DOI:
10.1039/C5RA01425E
(Paper)
RSC Adv., 2015,
5, 30631-30639
Anthraquinone chromophore covalently bonded blocked waterborne polyurethanes: synthesis and application
Received
24th January 2015
, Accepted 25th March 2015
First published on 25th March 2015
Abstract
Dye-bonded blocked waterborne polyurethanes were developed to synchronously realize coloring and finishing of textiles and improve colorfastness using an anthraquinone chromophore and a methyl ethyl ketoxime blocking agent. The number-average molecular weight of the dye-bonded blocked polyurethane is 2303 g mol−1 and the polydispersity is 1.06, indicating a narrow molecular weight distribution. The maximum absorption wavelengths do not shift after the monomolecular chromophores are bonded to the polyurethane. The self-colored polyurethanes provide better centrifugal stability and water solubility than the dye-mixed blocked polyurethanes. The dye-bonded blocked polyurethane offers good thermal stability below 100 °C and undertakes de-blocking at about 150 °C. The covalent bonding of the anthraquinone moiety with the polyurethane chain and temporary blocking of the terminal isocyanate groups are conducive to enhancing the tinting strength and colorfastness of the treated cotton fabrics. The self-colored blocked polyurethanes with good elasticity and toughness can improve the recovery angle of the treated cotton up to 120°, which can also endow the fabric with wrinkle resistance.
1. Introduction
Waterborne polyurethanes (WPUs) show various features such as good film-forming capability, flexibility, easy modification, excellent adhesion and especially environmentally-friendly nature, etc.1,2 As a result, they have received increasing interest in recent years and are widely employed in coating, leather processing, adhesives, elastomers and many other fields.3,4 In terms of textile coating and finishing, WPUs are not only able to efficiently enhance color depth, fastness and vividness, but also can endow fabrics with fullness, antistatic properties, crease resistance, comfort and breathability.5,6 Furthermore, WPUs which are tailored by organic silicon and nano-materials can be utilized in water and oil repellent, antifouling, pilling resistance and other functional finishes, providing added value for textiles. Besides, chromophores can also be introduced into polyurethane chains to obtain self-colored polyurethanes, namely polyurethane-based polymeric dyes, via reaction of isocyanate groups (NCO) in the polyurethane prepolymer and reactive groups in chromophores.7 These chromophores cover reactive dye,8–10 disperse dye,11,12 acid dye and even luminescent dye,13 photochromic dye,14 mechanochromic unit15 and other functional dyes.16,17 The self-colored polyurethanes are featured with both characters of chromophores and polyurethanes, including chromaticity, tinting strength, film-forming, abrasive, heat resistances, photoelectric properties, etc.18 They are potential candidates to substitute traditional colorful waterborne polyurethane coatings that are simply mixed with dye or pigment pastes. Therefore, the self-colored polyurethanes are able to simultaneously realize tinting and functional finishing to meet the demands of modern printing and dyeing industry for power saving, environmental protection and short processing sequence.
Self-colored polymers are a kind of polymeric dyes with chromophores covalently bonded to the main or side chains of the polymers.19 Self-colored polymers can overcome many weaknesses of macromolecular dyes or pigments like low utilization ratio, easy discoloration and poor color fastness.20 Some self-colored polymers provide similar structures with the treated fabrics, showing good compatibility and extraction resistance. The reactive groups in monomer dyes of self-colored polymers may also be chemically bonded with fabrics, for example, through carboxyl (–COOH) or hydroxy (–NH2) groups. However, these self-colored polymers may not be suitable for coloring different fabrics, and those self-colored polymers without reactive groups still show low color fastness. In addition, it may be necessary to bake the treated fabrics at high temperature to achieve fixation. They are therefore unsuitable for temperature sensitive materials and do not conform to the demand for energy conservation and environmental protection.
Isocyanate groups (NCO) exhibit strong activity in chemical reactions and can easily react with compounds containing active hydrogen, such as those containing hydroxyl, amino, and carboxyl groups. NCO groups react vigorously with moisture and generate polyurea, reducing available NCO groups for chain extension and largely retarding the preparation of waterborne polyurethane.21 The development of blocked polyurethane can possibly solve this problem effectively. Blocked polyurethanes may result in longer pot-life and lower film formation temperature than normal polyurethanes. Such technique is widely employed in fabric treating such as fixation finishing of reactive dyeing and acid dyeing for cotton and its blends. The blocked prepolymer is formed by reacting nucleophilic reagents containing active hydrogens as blocking agent with NCO groups, making NCO inactivate temporarily. When heated at a certain temperature, the blocked prepolymer is dissociated.22 The reproduced NCO groups then undergo cross-linking and curing reaction to form thermally more stable bonds with the treated substrates containing active hydrogen-derived functional groups.23 The common blocking agents are ketoxime, phenol, amines, lactams, sodium bisulphite, etc.24 With the idea of blocking polyurethane, the corresponding self-colored blocked polyurethanes can be obtained by reaction with an appropriate blocking agent after dye chromophores have been introduced into the prepolymer. The binding force between self-colored polyurethanes and substrates can be enhanced, improving the color fastness and durability.
The synthesis of a series of self-colored polyurethanes with anthraquinone, azo and fluorescent dyes as chromophores have been reported. For example, the self-colored polyurethanes based on 1,4-bis-(β)-iodopropionyloxy-9,10-anthraquinone were prepared and they showed good film forming ability and good adhesion to glass surfaces.12 Some silicon-containing waterborne polyurethanes grafted with reactive dyes were synthesized and applied in textile dyeing, which displayed lower dye migration and better colorfastness to light.25 A waterborne polyurethane dye with disperse violet 26 was found to have higher thermal stability and fluorescent intensity than micromolecular disperse violet 26.26 Most of these studies were focused on filtering or synthesizing novel micromolecular chromophores which were used to develop the self-colored polyurethanes. However, few reports were directed towards improving the binding strength between the self-colored polyurethanes and textiles and discussing the finishing effect of the self-colored polyurethanes. Therefore, this work aims to develop a novel self-colored, blocked waterborne polyurethane which is able to synchronously realize coloring and finishing of textiles and improve colorfastness. Disperse blue 3 (C.I. 61505) raw powders as chromophore monomers were covalently incorporated with polyurethane chain with methyl ethyl ketoxime (MEKO) as blocking agent. The structure and thermal stability of the synthesized dye-bonded blocked waterborne polyurethane (dye-bonded BWPU) was characterized. Then the dye-bonded BWPU was coated on cotton fabrics. Properties of the treated fabrics including color fastness, winkle resistance and surface morphology were analyzed.
2. Experimental
2.1. Materials
Isophorone diisocyanate (IPDI) was bought from Aladdin (Shanghai) Co., Ltd. Methyldiethanolamine (MDEA), polyethylene glycol 400 (PEG400) and glacial acetic acid (HAc), which were separately used as chain extender, soft segment and neutralizer, were procured from Sinopharm Chemical Reagent Co., Ltd. Disperse Blue 3 raw powder (DB, as shown in Scheme 1) was acquired from FILO Color & Chemicals (Wuxi) Co., Ltd. Methyl ethyl ketoxime (MEKO) employed as blocking agent was purchased from Suzhou Tianke Trading Co., Ltd. Acetone was bought from Sinopharm Chemical Reagent Co., Ltd. PEG400 and acetone were dehydrated under vacuum at 60 °C for 24 h and then kept with 4A molecular sieve before use. Other chemicals were utilized as received.
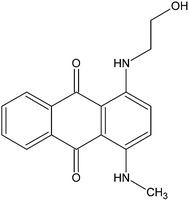 |
| Scheme 1 Structure of Disperse Blue 3 (C.I. 61505). | |
2.2. Preparation of dye-bonded BWPUs
The dye-bonded blocked waterborne polyurethane (sample 1–5) were prepared by a procedure described below. The detailed synthesis route and formulations are displayed in Scheme 2 and Table 1, respectively. PEG400 was mixed with IPDI in a three-necked flask equipped with a mechanical stirrer and a reflux condenser. The reaction was maintained at 80 °C for 1 h to obtain polyurethane prepolymer. Afterwards, the temperature was lowered to 60 °C and MDEA as a chain extender diluted with 3–5 mL acetone was dropwise added into the flask to prevent implosion. The chain-extension reaction was proceeded at 80 °C and continued for 1 h to increase the molecular weight. Subsequently, Disperse Blue dyes were added into the system at 80 °C and mixed for 2 h to form dyed polyurethane, followed by adding charged blocking agent MEKO and conducting at 80 °C for another 1 h. Then HAc, a neutralizer, was added at about 50 °C and stirred for 30 min to neutralize the carboxyl group in the chain. Acetone was removed via a rotary evaporator under reduced pressure. Finally, the dye-bonded BWPUs were obtained after unreacted Disperse Blue dyes were precipitated with methanol and removed by filtration.
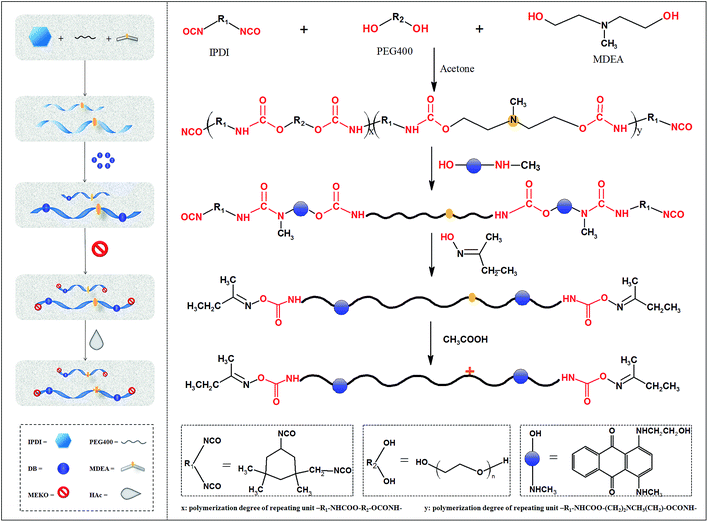 |
| Scheme 2 Synthesis of dye-bonded blocked waterborne polyurethane. | |
Table 1 Formulation of dye-bonded blocked waterborne polyurethane
Samples |
IPDI |
PEG400 |
MDEA |
DYE |
BDO |
MEKO |
1 |
2.5 |
1 |
0.7 |
0.1 |
0 |
1.4 |
2 |
2.5 |
0.7 |
1 |
0.1 |
0 |
1.4 |
3 |
2.5 |
0.4 |
0.8 |
0.3 |
0 |
2 |
4 |
2.5 |
0.7 |
0.7 |
0.4 |
0 |
1.4 |
5 |
2.5 |
0.4 |
0.4 |
0.5 |
0 |
2.8 |
6 |
2.5 |
1 |
0.7 |
0 |
0.1 |
1.4 |
Besides, blocked waterborne polyurethane (BWPU) was also prepared using 1,4-butanediol to take place of Disperse Blue dyes during the synthesis. Other procedures and conditions were the same as above-mentioned. Finally, the synthesized BWPU was further physically mixed with Disperse Blue 3 to obtain dye-mixed BWPU (sample 6).
2.3. Cotton fabrics coating with dye-bonded BWPU
Dye-bonded BWPU in concentration of 0.8 g mL−1 was diluted with deionized water, followed by stirring to obtain a uniform dispersion. The dispersion was applied onto cotton fabrics using printing network and doctor. The process was repeated at least two times in order to make more dye-bonded BWPU uniformly coated on the cotton fabric surface. After application, the coated fabric samples were dried at 60 °C for 4 min and then cured at 150 °C for 5 min. The coating process is shown in Scheme 3.
 |
| Scheme 3 Coating with dye-bonded BWPU for cotton. | |
2.4. Characterization
The chemical structure of samples was studied by a FTIR (NICOLET.is10) in the scanning range of 4000–350 cm−1. The viscous dye-bonded BWPU and monomolecular solid dye were measured via the liquid-film method and the KBr disc technique, respectively.
Gel permeation chromatography (GPC, Waters 1515 Isocratic HPLC, USA) was utilized to estimate the molecular weight of the dye-bonded BWPU with solvent tetrahydrofuran (THF) at 35 °C. The molecular weight spectrogram was then recorded in 190–800 nm scanning wavelength and 0–20 cm3 min−1 flow rate.
Thermo-gravimetric analysis (TGA) was investigated by a Mettler-Toledo SDTA851e thermo-gravimetric analyzer to measure the weight loss of the dye-bonded BWPU. Approximately 15–20 mg of the sample was scanned from 25 to 600 °C at a constant heating rate of 10 °C min−1 in nitrogen atmosphere with a gas flow rate of 10 mL min−1.
The obtained dye-bonded BWPU and monomolecular dye were dispersed in deionized water or ethanol with the same concentration. The visible absorption spectrum of the dispersion was analyzed in 200–800 nm by a UV-vis spectrophotometer (Cary50, Shanghai roachelab, Co., Ltd, China).
Centrifugal stability is estimated by the absorbance ratio. The dye-bonded BWPU was dispersed in deionized water, followed by centrifugalization at 3000 rpm for 15 min. The absorbance at the maximum absorption wavelength was obtained by a UV-visible spectrophotometer (Cary 50, Varian). The absorbance ratio (γ) of the dye was calculated by formula (1).
|
 | (1) |
where
A0 and
A is the absorbance of dye solution before and after centrifugalization, respectively.
The color properties (K/S value, L*, a*, b*, C*, h°) of the coated cotton samples were measured by an Xrite-8400 spectrophotometer under the illuminant D65 using a 10° standard observer. K/S value represents relative color strength.
The rubbing fastness of coated cotton fabrics was tested according to AATCC 8-2007. Samples were separately rubbed for 10 cycles with dry and wet (moisture content 95–105%) standard crocking cloth under 9 N vertical pressure by a crocking fastness tester (Y571, Electron Instrument Co., Ltd., Laizhou, China). The washing fastness was performed on the basis of AATCC Test Method 61-2006 standard with 5 g L−1 soap and 2 g L−1 Na2CO3 at 60 °C for 30 min using a washing fastness tester (Wenzhou Darong Textile Instrument Co., Ltd., China). The colorfastness to light was estimated using AATCC Test Method 16-2004, in which the coated cotton fabrics along with blue wool standards were exposed under artificial light.
The surface morphology of cotton fabrics before and after coating was observed with a scanning electron microscope (SEM, TM3030, Hitachi) under 5000 magnification. The fabric samples were dried and sputter coated with gold using a current of 10 mA.
The hand feeling of cotton fabric is represented by bending rigidity and bending hysteresis moment, which were measured with a pure bending tester (KES-FB 2, Kato Co., Ltd., Japan). The final value was an average of three measurements.
3. Results and discussion
3.1. Structure of dye-bonded BWPU
3.1.1 FTIR spectra. FTIR absorption spectra of dye-bonded BWPU (sample 3), BWPU and DB chromophore are displayed in Fig. 1. The absorption peaks corresponding to DB chromophore (Fig. 1a) can be observed at around 3413 cm−1 (–OH), 3223 cm−1 (–NH–), 2924–2853 cm−1 (–CH2–, –CH3), 1710 cm−1 (C
O, anthraquinone ring), 1600–1425 cm−1 (C
C, anthraquinone ring), 1260 cm−1 (C–N), 1008 cm−1 (C–H in-plane bending vibration, anthraquinone ring) and 725 cm−1 (C–H out-plane bending vibration, anthraquinone ring). In Fig. 1c, the strong absorptions at 3318 cm−1, 1710 cm−1 and 1304 cm−1 are assigned to the stretching band of N–H, C
O and C–O in the urethane group. The new absorption near 1104 cm−1 belongs to the stretching vibration of the ether groups (C–O–C) in the PEG400 soft segments. All the above typical peaks support the formation of polyurethane chains. Additionally, the absence of characteristic stretching vibration at 2270 cm−1 (–NCO) reveals that isocyanate groups have been effectively blocked by the blocking agent. Also, compared with BWPU in Fig. 1b, the appearance of strong bands at around 1008 cm−1 and 725 cm−1 due to C–H in-plane and out-plane bending vibrations in anthraquinone ring confirms that DB chromophore has been successfully introduced into polyurethane chains.
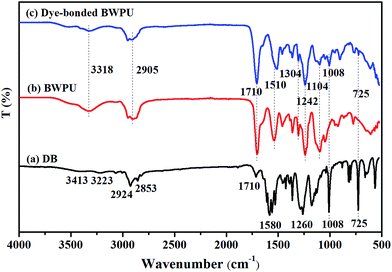 |
| Fig. 1 FTIR spectra of dye-bonded BWPU. | |
3.1.2 Molecular weight. The GPC data of dye-bonded BWPU (sample 3) are summarized in Table 2. The number-average molecular weight (Mn) and weight-average molecular weight (Mw) are 2303 g mol−1 and 2434 g mol−1, respectively, and the polydispersity is 1.06. The average molecular weight is closely related to proportion of diisocyanates in IPDI and hydroxyl in PEG400 and chain extender MDEA. Theoretically, as the proportion of NCO and OH groups is closer to 1
:
1, the molecular weight would infinitely increase. In this sample, the ratios of OH groups and oxime groups between NCO were about 0.48 and 0.4, respectively. NCO groups reacted with dye and blocking agents, revealing the end of polyreaction. The higher the usage of blocking agents, the more NCO groups were blocked, which limited the polyurethane chain growth. Thus, the average molecular weight of the dye-bonded BWPU is relatively low.
Table 2 Molecular weight of dye-bonded blocked waterborne polyurethane
Distribution |
Mn (Daltons) |
Mw (Daltons) |
Mp (Daltons) |
Mz + 1 (Daltons) |
Poly dispersity |
Mz/Mw |
Mz + 1/Mw |
Dye-bonded BWPU |
2303 |
2434 |
1879 |
2783 |
1.06 |
1.07 |
1.14 |
3.1.3 UV-vis spectra. UV-vis absorption spectra of dye-bonded BWPU (sample 3) were recorded in both ethanol and water solutions. The corresponding monomolecular DB dye in ethanol was also measured since DB was insoluble in water. As displayed in Fig. 2, the dye-bonded BWPU and the monomolecular dye in ethanol and water show similar absorption peaks in ultraviolet region and visible region. The maximum absorption wavelengths (λmax) exhibit at 253 nm, 595 nm and 642 nm, associated with the anthraquinone chromophore and auxochrome groups. This phenomenon further confirmed that DB was introduced into the polyurethane chain. Moreover, no significant bathosochromic or hypsochromic shift of λmax was observed between the dye monomer and dye-bonded BWPU, indicating that the n–π* and π–π* transitions in anthraquinone unit did not change before and after covalently bonding to the polyurethane chain. According to a relevant publication,26 a hypsochromic shift appears mainly because auxochromic groups (–NH2) directly conjugated with anthraquinone react with –NCO, influencing the n–π* and π–π* transitions in aromatic rings. Thus, if the active group does not directly link with chromophore, the λmax will not change after the dye monomer is incorporated into the polyurethane chain.
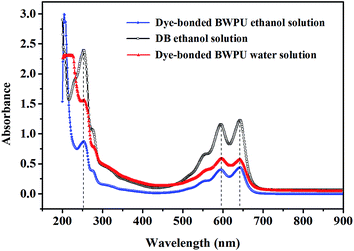 |
| Fig. 2 UV-vis spectra of dye-bonded BWPU and DB in water and ethanol solution. | |
3.2. Stability of dye-bonded BWPU
3.2.1 Centrifugal stability. The absorbance ratio (γ) is applied to represent centrifugal stability which is proportional to the absorbance ratio. As can be observed in Fig. 3, the absorbance ratios of samples 1–3 which are above 90% are higher than those of sample 4 and sample 5, demonstrating that the three dye-bonded BWPU samples provide better centrifugal stability and water solubility. It is ascribed to the hydrophilic chain extender content in the polyurethane chain, which determines the solubility of dye-bonded BWPU. The dye-mixed BWPU (sample 6) with only 71% absorbance ratio shows relatively poor centrifugal stability. This result suggests that the dispersion and storage stabilities of color coatings can be effectively promoted via chemically introducing dye to waterborne polyurethane chains. This method can also simplify the working procedure in application, saving time and cost.
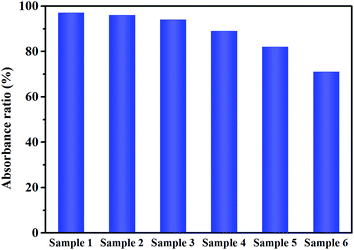 |
| Fig. 3 Centrifugal stability of the dye-bonded blocked polyurethane. | |
3.2.2 Thermal stability. To investigate the thermal stability, TGA analyses of the dye-bonded BWPU (sample 3) and BWPU were carried out, as shown in Fig. 4. It is reported that the thermal degradation of polyurethane generally includes two or three steps, which mainly depends on the structure and compositions of soft segments and hard segments and the stability of the formed urethane bonds.27–29 The TGA trace displays that the dye-bonded BWPU exhibits three stages of thermal deposition in nitrogen atmosphere. It is known that the hard segment is more prone to thermal decomposition than the soft segment in polyurethane.30 The first weight loss rate is about 24% in the range of 100–200 °C due to de-blocking of MEKO blocking agent. The second thermal degradation domain with approximately 21% weight loss between 200–300 °C is attributed to the decomposition of urethane bonds in hard segments (IPDI-Dye-MDEA). The last thermal decomposition rate between 300–450 °C is approximately 45% on account of chain scission of soft segments (PEG400) and dissociation of anthraquinone structure. The temperatures of 50% weight loss (T50) is 310 °C. As to single BWPU, the thermal degradation includes two steps. In the first step, about 25% thermal degradation before 200 °C is also owing to de-blocking of MEKO blocking agent. The second step in the range of 200–400 °C is ascribed to the degradation of polyurethane chain. Compared with BWPU, the thermal stability of dye-bonded BWPU is improved.
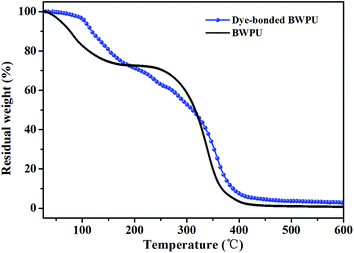 |
| Fig. 4 TGA for dye-bonded blocked polyurethane. | |
Furthermore, the dye-bonded blocked polyurethane (sample 3) was stored at −4 °C, 30 °C, 60 °C, 100 °C and 200 °C for 6 h. These samples were then dissolved in ethanol, respectively, and measured by UV-vis spectra. As displayed in Fig. 5, the λmax appears at 595 nm and 642 nm for the samples stored at −4 °C, 30 °C, 60 °C and 100 °C, which shows no color changes happen in comparison with that of the sample at 25 °C. This result illustrates that the dye-bonded blocked polyurethane exhibits good stability below 100 °C. However, the λmax of the dye-bonded BWPU at 200 °C shifts to 575 nm and the absorption peak at 642 nm almost disappears, the ethanol solution of which presents atropurpureus. This variation may be attributed to re-generated –NCO groups as a result of deblocking at 200 °C. The re-generated –NCO groups are self polymerized to synthesize dimer or trimer. The structures exhibit electron withdrawing effect, contributing to distinct hypsochromic shift of λmax.
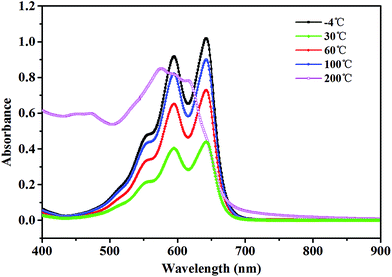 |
| Fig. 5 Thermal stability of dye-bonded blocked polyurethane at different temperature. | |
3.3. Properties of coated cotton fabrics with dye-bonded BWPU
3.3.1 Color property. Six different formulations were employed so as to investigate the influence of dye concentration on the color property of the cotton fabrics coated with the dye-bonded BWPUs. The chromatic values including K/S, L*, a*, b*, C*, and h° are displayed in Table 3.
Table 3 Color properties of dye-bonded BWPUs coated cotton fabrics
Samples |
K/S value |
L* |
a* |
b* |
C* |
h° |
Control |
— |
0.01 |
94.23 |
−0.02 |
3.19 |
3.19 |
90.31 |
1 |
 |
8.44 |
36.04 |
4.53 |
−32.07 |
32.39 |
278.04 |
2 |
 |
10.79 |
31.13 |
5.33 |
−28.45 |
28.94 |
280.61 |
3 |
 |
11.67 |
29.02 |
3.71 |
−23.70 |
23.99 |
278.89 |
4 |
 |
10.38 |
29.22 |
2.70 |
−18.56 |
18.76 |
278.27 |
5 |
 |
1.93 |
57.29 |
0.16 |
−26.52 |
26.52 |
270.34 |
6 |
 |
4.13 |
49.11 |
−0.33 |
−34.73 |
34.74 |
269.46 |
The K/S value of coated fabric with dye-mixed BWPU (sample 6) is 4.13, while K/S of the fabric coated with dye-bonded BWPU (sample 1) is increased to 8.44. The significant improvement of K/S value confirms that dye covalently bonded polyurethane is able to effectively enhance the tinting strength. It can also be observed that as the dye usage increases relatively, the K/S value of the coated fabric (sample 3) can be improved to 11.67. However, compared with other samples, sample 5 shows the lowest color strength. Although the dye usage is the highest, most dyes fail to bond into polymer skeleton, leading to the lowest dye proportion in the polyurethane chains.
Moreover, the color lightness (L*) decrease with increasing dye usage (sample 1–4), which vary inversely with the K/S values. The color chroma (C*) of coated fabrics drops as increasing the dye usage (sample 1–4), which indicates that the colors of the coated fabrics become slightly dim. The hues (h°) of the coated cotton with dye-bonded BWPUs (sample 1–4) are similar, whereas dye-bonded BWPUs (sample 5) and dye-mixed BWPU (sample 6) vary slightly. Red color value (a*) turns smaller while blue color value (−b*) turns lager, which mean the coated fabrics show less red and blue. However, samples 1–4 seem redder and bluer from the corresponding pictures while blue color of samples 5–6 seems to be lighter. This is because the visual effect of color is decided collaboratively by L*, a*, b* and C*. When L* value is constant, the lager the a* value is, the redder the sample is, the smaller the −b* value is, the bluer the sample is, and the lager the C* value is, the higher the color saturation is. If the L* value changes significantly, a*, b* and C* values may not change regularly.
3.3.2 Colorfastness. The colorfastness of cotton fabrics coated with all dye-bonded BWPUs are comparatively analyzed, as listed in Table 4. Results show that the rubbing, washing and light fastness of cotton fabrics coated with dye-mixed BWPU are 3–4 grade, 1 grade and 3 grade, respectively. However, they increase accordingly up to 4–5 grade, 3 grade and 4 grade for dye-bonded BWPU. It is assumed that the color fastness mainly depends on the durability between polymer chain and cotton after chromophore was covalently introduced into polymers, which at least can correspond to fastness grade of reactive dyes. Furthermore, the application of disperse dyes can be expanded as they are chemically bonded with polymers. It can also be found that the color fastness of dye-bonded BWPUs (sample 1–3) improves slightly, some (sample 4–5) even decrease, though the blocking agent is increased. It is interpreted that more blocking agent means more re-generated NCO groups to crosslink with functional OH groups in cotton fibers. Nevertheless, when the amount of NCO group reaches to a certain level, most OH active sites have crosslinked with NCO or been isolated by polyurethane chains. And then the surplus NCO groups conduct self polymerization, thereby physically accumulating on the fiber surface and falling off the fabrics in rubbing or washing process. As a result, the colorfastness reduces.
Table 4 Color fastness of dye-bonded BWPUs coated cotton fabrics
Samples |
Rubbing fastness |
Washing fastness |
Light fastness |
Dry |
Wet |
Discoloring |
Staining |
1 |
4–5 |
4–5 |
3 |
4 |
4 |
2 |
4–5 |
4 |
3 |
4 |
4 |
3 |
4–5 |
4 |
3 |
3 |
4–5 |
4 |
3–4 |
3 |
2 |
2–3 |
4 |
5 |
5 |
4–5 |
2 |
2 |
3–4 |
6 |
4 |
3–4 |
1 |
1 |
3 |
3.3.3 Wrinkle resistance. Fabric finishing generally takes place separately either before or after coloring, often leading to some undesirable consequences. For example, finishing after coloring may change the color tone and strength, while finishing before coloring usually makes it difficult to obtain dark color due to coloring sites filled by finishing agents.31,32 Simultaneous coloring and finishing process provides energy conservation, timesaving, water economy, cost effectiveness and other features, thereby attracting significant research effort.33 Here, the anti-wrinkle effect of cotton fabrics coated by the dye-bonded BWPUs was evaluated, as shown in Fig. 6.
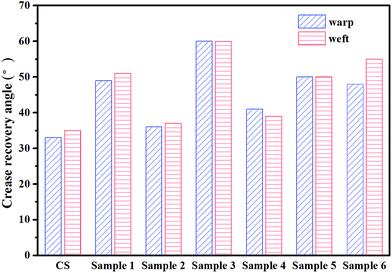 |
| Fig. 6 Crease recovery angle of dye-bonded BWPUs coated cotton fabrics. | |
The test result reveals that the total crease recovery angle (warp + weft) of the uncoated cotton fabric (control sample, CS) is 68°, while the recovery angle of the cotton coated by the dye-bonded BWPU is improved as large as 120° (sample 3). The anti-wrinkle effect of the dye-bonded BWPUs depends on the amount of re-generated NCO groups and the flexibility of polyurethane chains. In the curing process, the reactive NCO groups in the dye-bonded BWPU were released and then reacted with OH groups in cellulose to form cross-links among cellulose chains. The elastic modulus of the coated fabric was increased, reducing the amount of deformation of the coated fabric under a given load. Besides, the retardation of new hydrogen bond caused by OH groups in cellulosic fibers under stress was reduced, leading to high wrinkle recovery. So the synthesized dye-bonded BWPUs are able to color cotton fabrics and simultaneously act as wrinkle resistant finishing agent.
3.3.4 Surface morphology. Fig. 7 shows the surface morphology of cotton fabrics coated with dye-bonded BWPU (sample 3). The surface of the original cotton fiber shows natural grooves and characteristic parallel ridges (Fig. 7a).34 After coated with the dye-bonded BWPUs (Fig. 7b), such characteristics become unnoticeable, and the linear polyurethanes are uniformly distributed on the cellulosic fibers. It can also be seen that some dye-bonded BWPUs are connected with fibers with one terminal of the polyurethane micro fibrils, and others with both terminals. This is because that the re-generated NCO groups in one end or both ends of polyurethane micro fibrils covalently bonded with –OH groups of cellulosic fibers, which enhances the color fastness of treated cotton (as shown in Scheme 4).
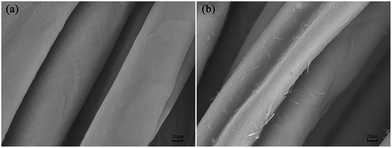 |
| Fig. 7 SEM micrographs of the treated cotton fabrics (a) control cotton; (b) coated cotton. | |
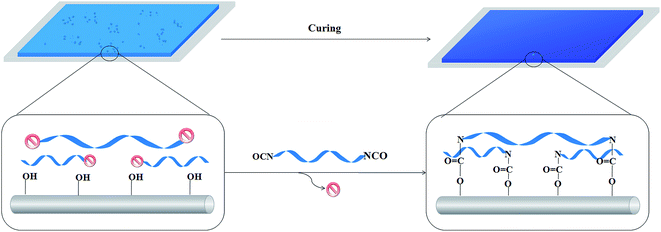 |
| Scheme 4 Coating principle of dye-bonded BWPU on cotton surface. | |
3.3.5 Fabric handle. The bending rigidity and bending hysteresis moment are utilized to evaluate the handle of cotton fabrics coated with dye-bonded BWPU (sample 3). Relevant results are displayed in Table 5. Compared to the control cotton, the bending rigidity and bending hysteresis moment of the coated cotton fabric are distinctly increased, illustrating the reduced softness and poor handle. The dye-bonded BWPU de-blocked and rebuilt reactive NCO groups in the curing process which then reacted with OH in cellulose, thereby confining the free shift of fibers in the fabric. Furthermore, the hard segment ratio shows relatively rigid molecular chain due to high hard segment ratio in the polyurethane chains. Consequently, the handle of the treated cotton becomes poor, while in practice a compromise between fabric handle and wrinkle resistance must be reached, a better balance between the two properties may be possible with soft finishing or increasing the soft segment ratio in dye-bonded BWPUs to improving the chain flexibility.
Table 5 The bending rigidity and bending hysteresis moment
Samples |
Bending rigidity (N cm2 cm−1) |
Bending hysteresis moment (N cm cm−1) |
Warp |
Weft |
Warp |
Weft |
Coated cotton fabric |
0.271 |
0.209 |
0.194 |
0.267 |
Cotton fabric |
0.022 |
0.024 |
0.021 |
0.023 |
4. Conclusion
Anthraquinone dye moiety was successfully introduced into blocked waterborne polyurethane which was synthesized by IPDI, PEG400, MDEA and MEKO. Mn and Mw of the dye-bonded BWPU are 2303 g mol−1 and 2434 g mol−1, respectively, with a polydispersity of 1.06. The maximum absorption wavelengths of the dye-bonded BWPUs in both water and ethanol exhibit at 253 nm, 595 nm and 642 nm, which are similar to those of corresponding monomolecular dye. The absorbance ratio of the dye-bonded BWPU with more hydrophilic chain extender reaches above 90%, providing better centrifugal stability and water solubility than the dye-mixed BWPU. The dye-bonded BWPU undertakes de-blocking at about 150 °C and exhibits good thermal stability below 100 °C. The incorporation of anthraquinone chromophores into polyurethane chain and temporarily blocking the terminal NCO groups can effectively enhance the color fastness, especially the washing fastness. The recovery angle of the cotton coated by the dye-bonded BWPU can be up to 120°, achieving simultaneous coloring and wrinkle-resistant finishing effects. And the linear polyurethanes are uniformly distributed on the cellulosic fibers with one or both ends of polyurethane micro fibrils covalently bonded with –OH groups of cellulosic fibers. The handle of the treated cotton becomes poor, a phenomenon often associated with improved wrinkle resistance. Better balance between fabric handle and wrinkle resistance may be accomplished by improving the chain flexibility of dye-bonded BWPU in a future study.
Acknowledgements
The authors are grateful for the financial support of the National Natural Science Foundation of China (21174055), and the Graduate Students Innovation Project of Jiangsu Province in China (CXZZ13_0753).
References
- R. Chen, C. Zhang and M. R. Kessler, RSC Adv., 2014, 4(67), 35476 RSC.
- V. García-Pacios, J. A. Jofre-Reche, V. Costa, M. Colera and J. M. Martín-Martínez, Prog. Org. Coat., 2013, 76(10), 1484–1493 CrossRef PubMed.
- H. Sardon, L. Irusta, A. González and M. J. Fernández-Berridi, Prog. Org. Coat., 2013, 76(9), 1230–1235 CrossRef CAS PubMed.
- H. Sardon, L. Irusta, M. J. Fernández-Berridi, M. Lansalot and E. Bourgeat-Lami, Polymer, 2010, 51(22), 5051–5057 CrossRef CAS PubMed.
- Y. Yu, B. Liao, G. Li, S. Jiang and F. Sun, Ind. Eng. Chem. Res., 2014, 53(2), 564–571 CrossRef CAS.
- Q. B. Meng, S.-I. Lee, C. Nah and Y.-S. Lee, Prog. Org. Coat., 2009, 66(4), 382–386 CrossRef CAS PubMed.
- B. Li, J. Shen, R. Liang, W. Ji and C. Kan, Colloid Polym. Sci., 2012, 290(18), 1893–1900 CAS.
- A. Altomare, F. Ciardelli and R. Solaro, Macromol. Symp., 2004, 218, 353–362 CAS.
- A. Altomare, F. Ciardelli, G. Faralli and R. Solaro, Macromol. Mater. Eng., 2003, 288(9), 679–692 CrossRef CAS.
- H. H. Wang and C. T. Gen, J. Appl. Polym. Sci., 2002, 84(4), 797–805 CrossRef CAS.
- M. Joshi, S. Jauhari and K. R. Desai, Res. Chem. Intermed., 2012, 38(9), 2137–2148 CrossRef CAS.
- E. C. Buruiana and T. Buruiana, Eur. Polym. J., 2001, 37(12), 2505–2511 CrossRef CAS.
- E. C. Buruiana, T. Buruiana, G. Strat and M. Strat, J. Photochem. Photobiol., A, 2004, 162(1), 23–31 CrossRef CAS.
- S. Mallakpour, F. Rafiemanzelat and K. Faghihi, Dyes Pigm., 2007, 74(3), 713–722 CrossRef CAS PubMed.
- F. Ciardelli, G. Ruggeri and A. Pucci, Chem. Soc. Rev., 2013, 42(3), 857–870 RSC.
- C. W. Chang, G. S. Liou and S. H. Hsiao, J. Mater. Chem., 2007, 17(10), 1007–1015 RSC.
- L. Tang, B. Tang and S. Zhang, Chin. J. Chem. Eng., 2011, 19(4), 661–665 CrossRef CAS.
- X. X. Wang, S. F. Zhang, T. B. Tao and Y. J. Zong, Proceeding of the 3rd international conference on functional molecules, 2005, pp. 8–11 Search PubMed.
- J. Su, X. Zhang, J. Dai and J. Zhang, J. Dispersion Sci. Technol., 2012, 33(9), 1373–1378 CrossRef CAS.
- M. Kabir Yakubu, Adv. Chem. Eng. Sci., 2012, 02(02), 300–308 CrossRef.
- S. Subramani, I. W. Cheong and J. H. Kim, Eur. Polym. J., 2004, 40(12), 2745–2755 CrossRef CAS PubMed.
- S. Subramani, I. W. Cheong and J. H. Kim, Prog. Org. Coat., 2004, 51(4), 328–338 CrossRef PubMed.
- S. Subramani, Y.-J. Park, Y.-S. Lee and J.-H. Kim, Prog. Org. Coat., 2003, 48(1), 71–79 CrossRef CAS.
- E. Delebecq, J. P. Pascault, B. Boutevin and F. Ganachaud, Chem. Rev., 2013, 113(1), 80–118 CrossRef CAS PubMed.
- H.-H. Wang and Y.-T. Lin, J. Appl. Polym. Sci., 2003, 90(8), 2045–2052 CrossRef CAS.
- X. H. Hu, X. Y. Zhang, J. Liu and J. B. Dai, J. Lumin., 2013, 142, 23–27 CrossRef CAS PubMed.
- Z. S. Petrovic, Z. Zavargo, J. H. Flynn and W. J. Macknight, J. Appl. Polym. Sci., 1994, 51, 1087–1095 CrossRef CAS.
- Z. Chang, M. Zhang, A. G. Hudson, E. B. Orler, R. B. Moore, G. L. Wilkes and S. R. Turner, Polymer, 2013, 54(26), 6910–6917 CrossRef CAS PubMed.
- S. S. Umare and A. S. Chandure, Chem. Eng. J., 2008, 142(1), 65–77 CrossRef CAS PubMed.
- L. Lei, L. Zhong, X. Lin, Y. Li and Z. Xia, Chem. Eng. J., 2014, 253, 518–525 CrossRef CAS PubMed.
- M. Raheel and C. Guo, Text. Res. J., 1998, 68(8), 571–577 CrossRef CAS PubMed.
- H. M. Choi, Text. Res. J., 2002, 72(6), 469–473 CrossRef CAS PubMed.
- H. M. Choi, M. Sriniasan and N. M. Morris, J. Appl. Polym. Sci., 1994, 54, 2107–2118 CrossRef CAS.
- Y. Li, H. Liu, J. Song, O. J. Rojas and J. P. Hinestroza, ACS Appl. Mater. Interfaces, 2011, 3(7), 2349–2357 CAS.
|
This journal is © The Royal Society of Chemistry 2015 |