DOI:
10.1039/C4RA16399K
(Paper)
RSC Adv., 2015,
5, 21012-21018
Synthesis, optical, electrochemical and photovoltaic properties of a D–π–A fluorescent dye with triazine ring as electron-withdrawing anchoring group for dye-sensitized solar cells
Received
15th December 2014
, Accepted 16th February 2015
First published on 17th February 2015
Abstract
The D–π–A fluorescent dye OUJ-1 with 1,3,5-triazine ring as electron-withdrawing anchoring group and (diphenylamino)carbazole containing a thiophene ring as D–π moiety have been newly developed and their optical and electrochemical properties, adsorption states on TiO2 nanoparticles, and photovoltaic performance in dye-sensitized solar cell (DSSC) were investigated. The absorption maximum (λabsmax) for the intramolecular charge-transfer (ICT) absorption band of the D–π–A dye OUJ-1 occurs at a longer wavelength than those of D–π–A dye NI-6 with a pyridyl group and D–π–A dye OUK-1 with a pyrzayl group. Moreover, the dye OUJ-1 exhibits significant fluorescence solvatochromic properties, that is, a bathochromic shift of the fluorescence band and a decrease in the fluorescence quantum yield (Φ) due to a change from the 1ICT excited state to the twisted intramolecular charge transfer (TICT) excited state with increasing solvent polarity were observed. The photovoltaic performance of a DSSC based on OUJ-1 is lower than those of NI-6 and OUK-1 due to the low dye loading of OUJ-1 on TiO2 electrode. It was found that the dye OUJ-1 is adsorbed onto the TiO2 surface through the formation of trizainium ions at the Brønsted acid sites (surface-bound hydroxyl groups, Ti–OH) on the TiO2 surface, although the dye NI-6 was predominantly adsorbed on the TiO2 through coordinate bonding between the pyridyl group of the dye and the Lewis acid sites (exposed Tin+ cations) on the TiO2 surface, and the dye OUK-1 was adsorbed on the TiO2 surface through both the formations of hydrogen bonding of pyrazyl groups and pyrazinium ions at Brønsted acid sites on the TiO2 surface. This work revealed that the binding mode of D–π–A dye sensitizers with azine rings on the TiO2 surface can be changed by control of the basicity and electron density of the azine rings.
Introduction
Donor–acceptor π-conjugated (D–π–A) fluorescent dyes having both electron-donating (D) and -accepting (A) groups linked by π-conjugated bridges are useful as emitters for organic light emitting diodes (OLEDs),1–3 photosensitizers for dye-sensitized solar cells (DSSCs),4–6 and fluorescence sensors for a variety of applications,7–9 because of their strong absorption and emission properties originating from the intramolecular charge transfer (ICT) excitation from the donor to acceptor moiety in the D–π–A structures. As a noteworthy structural feature of D–π–A dyes, the highest occupied molecular orbital (HOMO) is localized over the π-conjugated system close to the donor part, and the lowest unoccupied molecular orbital (LUMO) is localized over the acceptor part. In the light of the D–π–A structure concept, the expansion of π conjugation and the increase in the electron-donating and electron-accepting abilities of donors (D) and acceptors (A), respectively, leads to the decrease in the energy gap between the HOMO and LUMO. Thus, the photophysical, electrochemical, and ICT properties of a D–π–A dye strongly depend on the electron-donating ability of D and the electron-accepting ability of A, as well as on the electronic characteristics of the π bridge, that is, they should be strategically tunable through chemical modification on each component (D, A, or π bridge) to suit the requirements for efficient optoelectronic devices and optical, biochemical, and medicinal sensors.
In this work, D–π–A fluorescent dye OUJ-1 with 1,3,5-triazine ring as electron-withdrawing anchoring group and (diphenylamino)carbazole containing a thiophene ring as D–π moiety have been newly developed and their optical and electrochemical properties, adsorption states on TiO2 nanoparticles, and photovoltaic performances in DSSC were investigated (Scheme 1). The absorption maximum (λabsmax) for the ICT absorption band of the D–π–A dye OUJ-1 occurs at a longer wavelength than those of D–π–A dye NI-6 with a pyridyl group10 and D–π–A dye OUK-1 with a pyrzayl group.11 Moreover, the dye OUJ-1 exhibits significant fluorescence solvatochromic properties. It was found that the dye OUJ-1 is adsorbed onto the TiO2 surface through the formation of trizainium ion at the Brønsted acid sites (surface-bound hydroxyl groups, Ti–OH) on TiO2 surface, although the dye NI-6 was predominantly adsorbed on the TiO2 through coordinate bonding between the pyridyl group of the dye and the Lewis acid site (exposed Tin+ cations) on the TiO2 surface, and the dye OUK-1 was adsorbed on the TiO2 surface through both the formations of hydrogen bonding of pyrazyl group and pyrazinium ion at Brønsted acid sites on the TiO2 surface. The photovoltaic performance of DSSC based on OUJ-1 is lower those of NI-6 and OUK-1 due to low dye loading of OUJ-1 on TiO2 electrode. On the basis of the experimental results and molecular orbital calculations, the differences of optical and electrochemical properties, adsorption states on TiO2 nanoparticles, and photovoltaic performances in DSSC among these D–π–A dyes with azine rings are discussed.
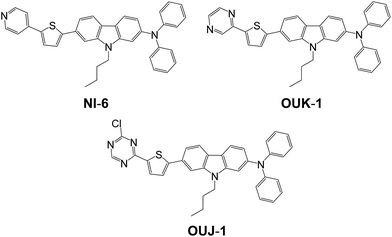 |
| Scheme 1 Chemical structures of D–π–A dye sensitizers NI-6, OUK-1 and OUJ-1 with azine rings. | |
Results and discussion
Synthesis
The synthesis of NI-6 and OUK-1 have been reported elsewhere.10a,11 The synthetic pathway of D–π–A fluorescent dye OUJ-1 is shown in Scheme 2. The dye OUJ-1 was prepared by Stille coupling of stannyl compound 1 (ref. 11) with 2,4-dichloro-1,3,5-triazine.
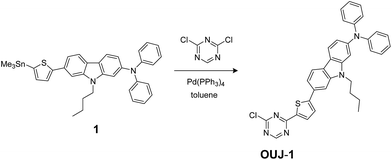 |
| Scheme 2 Synthetic pathway of D–π–A fluorescent dye OUJ-1 with 1,3,5-triazine ring. | |
Optical properties
The absorption and fluorescence spectra of NI-6, OUK-1, OUJ-1 in various solvents are shown in Fig. 1 and their spectral data are summarized in Table 1. The three dyes in 1,4-dioxane show the absorption maximum (λabsmax) at around 395–430 nm, which is assigned to the ICT excitation from electron donor moiety (diphenylamino group) to electron acceptor moiety (pyridyl, pyrazyl or trizayl group) (Fig. 1a). The λabsmax for ICT band of the D–π–A dye OUJ-1 with 2-chloro-1,3,5-triazyl group occurs at a longer wavelength by 35 nm and 29 nm, respectively, than those of D–π–A dye NI-6 with a pyridyl group and D–π–A dye OUK-1 with a pyrzayl group, because of stronger electron-withdrawing ability of 2-chloro-1,3,5-triazyl group relative to pyridyl and pyrazyl groups. The molar extinction coefficient (ε) for the ICT band is 49
600 M−1 cm−1 for NI-6, 45
400 M−1 cm−1 for OUK-1 and 36
500 M−1 cm−1 for OUJ-1, respectively. The corresponding fluorescence maxima (λflmax) of OUJ-1 also occurs at a longer wavelength than those of NI-6 and OUK-1. Interestingly, the dye OUJ-1 exhibits significant fluorescence solvatochromic properties compared with NI-6 and OUK-1, that is, bathochromic shift of fluorescence band and a decrease in the fluorescence quantum yields (Φ) with increasing solvent polarity were observed, although the absorption spectra are nearly independent of solvent polarity (Fig. 1b–d). In low polar solvent such as 1,4-dioxane and THF, the dye OUJ-1 exhibits fluorescence band at 547 nm in 1,4-dioxane and 600 nm in THF, respectively, arising from the 1ICT excited state. In polar solvent such as acetone, on the other hand, a new fluorescence band with the a significant decrease in the Φ value appeared at 638 nm, arising from the twisted intramolecular charge transfer (TICT) excited state which is due to the twisting between the triazyl group and the (diphenylamino)carbazole moiety, leading to non-radiative deactivation. Moreover, in highly polar solvent such as DMSO, owing to significant non-radiative deactivation from the TICT excited state leading to a decrease in the Φ value, the fluorescence band from only the 1ICT excited state was observed at 516 nm. Consequently, this result suggested that the predominant excited state for the D–π–A fluorescent dye OUJ-1 with triazyl group, which possesses stronger electron-withdrawing ability than those of pyridyl and pyrazyl groups, changes from the 1ICT state to the TICT state with increasing solvent polarity, resulting in a significant fluorescence solvatochromism.12,13
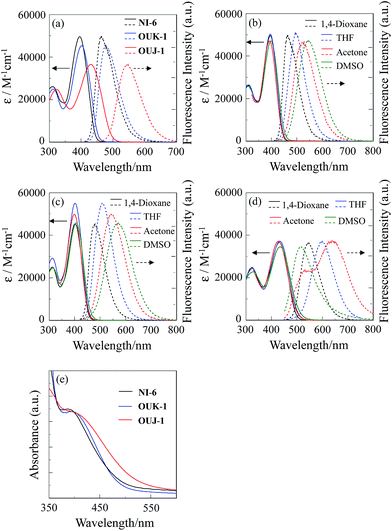 |
| Fig. 1 (a) Absorption ( ) and fluorescence (⋯) spectra of NI-6, OUK-1 and OUJ-1 in 1,4-dioxane. Absorption ( ) and fluorescence (⋯) spectra of (b) NI-6, (c) OUK-1 and (d) OUJ-1 in 1,4-dioxane, THF, acetone and DMSO. (e) Absorption spectra of NI-6, OUK-1 and OUJ-1 adsorbed on TiO2 film. | |
Table 1 Optical data of NI-6, OUK-1 and OUJ-1
Dye |
Solvent |
λ
absmax
a/nm (ε M−1 cm−1) |
λ
flmax
a/nm (Φf) |
Stokes shift cm−1 |
Fluorescence quantum yields (Φf) were determined by using a calibrated integrating sphere system (the observed λabsmax in each solvent was used as the excitation wavelengths).
|
NI-6
|
1,4-Dioxane |
396 (49 600) |
464 (0.58) |
3700 |
THF |
395 (49 900) |
495 (0.77) |
5114 |
Acetone |
393 (48 000) |
528 (0.58) |
6505 |
DMSO |
399 (47 100) |
547 (0.68) |
6781 |
OUK-1
|
1,4-Dioxane |
402 (45 400) |
478 (0.46) |
3955 |
THF |
402 (55 000) |
508 (0.68) |
5190 |
Acetone |
399 (49 800) |
542 (0.56) |
6612 |
DMSO |
406 (45 800) |
572 (0.55) |
7148 |
OUJ-1
|
1,4-Dioxane |
431 (36 500) |
547 (0.57) |
5780 |
THF |
433 (37 200) |
600 (0.63) |
6428 |
Acetone |
428 (37 200) |
638 (0.06) |
7690 |
DMSO |
434 (34 500) |
516 (<0.02) |
3661 |
The absorption spectra of NI-6, OUK-1 and OUJ-1 adsorbed on TiO2 film are shown in Fig. 1e. The absorption band of OUJ-1 are broadened compared with those of NI-6 and OUK-1. However, the absorption peak wavelengths of the three dyes adsorbed on TiO2 are similar to those in 1,4-dioxane, although the absorption bands of the three dyes adsorbed on the TiO2 film are broadened compared with those in 1,4-dioxane. Thus, this result indicates that the three dyes form weak π-stacked aggregates on TiO2 surface.
Electrochemical properties
The electrochemical properties of NI-6, OUK-1 and OUJ-1 and were determined by cyclic voltammetry (CV). The CV curve of the three dyes are shown in Fig. 2. The reversible oxidation waves for the three dyes were observed at 0.37 V for NI-6, 0.42 V for OUK-1, 0.45 V for OUJ-1, respectively, vs. ferrocene/ferrocenium (Fc/Fc+) (Table 2). The corresponding reduction waves appeared at 0.30 V for NI-6, 0.35 V for OUK-1 and 0.37 V for OUJ-1, respectively, thus showing that the oxidized states of the three dyes are stable. The HOMO energy level vs. the normal hydrogen electrode (NHE) was evaluated from the half-wave potential for oxidation (Eox1/2 = 0.34 V for NI-6, 0.39 V for OUK-1 and 0.41 V for OUJ-1). The HOMO energy level was 0.97 V for NI-6, 1.11 V for OUK-1 and 1.13 V for OUJ-1, respectively, vs. NHE, thus indicating that the three dyes have comparable HOMO energy levels. This result shows that the HOMO energy levels are more positive than the I3−/I− redox potential (0.4 V), and thus this ensures an efficient regeneration of the oxidized dyes by electron transfer from the I3−/I− redox couple in the electrolyte. The LUMO energy level was estimated from the Eox1/2 and an intersection of absorption and fluorescence spectra (436 nm; 2.84 eV for NI-6, 445 nm; 2.79 eV for OUK-1, 491 nm; 2.52 eV for OUJ-1) in 1,4-dioxane, that is, the LUMO energy level was obtained through eqn – [E0–0 − HOMO], where E0–0 transition energy is an intersection of absorption and fluorescence spectra corresponding to the energy gap between HOMO and LUMO, and HOMO vs. NHE was evaluated from the Eox1/2 value. The LUMO energy levels decrease in the order of NI-6 (−1.87 V) > OUK-1 (−1.68 V) > OUJ-1 (−1.40 V), showing that the lowering of LUMO energy levels is dependent on the electron-withdrawing ability of azine ring. Consequently, it was revealed that the red-shift of the ICT absorption band for OUJ-1 relative to NI-6 and OUK-1 is attributed to stabilization of the LUMO level because of stronger electron-withdrawing ability of triazyl group relative to pyridyl and pyrazyl groups, resulting in a decrease in the HOMO–LUMO band gap. Evidently, the LUMO energy levels of the three dyes are higher than the energy level (Ecb) of the CB of TiO2 (−0.5 V), suggesting that an electron injection to the CB of TiO2 is thermodynamically feasible.
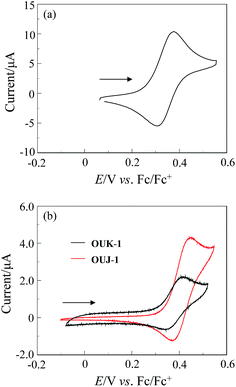 |
| Fig. 2 Cyclic voltammograms of (a) NI-6 in CH2Cl2 containing 0.1 M Bu4NClO4 and (b) OUK-1 and OUJ-1 in DMF containing 0.1 M Bu4NClO4. The arrow denotes the direction of the potential scan. | |
Table 2 Electrochemical data and HOMO and LUMO energy levels of NI-6, OUK-1 and OUJ-1
Dye |
E
pa/Va |
E
pc/Va |
E
ox1/2/Va |
HOMO/Vb |
LUMO/Vb |
Anodic (Epa) and cathodic (Epc) peak potentials, and half-wave potentials for oxidation (Eox1/2) vs. Fc/Fc+ were recorded in CH2Cl2/Bu4NClO4 (0.1 M) solution for NI-6 and DMF/Bu4NClO4 (0.1 M) solution for OUK-1 and OUJ-1, respectively.
vs. normal hydrogen electrode (NHE).
|
NI-6
|
0.37 |
0.30 |
0.34 |
0.97 |
−1.87 |
OUK-1
|
0.42 |
0.35 |
0.39 |
1.11 |
−1.68 |
OUJ-1
|
0.45 |
0.37 |
0.41 |
1.13 |
−1.40 |
Theoretical calculations
In order to examine the electronic structures of NI-6, OUK-1 and OUJ-1, the molecular orbitals of the three dyes were calculated using density functional theory (DFT) at the B3LYP/6-31G(d, p) level. The DFT calculations indicate that for the three dyes the HOMOs were mostly localized on the (diphenylamino)carbazole containing a thiophene ring, and the LUMOs were mostly localized on the thienylpyridine for NI-6, thienylpyrazine for OUK-1 and thienyltriazine for OUJ-1, respectively (Fig. 3). Accordingly, the DFT calculations reveal that dye excitations upon light irradiation induce a strong ICT from the (diphenylamino)carbazole to the azine ring.
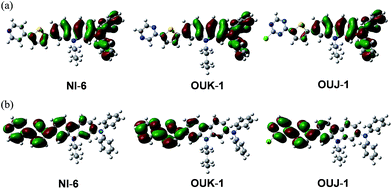 |
| Fig. 3 (a) HOMO and (b) LUMO of NI-6, OUK-1 and OUJ-1 by the density functional theory (DFT) calculations at B3LYP/6-31G(d, p) level. | |
FTIR spectra
To elucidate the adsorption states of OUJ-1 on TiO2 nanoparticles, we measured the FTIR spectra of the dye powders and the dyes adsorbed on TiO2 nanoparticles (Fig. 4). In our previous study, it was found that the dye NI-6 was predominantly adsorbed on the TiO2 through coordinate bonding between the pyridyl group of the dye and the Lewis acid site (exposed Tin+ cations) on the TiO2 surface, and the dye OUK-1 was adsorbed on the TiO2 through both the formations of hydrogen bonding of pyrazyl group and pyrazinium ion at Brønsted acid sites (surface-bound hydroxyl groups, Ti–OH) on the TiO2 surface, where the adsorption amount of the dye adsorbed on TiO2 electrode is 3.1 × 1016 and 3.0 × 1016 molecules per cm2 for NI-6 and OUK-1, respectively.10a,11 For the powders of OUJ-1, the C
N stretching band of trizaine ring was clearly observed at 1536 cm−1. When the dye OUJ-1 was adsorbed on the TiO2 surface, where the adsorption amount of the dye adsorbed on TiO2 electrode is 2.5 × 1016 molecules per cm2, the band at 1536 cm−1 disappeared completely and a new band appeared at around 1650 cm−1, indicating that the dye OUJ-1 is adsorbed onto the TiO2 through the formation of trizainium ion at the Brønsted acid sites on TiO2 surface. These results suggest that the binding modes of D–π–A dye sensitizers with azine rings on TiO2 surface can be changed by control of the basicity and electron density of azine rings.
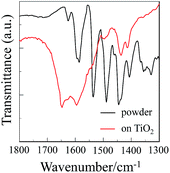 |
| Fig. 4 FTIR spectra of the dye powders and the dyes (2.5 × 1016 molecules per cm2) adsorbed on TiO2 nanoparticles for OUJ-1. | |
Dye-sensitized solar cells
The DSSCs were fabricated by using the dye-adsorbed TiO2 electrode (9 μm), Pt-coated glass as a counter electrode, and an acetonitrile solution with iodine (0.05 M), lithium iodide (0.1 M), and 1,2-dimethyl-3-propylimidazolium iodide (0.6 M) as an electrolyte. The photocurrent–voltage (I–V) characteristics were measured under simulated solar light (AM 1.5, 100 mW cm−2). The incident photon-to-current conversion efficiency (IPCE) spectra and the I–V curves are shown in Fig. 5. The photovoltaic performance parameters are collected in Table 3. The adsorption amount of dyes adsorbed on TiO2 is 3.0 × 1016 and 2.5 × 1017 molecules per cm2 for OUK-1 and OUJ-1, respectively, when the TiO2 electrode was immersed into 1.0 mM dye solution of OUK-1 or OUJ-1. It is worth mentioning here that the adsorption amounts of NI-6 on the co-adsorbed TiO2 electrode is 3.1 × 1016 molecules cm−2, which is equivalent to that of OUK-1 and OUJ-1 under the adsorption condition of 0.1 mM dye solution, when the TiO2 electrode was immersed into dye NI-6 solution as low as 0.06 mM. Moreover, the adsorption amount of NI-6 reached as high as 6.9 × 1016 molecules cm−2 under the adsorption condition of 0.1 mM dye solution. The difference in adsorption ability onto TiO2 surface among the three dyes may be associated with the basicity and electron density of azine rings, leading to changes of the binding modes of azine rings on TiO2 surface. Consequently, this result indicates that the pyridyl unit is better anchoring group for achieving high dye loading than pyrazyl and triazyl group. When the adsorption amount of the dye is 2.5–3.1 × 1016 molecules per cm2, the maximum IPCE value at around at 420 nm increases in the order of OUJ-1 (18%) < NI-6 (36%) ≈ OUK-1 (38%) (Fig. 5a). The short-circuit photocurrent density (Jsc) and solar energy-to-electricity conversion yield (η) also increase in the order of OUJ-1 (2.00 mA cm−2, 0.54%) < NI-6 (2.96 mA cm−2, 0.79%) ≈ OUK-1 (2.99 mA cm−2, 0.89%) (Fig. 5b and Table 2). The lower photovoltaic performances for DSSC based on OUJ-1 are attributed to low adsorption amount of the dyes on TiO2 electrode. On the other hand, the DSSC based on NI-6-adsorbed TiO2 electrode with 6.9 × 1016 molecules per cm2 showed the Jsc (4.76 mA cm−2), η values (1.47%) and maximum IPCE value (64% at 420 nm), which are twice as high as those of low adsorption amount of the dye (3.1 × 1016 molecules cm−2) due to the enhancement of LHE with increasing dye loading on TiO2 electrode. Moreover, the open-circuit photovoltage (Voc) for DSSCs based on OUK-1 (448 mV) and OUJ-1 (444 mV) is lower than that of NI-6 (460 mV and 523 mV for 3.1 × 1016 and 6.9 × 1016 molecules per cm2, respectively). Thus, electrochemical impedance spectroscopy (EIS) analysis was performed to study the electron recombination process in DSSCs in the dark under a forward bias of −0.45 V or −0.60 V with a frequency range of 10 mHz to 100 kHz. The large semicircle in the Nyquist plot (Fig. 6a), which corresponds to the midfrequency peaks in the Bode phase plots, represents the charge recombination between the injected electrons in TiO2 and I3− ions in the electrolyte, that is, the charge-transfer resistances at the TiO2/dye/electrolyte interface. The Nyquist plots show that the resistance value of the large semicircle for NI-6 (44 Ω and 50 Ω for 3.1 × 1016 and 6.9 × 1016 molecules per cm2, respectively) is larger than those of OUK-1 (26 Ω) and OUJ-1 (20 Ω), indicating that the electron recombination resistance of NI-6 is higher than those of those of OUK-1 and OUJ-1. The electron recombination lifetimes (τe) expressing the electron recombination between the injected electrons in TiO2 and I3− ions in the electrolyte, extracted from the angular frequency (ωrec) at the midfrequency peak in the Bode phase plot (Fig. 6b) using τe = 1/ωrec, are 16 ms for both DSSCs based on NI-6-adsorbed TiO2 electrode with 3.1 × 1016 and 6.9 × 1016 molecules per cm2, which is larger than 10 ms and 8 ms for DSSCs based on OUK-1 and OUJ-1, respectively. Thus, this result revealed that the charge recombination between the injected electrons in TiO2 and I3− ions in the electrolyte is not major reason for the difference in Voc value between the three dyes, but the negative shift of the Ecb of TiO2 by the formations of coordinate bonding between the pyridyl group of the dye and the Lewis acid site on the TiO2 surface may result in a higher Voc value for NI-6.6
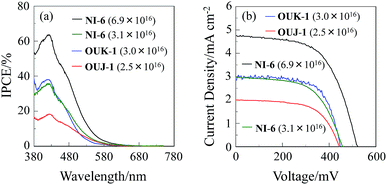 |
| Fig. 5 (a) IPCE spectra and (b) I–V curves and of DSSCs based on NI-6, OUK-1 and OUJ-1. The adsorption amount of the dye adsorbed on TiO2 electrode is 3.1 × 1016 and 6.9 × 1016 molecules per cm2 for NI-6, and 3.0 × 1016 and 2.5 × 1016 molecules per cm2 for OUK-1 and OUJ-1, respectively. | |
Table 3 DSSC performance parameters of NI-6, OUK-1 and OUJ-1
Dye |
Moleculesa cm−2 |
J
sc
e/mA cm−2 |
V
oc
e/mV |
FFe |
η
e (%) |
The 9 μm thick TiO2 electrode was immersed into dye solution in THF.
Under the adsorption condition of 0.1 mM dye solution in THF.
Under the adsorption condition of 0.06 mM dye solution in THF.
Under the adsorption condition of 1.0 mM dye solution in THF.
Under a simulated solar light (AM 1.5, 100 mW cm−2).
|
NI-6
|
6.9 × 1016b |
4.76 |
523 |
0.59 |
1.47 |
3.1 × 1016c |
2.96 |
460 |
0.58 |
0.79 |
OUK-1
|
3.0 × 1016d |
2.99 |
448 |
0.67 |
0.89 |
OUJ-1
|
2.5 × 1016d |
2.00 |
444 |
0.61 |
0.54 |
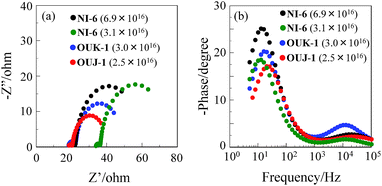 |
| Fig. 6 (a) Nyquist plots and (b) Bode phase plots of DSSCs based on NI-6, OUK-1 and OUJ-1. The adsorption amount of the dye adsorbed on TiO2 electrode is 3.1 × 1016 and 6.9 × 1016 molecules per cm2 for NI-6, and 3.0 × 1016 and 2.5 × 1016 molecules per cm2 for OUK-1 and OUJ-1, respectively. | |
Conclusions
We have designed and synthesized D–π–A fluorescent dye OUJ-1 with 1,3,5-triazine ring as electron-withdrawing anchoring group and (diphenylamino)carbazole containing a thiophene ring as D–π moiety. The dye OUJ-1 exhibits significant fluorescence solvatochromic properties due to change from the 1ICT excited state to the TICT excited state with increasing solvent polarity, compared with D–π–A dye NI-6 with a pyridyl group and D–π–A dye OUK-1 with a pyrzayl group. It was found the dye OUJ-1 is adsorbed onto the TiO2 surface through the formation of trizainium ion at the Brønsted acid sites on TiO2 surface, although the dye NI-6 was predominantly adsorbed on the TiO2 through coordinate bonding between the pyridyl group of the dye and the Lewis acid site on the TiO2 surface, and the dye OUK-1 was adsorbed on the TiO2 surface through both the formations of hydrogen bonding of pyrazyl group and pyrazinium ion at Brønsted acid sites on the TiO2 surface. The photovoltaic performance of DSSC based on OUJ-1 is lower those of NI-6 and OUK-1 due to low dye loading of OUJ-1 on TiO2 electrode. In addition, our results indicate that the pyridyl unit is better anchoring group for achieving high dye loading than pyrazyl and triazyl group. Consequently, this work suggests that the binding modes of D–π–A dye sensitizers with azine rings on TiO2 surface can be changed by control of the basicity and electron density of azine rings. Further studies to ensure this conclusion are now in progress by estimating pKa values of D–π–A dye sensitizers with azine rings.
Experimental
General
Melting points were measured with a Yanaco micro melting point apparatus MP model. IR spectra were recorded on a Perkin Elmer Spectrum One FT-IR spectrometer by ATR method. High-resolution mass spectral data were acquired on a Thermo Fisher Scientific LTQ Orbitrap XL. 1H NMR spectra were recorded on a Varian-400 (400 MHz) FT NMR spectrometer. Absorption spectra were observed with a Shimadzu UV-3150 spectrophotometer and fluorescence spectra were measured with a HORIBA FluoroMax-4 spectrofluorometer. The fluorescence quantum yields in solution were determined by a HORIBA FluoroMax-4 spectrofluorometer by using a calibrated integrating sphere system. Cyclic voltammetry (CV) curves were recorded in CH2Cl2/Bu4NClO4 (0.1 M) solution for NI-6 and DMF/Bu4NClO4 (0.1 M) solution for OUJ-1 and OUJ-1, respectively, with a three-electrode system consisting of Ag/Ag+ as reference electrode, Pt plate as working electrode, and Pt wire as counter electrode by using a AMETEK Versa STAT 4 potentiostat. The highest occupied molecular orbital (HOMO) and lowest unoccupied molecular orbital (LUMO) energy levels of NI-6, OUK-1 and OUJ-1 were evaluated from the spectral analyses and the CV data (the HOMO energy level was evaluated from the Eox1/2). The LUMO energy level was estimated from the Eox1/2 and an intersection of absorption and fluorescence spectra (436 nm; 2.84 eV for NI-6, 445 nm; 2.79 eV for OUK-1, 491 nm; 2.52 eV for OUJ-1) in 1,4-dioxane, that is, the LUMO energy level was obtained through eqn – [E0–0 − HOMO], where E0–0 transition energy is an intersection of absorption and fluorescence spectra corresponding to the energy gap between HOMO and LUMO, and HOMO vs. NHE was evaluated from the Eox1/2 value. Electrochemical impedance spectroscopy (EIS) for DSSCs in the dark under a forward bias of −0.60 V for NI-6 and OUK-1 and −0.45 V for OUJ-1 with a frequency range of 10 mHz to 100 kHz was measured with a AMETEK Versa STAT 3.
Synthesis
9-Butyl-7-(5-(4-chloro-1,3,5-triazin-2-yl)thiophen-2-yl)-N,N-diphenyl-9H-carbazol-2-amine (OUJ-1).
A solution of 1 (ref. 11) (0.34 g, 0.53 mmol), 2,4-dichloro-1,3,5-triazine (0.09 g, 0.58 mmol), and Pd(PPh3)4 (0.018 g, 0.016 mmol) in toluene (15 mL) was stirred for 3 h at 110 °C under an argon atmosphere. After concentrating under reduced pressure, the resulting residue was dissolved in dichloromethane and washed with water. The dichloromethane extract were evaporated under reduced pressure. The residue was chromatographed on silica gel (ethyl acetate–hexane = 1
:
6 as eluent as eluent) to give OUJ-1 (0.13 g, yield 42%) as a yellow solid; m.p. 201–202 °C; IR (ATR):
= 1586, 1536, 1488, 1444 cm−1; 1H NMR (400 MHz, CD2Cl2) δ = 0.88 (t, J = 7.4 Hz, 3H), 1.26–1.33 (m, 2H), 1.73–1.80 (m, 2H), 4.19 (t, J = 7.0 Hz, 2H), 6.95 (dd, J = 1.6 and 8.4 Hz, 1H), 7.02–7.06 (m, 2H), 7.12–7.16 (m, 5H), 7.25–7.30 (m, 4H), 7.56 (d, J = 4.0 Hz, 1H), 7.58 (dd, J = 1.6 and 8.1 Hz, 1H), 7.69 (d, J = 1.2 Hz, 1H), 7.94 (d, J = 8.4 Hz, 1H), 8.03 (d, J = 8.1 Hz, 1H), 8.24 (d, J = 4.0 Hz, 1H), 8.89 (s, 1H); HRMS (APCI): m/z (%): [M + H+] calcd for C35H29N5ClS, 586.18267; found 586.18292.
Preparation of DSSCs
The TiO2 paste (JGC Catalysts and Chemicals Ltd., PST-18NR) was deposited on a fluorine-doped-tin-oxide (FTO) substrate by doctor-blading, and sintered for 50 min at 450 °C. The 9 μm thick TiO2 electrode was immersed into 0.06 mM or 0.1 mM dye (NI-6) solution in THF or 1.0 mM dye (OUK-1 and OUJ-1) solution in THF for 15 hours enough to adsorb the dye sensitizers. The DSSCs were fabricated by using the TiO2 electrode (0.5 × 0.5 cm2 in photoactive area) thus prepared, Pt-coated glass as a counter electrode, and a solution of 0.05 M iodine, 0.1 M lithium iodide, and 0.6 M 1,2-dimethyl-3-propylimidazolium iodide in acetonitrile as electrolyte. The photocurrent–voltage characteristics were measured using a potentiostat under a simulated solar light (AM 1.5, 100 mW cm−2). IPCE spectra were measured under monochromatic irradiation with a tungsten–halogen lamp and a monochromator. The dye-coated film for NI-6 and OUK-1 was immersed in a mixed solvent of THF–DMSO–NaOH aq. 1 M (5
:
4
:
1), which was used to determine the amount of dye molecules adsorbed onto the film by measuring the absorbance. The quantification of dye was made based on the molar extinction coefficient for λabsmax of dye in the above solution. For OUJ-1, the amount of adsorbed dye on TiO2 nanoparticles was determined form the calibration curve by absorption spectral measurement of the concentration change of the dye solution before and after adsorption, because the dye OUJ-1 is decomposed by the mixed solvent of THF–DMSO–NaOH aq. 1 M (5
:
4
:
1). Absorption spectra of the dyes adsorbed on TiO2 nanoparticles were recorded on the dyes-adsorbed TiO2 film in the transmission mode with a calibrated integrating sphere system.
Acknowledgements
This work was supported by The Iwatani Naoji Foundation's Research Grant.
Notes and references
-
(a) H.-C. Yeh, L.-H. Chan, W.-C. Wu and C.-T. Chen, J. Mater. Chem., 2004, 14, 1293 RSC;
(b) C.-T. Chen, Chem. Mater., 2004, 16, 4389 CrossRef CAS;
(c) G. Hughes and M. R. Bryce, J. Mater. Chem., 2005, 15, 94 RSC;
(d) S.-L. Lin, L.-H. Chan, R.-H. Lee, M.-Y. Yen, W.-J. Kuo, C.-T. Chen and R.-J. Jeng, Adv. Mater., 2008, 20, 3947 CrossRef CAS;
(e) G. Qian, Z. Zhong, M. Luo, D. Yu, Z. Zhang, Z. Y. Wang and D. Ma, Adv. Mater., 2009, 21, 111 CrossRef CAS.
- W. Li, L. Yao, H. Liu, Z. Wang, S. Zhang, R. Xiao, H. Zhang, P. Lu, B. Yang and Y. Ma, J. Mater. Chem. C, 2014, 2, 4733 RSC.
- F. Bureš, RSC Adv., 2014, 4, 58826 RSC.
-
(a) H. Choi, C. Baik, S. O. Kang, J. Ko, M.-S. Kang, M. K. Nazeeruddin and M. Grätzel, Angew. Chem., Int. Ed., 2008, 47, 327 CrossRef CAS PubMed;
(b) S. Kim, D. Kim, H. Choi, M.-S. Kang, K. Song, S. O. Kang and J. Ko, Chem. Commun., 2008, 4951 RSC;
(c) W.-H. Liu, I.-C. Wu, C.-H. Lai, C.-H. Lai, P.-T. Chou, Y.-T. Li, C.-L. Chen, Y.-Y. Hsu and Y. Chi, Chem. Commun., 2008, 5152 RSC.
-
(a) B. Liu, W. Zhu, Q. Zhang, W. Wu, M. Xu, Z. Ning, Y. Xie and H. Tian, Chem. Commun., 2009, 1766 RSC;
(b) G. Li, Y.-F. Zhou, X.-B. Cao, P. Bao, K.-J. Jiang, Y. Lin and L.-M. Yang, Chem. Commun., 2009, 2201 RSC;
(c) H. Choi, I. Raabe, D. Kim, F. Teocoli, C. Kim, K. Song, J.-H. Yum, J. Ko, Md. K. Nazeeruddin and M. Grätzel, Chem.–Eur. J., 2010, 16, 1193 CrossRef CAS PubMed.
-
(a) A. Mishra, M. K. R. Fischer and P. Bäuerle, Angew. Chem., Int. Ed., 2009, 48, 2474 CrossRef CAS PubMed;
(b) Y. Ooyama and Y. Harima, Eur. J. Org. Chem., 2009, 18, 2903 CrossRef;
(c) Z. Ning and H. Tian, Chem. Commun., 2009, 5483 RSC;
(d) Z. Ning, Y. Fu and H. Tian, Energy Environ. Sci., 2010, 3, 1170 RSC;
(e) Y. Ooyama and Y. Harima, ChemPhysChem, 2012, 13, 4032 CrossRef CAS PubMed.
-
(a) L. Xue, C. Liu and H. Jiang, Chem. Commun., 2009, 1061 RSC;
(b) W. Jiang and W. Wang, Chem. Commun., 2009, 3913 RSC;
(c) M. H. Kim, H. H. Jang, S. Yi, S.-K. Chang and M. S. Han, Chem. Commun., 2009, 4838 RSC;
(d) F. Qian, C. Zhang, Y. Zhang, W. He, X. Gao, P. Hu and Z. Guo, J. Am. Chem. Soc., 2009, 131, 1460 CrossRef CAS PubMed;
(e) R. Guliyev, A. Coskun and E. U. Akkaya, J. Am. Chem. Soc., 2009, 131, 9007 CrossRef CAS PubMed.
- Y. Hu, Z. Zhao, X. Bai, X. Yuan, X. Zhangaand and T. Masuda, RSC Adv., 2014, 4, 55179 RSC.
- Y. I. Park, O. Postupna, A. Zhugayevych, H. Shin, Y.-S. Park, B. Kim, H.-J. Yen, P. Cheruku, J. S. Martinez, J. W. Park, S. Tretiak and H.-L. Wang, Chem. Sci., 2015, 6, 789 RSC.
-
(a) Y. Ooyama, S. Inoue, T. Nagano, K. Kushimoto, J. Ohshita, I. Imae, K. Komaguchi and Y. Harima, Angew. Chem., Int. Ed., 2011, 50, 7429 CrossRef CAS PubMed;
(b) Y. Ooyama, T. Nagano, S. Inoue, I. Imae, K. Komaguchi, J. Ohshita and Y. Harima, Chem.–Eur. J., 2011, 17, 14837 CrossRef CAS PubMed;
(c) Y. Harima, T. Fujita, Y. Kano, I. Imae, K. Komaguchi, Y. Ooyama and J. Ohshita, J. Phys. Chem. C, 2013, 117, 16364 CrossRef CAS;
(d) N. Shibayama, H. Ozawa, M. Abe, Y. Ooyama and H. Arakawa, Chem. Commun., 2014, 50, 6398 RSC.
- Y. Ooyama, K. Uenaka, Y. Harima and J. Ohshita, RSC Adv, 2014, 4, 30225 RSC.
-
(a)
B. Valeur, Molecular Fluorescence, VCH, Weinheim, 2002 Search PubMed;
(b)
C. Reichardt, Solvents and Solvent Effects in Organic Chemistry, VCH, Weinheim, 2003 Search PubMed;
(c)
H. Zollinger, Color Chemistry, VCH, Weinheim, 2003 Search PubMed.
-
(a) S. Sumalekshmy and K. R. Gopidas, J. Phys. Chem. B, 2004, 108, 3705 CrossRef CAS;
(b) S. Sumalekshmy and K. R. Gopidas, New J. Chem., 2005, 29, 325 RSC;
(c) S. Sumalekshmy and K. R. Gopidas, Photochem. Photobiol. Sci., 2005, 4, 539 RSC;
(d) Y. Ooyama, G. Ito, K. Kushimoto, K. Komaguchi, I. Imae and Y. Harima, Org. Biomol. Chem., 2010, 8, 2756 RSC.
|
This journal is © The Royal Society of Chemistry 2015 |