. Barrios and J. Sánchez-Pena, Sol. Energy Mater. Sol. Cells, 2008, 92, 101 CrossRef CAS PubMed.
- L. Gomes, A. Marques, A. Branco, J. Araujo, M. Simoes, S. Cardoso, F. Silva, I. Henriques, C. A. T. Laia and C. Costa, Displays, 2013, 34, 326 CrossRef CAS PubMed.
- G. Casella, V. Causin, F. Rastrelli and G. Saielli, Phys. Chem. Chem. Phys., 2014, 16, 5048 RSC.
- M. Bonchio, M. Carraro, G. Casella, V. Causin, F. Rastrelli and G. Saielli, Phys. Chem. Chem. Phys., 2012, 14, 2710 RSC.
- G. Casella and G. Saielli, New J. Chem., 2009, 35, 1453 RSC.
- V. Causin and G. Saielli, J. Mol. Liq., 2009, 145, 41 CrossRef CAS PubMed.
- V. Causin and G. Saielli, J. Mater. Chem., 2009, 19, 9153 RSC.
- E. Marotta, F. Rastrelli and G. Saielli, J. Phys. Chem. B, 2008, 112, 16566 CrossRef CAS PubMed.
- G. Saielli, J. Phys. Chem. A, 2008, 112, 7987 CrossRef CAS PubMed.
- R. J. Mortimer, Annu. Rev. Mater. Res., 2011, 41, 241 CrossRef CAS.
- O. Toma, N. Mercier and C. Botta, Eur. J. Inorg. Chem., 2013, 7, 1113 CrossRef.
- Y. Tan, Z. Y. Fu, Y. Zeng, H. J. Chen, S. J. Liao, J. Zhang and J. C. Dai, J. Mater. Chem., 2012, 22, 17452 RSC.
- Y. Chen, Z. Yang, X. Y. Wu, C. Y. Ni, Z. G. Ren, H. F. Wang and J. P. Lang, Phys. Chem. Chem. Phys., 2011, 13, 5659 RSC.
- L. E. Ator, M. C. Jennings, A. R. McGettigan, J. J. Paul, W. M. Wuest and K. P. C. Minbiole, Bioorg. Med. Chem. Lett., 2014, 24, 3706 CrossRef CAS PubMed.
- M. C. Grenier, R. W. Davis, K. L. Wilson-Henjum, J. E. LaDow, J. W. Black, K. L. Caran, K. Seifert and K. P. C. Minbiole, Bioorg. Med. Chem. Lett., 2012, 22, 4055 CrossRef CAS PubMed.
- R. D. De La Fuente, N. Sonawane, D. Arumainayagam and A. S. Verkman, Br. J. Pharmacol., 2006, 149, 551 CrossRef CAS PubMed.
- A. D. Kennedy and L. A. Summers, J. Heterocycl. Chem., 1981, 18, 409 CrossRef.
- L. A. Summers, Bipyridinium Herbicides, Academic Press, New York, 1980, p. 450 Search PubMed.
- B. M. Vittimberg, U.S. Pat, US 07,241,811, Dez 11, 1990.
- P. Y. Chen, C. S. Chen and T. H. Yeh, J. Appl. Polym. Sci., 2014, 131(40485), 1 Search PubMed.
- R. Sydam, M. Deepa and A. G. Joshi, Org. Electron., 2013, 14, 1027 CrossRef CAS PubMed.
- G. Wang, X. K. Fu, J. Huang, C. L. Wu, L. Wu and Q. L. Du, Org. Electron., 2011, 12, 1216 CrossRef CAS PubMed.
- S. H. Kim, J. S. Bae, S. H. Hwang, T. S. Gwon and M. K. Doh, Dyes Pigm., 1997, 33, 167 CrossRef CAS.
- V. Jain, M. Khiterer, R. Montazami, H. M. Yochum, K. J. Shea and J. R. Heflin, ACS Appl. Mater. Interfaces, 2009, 1, 83 CAS.
- D. Corr, U. Bach, D. Fay, M. Kinsella, C. McAtamney, F. O'Reilly, S. N. Rao and N. Stobie, Solid State Ionics, 2003, 165, 315 CrossRef CAS PubMed.
- S. H. Lee, R. Deshpande, P. A. Parilla, K. M. Jones, B. To, A. H. Mahan and A. C. Dillon, Adv. Mater., 2006, 18, 763 CrossRef CAS.
- S. Y. Choi, M. Mamak, N. Coombs, N. Chopra and G. A. Ozin, Nano Lett., 2004, 4, 1231 CrossRef CAS.
- P. M. S. Monk in The viologen: Physicochemical Properties, Synthesis and Applications of the Salts of 4,4′-bipyridine, J. Wiley and Sons, Chichester, 1998 Search PubMed.
- P. M. S. Monk, N. M. Hodgkinson and S. A. Ramzan, Dyes Pigm., 1999, 43, 207 CrossRef CAS.
- P. M. S. Monk, R. D. Fairweather, M. D. Ingram and J. A. Duffy, J. Chem. Soc., Perkin Trans. 2, 1992, 2039 RSC.
- R. J. Mortimer, Electrochim. Acta, 1999, 44, 2971 CrossRef CAS.
- K. S. Schanze, R. Ramamurthy and V. Ramamurthy in Semiconductor Photochemistry And Photophysics/Volume Ten in Molecular and Supramolecular Photochemistry, ed. V. Ramamurthy and Kirk S. Schanze, CRC Press, New York, U.S., 2005 Search PubMed.
- The signal of the current depends on the face where reduction takes place. When the potential is reverted and the process occurs in the other face the signal is opposite independently if it is an anodic or cathodic current.
- The electrolyte layer is thin and we have some perception of the other face.
- C. L. Bird and A. T. Kuhn, Chem. Soc. Rev., 1981, 10, 49 RSC.
- N. M. Rowley and R. J. Mortimer, Sci. Prog., 2002, 85, 243 CrossRef CAS.
- P. M. S. Monk, R. J. Mortimer and D. R. Rosseinsky, in Electrochromism and Electrochromic Devices, Cambridge University Press, Cambridge, 2007 Search PubMed.
- N. Jordão, L. Cabrita, F. Pina and L. C. Branco, Chem.–Eur. J., 2014, 20, 3982 CrossRef PubMed.
- N. Jordão, H. Cruz, A. Branco, F. Pina and L. C. Branco, ChemPlusChem, 2015, 80, 202 CrossRef.
- A. Branco, L. C. Branco and F. Pina, Chem. Commun., 2011, 47, 2300 RSC.
- A. Branco, J. Belchior, L. C. Branco and F. Pina, RSC Adv., 2013, 3, 25627 RSC.
- T.-H. Kuo, C.-Y. Hsu, K.-M. Lee and K.-C. Ho, Sol. Energy Mater. Sol. Cells, 2009, 93, 1755 CrossRef CAS PubMed.
- R. Cinnsealach, G. Boschloo, S. N. Rao and D. Fitzmaurice, Sol. Energy Mater. Sol. Cells, 1998, 55, 215 CrossRef CAS.
- C. Pozo-Gonzalo, M. Salsamendi, A. Viñuales, J. A. Pomposo and H.-J. Grande, Sol. Energy Mater. Sol. Cells, 2009, 93, 2093 CrossRef CAS PubMed.
- M. Döbbelin, I. Azcune, M. Bedu, A. R. Luzuriaga, A. Genua, V. Jovanovski, G. Cabañero and I. Odriozola, Chem. Mater., 2012, 24, 1583 CrossRef.
Footnote |
† Electronic supplementary information (ESI) available. See DOI: 10.1039/c5ra02368h |
|
This journal is © The Royal Society of Chemistry 2015 |
Click here to see how this site uses Cookies. View our privacy policy here.