DOI:
10.1039/C4RA13647K
(Communication)
RSC Adv., 2015,
5, 493-496
Brønsted acid-catalyzed hydroarylation of activated olefins†
Received
2nd November 2014
, Accepted 17th November 2014
First published on 19th November 2014
Abstract
A mild, regiospecific Brønsted acid-catalyzed hydroarylation of activated olefins, capable of the formation of quinone methide-like intermediates, has been investigated. Variously substituted 2- and 4-vinylphenols, 4-vinylaniline or 6-vinyl-naphthalen-2-ol were successfully implemented in a sequential protonation and Friedel–Crafts-type alkylation reaction of electron-rich arenes.
Introduction
A number of metal-catalyzed hydroarylations applying palladium,1 cobalt,2 rhodium,3 iridium,4 iron5 and other metal catalysts6 have been reported within the past 15 years. Besides that, it has been shown that Brønsted-acids are capable of promoting the coupling of electron-rich arenes with vinyl arenes7 and allylic and benzylic alcohols.8 However, all these protocols make use of strong acids and/or drastic reaction conditions thus limiting their applicability in conjunction with functionalized reactants.
We have recognized that hydroarylation of activated olefins, capable of forming a quinone methide-like intermediate,9 can be catalyzed by a number of mild Brønsted acids (pKa ∼ 3, see Table 1) simply by the generation of a stabilized carbocation and the following electrophilic substitution on electron-rich arenes (Scheme 1).10 The presence of hydroxyl or amino-group on the aryl attached to the double bond (the so-called activating group) enables application of relatively mild conditions for this kind of transformation. These substrates were applied in Pd-catalyzed hydroarylation by Sigman et al., however high catalyst loading, excess of the nucleophile and inert reaction conditions were necessary.1c Our strategy allowed for the use of 2- and 4-vinylphenols (–OH), 4-vinylaniline (–NH2) and 6-vinylnaphthalen-2-ol.
Table 1 Hydroarylation of 2-vinylphenol: optimizationa
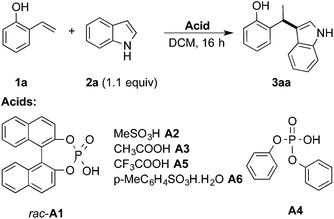
|
Entry |
Acid (x mol%) |
T (°C) |
Conv.b (%) |
Yieldb,c (%) |
Reaction conditions: 1a (60 mg, 0.50 mmol), 2a (64 mg, 0.55 mmol), acid in DCM (1 mL), 16 h (with 1a and 2a stock solution).
Determined by GC with isooctane as internal standard.
Isolated yields are given in parentheses.
40 hours.
|
1 |
rac-A1 (8) |
80 |
Quant. |
94 (80) |
2 |
rac-A1 (2) |
80 |
82 |
76 |
3 |
rac-A1 (1) |
80 |
59 |
58 |
4 |
rac-A1 (10) |
25d |
16 |
16 |
5 |
A2 (2) |
80 |
67 |
63 |
6 |
A3 (2) |
80 |
38 |
38 |
7 |
A4 (2) |
80 |
87 |
86 |
8 |
A5 (2) |
80 |
58 |
42 |
9 |
A6 (2) |
80 |
71 |
69 |
10 |
— |
80 |
27 |
26 |
11 |
A2 (5) |
60 |
37 |
33 |
12 |
A4 (5) |
60 |
57 |
57 |
13 |
A5 (5) |
60 |
49 |
46 |
14 |
A4 (5) |
80 |
94 |
93 (88) |
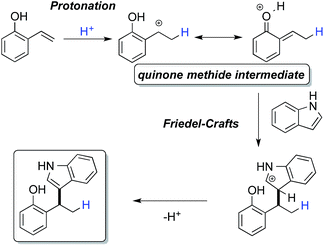 |
| Scheme 1 Proposed mechanism for the Brønsted acid-mediated hydroarylation of activated olefins. | |
Results and discussion
The initial tests of the reaction of 2-vinylphenol with indole were performed with racemic phosphoric acid A1 at 80 °C (entry 1, Table 1). A quantitative conversion was obtained with 8 mol% of the acid. The desired product 2-(1-(1H-indol-3-yl)ethyl)phenol (3aa) was isolated in 80% yield. A decrease in yield is correlated with a decrease of the percentage of acid used, thus demonstrating its importance in this reaction. However, even with only 1 mol% of rac-A1 a decent conversion of 58% to the desired product could be achieved (entry 3, Table 1) and, interestingly, 27% conversion was observed in the absence of the catalyst (entry 10, Table 1). Noteworthy, in previous protocols with different substrates, usually 10–20 mol% of strong acids (pTsOH,7c H2SO4 or CF3SO3H8a) have been used. Conversely, under the tested reaction conditions, strong acids did not lead to a full conversion of the starting materials (entries 5, 8 and 9, Table 1). Selected acids were also compared at lower temperature confirming that diphenyl hydrogen phosphate (A4) provides the best results (entries 11–13, Table 1). The most optimal outcome was achieved when 5 mol% of diphenyl hydrogen phosphate (A4) was used allowing to isolate 3aa in 88% yield (entry 14, Table 1).
It is well known that protonated 2-vinylphenol can be described with its ortho-quinone methide resonance structure. This mesomeric form both stabilizes the positive charge and reinforces nucleophilic attack on the exocyclic carbon. Pathak and Sigman have shown that also 4-vinylphenol yields the desired hydroarylation product when reacted with indole (15 equiv.) under palladium-catalyzed reaction conditions, albeit in low yield (38%).1c In the following, we have been interested if this mode of stabilization/reactivity can be translated to other structures able to form quinone methide-like intermediates applying Brønsted-acidic reaction conditions.
The results depicted in Table 2 support that the reaction is facilitated through the formation of an quinone methide intermediate that can be postulated for ortho- and para-vinylphenols (3aa and 3ba) and anilines (3ea). The corresponding products were obtained in 64–88% yields. The presence of a remote OH-group in a vinylnaphthalene derivative was also sufficient to promote the acid-catalyzed hydroarylation (3da). A substituent on the oxygen of the substrate had a disadvantageous influence on the reaction outcome, since higher temperature was necessary and several side-products were formed (3ca) impeding the isolation. Styrene derivatives lacking the possibility of mesomeric stabilization show no reactivity under the applied reaction conditions (3fa and 3ga).
Table 2 Reactivity in dependence of the accessibility of the quinone methide intermediatea
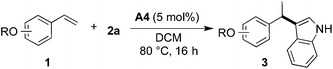
|
Reaction conditions: 1 (0.50 mmol), 2a (64 mg, 0.55 mmol), A4 (5.0 mol%), DCM (1 mL), 80 °C, 16 h. Isolated yields are given.
Reaction conditions: A4 (10 mol%), DCE (1 mL), 100 °C, 16 h. GC-yield.
|
|
Next we investigated the scope of the Brønsted acid catalyzed hydroarylation reaction (Table 3). Therein we especially concentrated on altering functional groups on the electrophile 1. In this respect, we could show that both functional groups attached to the phenol core (3ka–na) as well as substitution on the vinylic position (3ha, 3ia and 3ja) were well tolerated. To our surprise, sterically encumbered 2-(prop-1-en-2-yl)phenol 1h proved to be an excellent substrate, assumingly due to superior stabilization of the α-carbenium species. The corresponding product 3ha was isolated in 97% yield. Internal alkenes could also be successfully hydroarylated under Brønsted-acidic reaction conditions. 2-(buta-1,3-dien-1-yl)phenol reacted with indole under the formation of 2-(1-(1H-indol-3-yl)but-2-en-1-yl)phenol (3ia) in a combined yield of 67%. The E/Z stereoisomers (E/Z = 81
:
19) of 3ia could be separated by column chromatography. Likewise, 2-(prop-1-en-1-yl)phenol resulted in the formation of 2-(1-(1H-indol-3-yl)propyl)phenol (3ja) in 90% isolated yield, albeit under more forcing conditions. Noteworthy, no hydroarylation activity was evident starting from 2-allylphenol underlying the importance of the accessibility of a quinone methide intermediate.
Table 3 Hydroarylation of alkenes with indolea
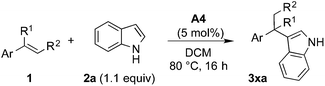
|
Reaction conditions: 1 (0.50 mmol), 2a (64 mg, 0.55 mmol), A4 (5.0 mol%), DCM (1 mL), 80 °C, 16 h.
A4 (10 mol%), DCE (1 mL), 100 °C, 16 h. Isolated yields are given.
|
|
Next, we investigated the scope of nucleophiles. Besides the excellent yields and expedient equimolar substrate ratios, in contrast to the Pd-catalyzed approach, the Brønsted-acid catalyzed hydroarylation tolerates halide substituents on the arene moiety (Table 4). 2-(1-(5-halo-1H-indol-3-yl)ethyl)phenol derivatives 3ab–3ad were obtained in moderate yields of 52–63%. 1-Methyl indole was converted to the corresponding product 3ae in 80% yield. In addition, two 2-substituted derivatives of indole were tested and the reaction provided the products 3af and 3ag in good yields. Unfortunately, 3-methyl indole furnished a mixture of the desired and oxidized compound and pyrrole led to a low conversion of a complex mixture.
Table 4 Hydroarylation of 2-vinylphenol with indole derivativesa
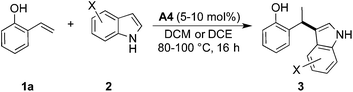
|
Reaction conditions: 1 (0.50 mmol), 2a (64 mg, 0.55 mmol), A4 (5.0 mol%), DCM (1 mL), 80 °C, 16 h.
A4 (10 mol%), DCE (1 mL), 100 °C, 16 h. Isolated yields are given.
|
|
Furthermore, we could demonstrate that the scope of the Brønsted-acid catalyzed hydroarylation can be further translated to common electron rich arenes, such as 1,3,5-trimethoxybenzene 5 (Table 5). It is noteworthy to mention that in this case an excess of the arene (2 equivalents) is required to obtain optimal results. Thus, 4-(1-(2,4,6-trimethoxyphenyl)-ethyl)phenol (6ba) was isolated in 91% on 5 mmol scale.
Table 5 Hydroarylation of selected alkenes with 1,3,5-trimethoxybenzene 5a
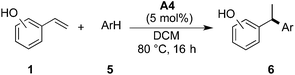
|
Reaction conditions: 1 (0.50 mmol), 5 (0.55 mmol), A4 (5.0 mol%) DCM (1 mL), 16 h. Isolated yields are given.
With 2 equiv. 1b, A4 (10 mol%), DCE (1 mL), 100 °C, 16 h.
|
|
Conclusions
In summary, we could demonstrate that the hydroarylation of activated olefins can be conveniently achieved by Brønsted-acid catalysis in the absence of transition-metal catalysts. The reaction follows a simple mechanism based on the formation of a stabilized carbocation, which serves as an electrophile in the Friedel–Crafts alkylation. The developed methodology does neither require the application of inert reaction conditions nor protection of the NH group of the indole. The hydroarylation products are obtained in moderate to excellent yields by applying a nearly equimolar substrate ratio. Compared to existing protocol, we extended the scope of the reaction to 4-vinylanilines and 6-vinylnaphthalene-2-ol and 5-halo indoles.
Our future endeavor encompasses the development of a stereoselective variant of the presented protocol by means of asymmetric Brønsted-acid catalysis.11–13
Acknowledgements
We are grateful to the Leibniz Institute of Catalysis, Prof. Dr Matthias Beller and Fonds der Chemischen Industrie (Liebig Fellowship for I.F.) for the financial support. We thank the analytical departments, workshops and glassblowers of the Leibniz Institute of Catalysis and University of Regensburg for their support; and Dr A. Spannenberg for crystallographic analysis.
Notes and references
- For selected examples, see:
(a) D. Drago and P. S. Pregosin, Organometallics, 2002, 21, 1208–1215 CrossRef CAS;
(b) C. Liu and R. A. Widenhoefer, Chem.–Eur. J., 2006, 12, 2371–2382 CrossRef CAS PubMed;
(c) T. P. Pathak and M. S. Sigman, Org. Lett., 2011, 13, 2774–2777 CrossRef CAS PubMed;
(d) S. M. Podhajsky, Y. Iwai, A. Cook-Sneathen and M. S. Sigman, Tetrahedron, 2011, 67, 4435–4441 CrossRef CAS PubMed.
- For selected examples, see:
(a) P.-S. Lee and N. Yoshikai, Angew. Chem., Int. Ed., 2013, 52, 1240–1244 CrossRef CAS PubMed;
(b) Z. Ding and N. Yoshikai, Angew. Chem., Int. Ed., 2013, 52, 8574–8578 CrossRef CAS PubMed;
(c) Z. Yang, H. Yu and Y. Fu, Chem.–Eur. J., 2013, 19, 12093–12103 CrossRef CAS PubMed.
-
(a) M. Beller, O. R. Thiel and H. Trauthwein, Synlett, 1999, 243–245 CrossRef CAS PubMed;
(b) R. Jana and J. A. Tunge, Org. Lett., 2009, 11, 971–974 CrossRef CAS PubMed;
(c) G. C. Tsui, F. Menard and M. Lautens, Org. Lett., 2010, 12, 2456–2459 CrossRef CAS PubMed.
-
(a) G. Bhalla, J. Oxgaard, W. A. Goddard and R. A. Periana, Organometallics, 2005, 24, 3229–3232 CrossRef CAS;
(b) C. S. Sevov and J. F. Hartwig, J. Am. Chem. Soc., 2013, 135, 2116–2119 CrossRef CAS PubMed.
-
(a) J. Kischel, I. Jovel, K. Mertins, A. Zapf and M. Beller, Org. Lett., 2005, 8, 19–22 CrossRef PubMed;
(b) S. Haldar and S. Koner, J. Org. Chem., 2010, 75, 6005–6008 CrossRef CAS PubMed.
- For selected examples, see:
(a) Y. Uchimaru, Chem. Commun., 1999, 1133–1134 RSC;
(b) M. Rueping, B. J. Nachtsheim and T. Scheidt, Org. Lett., 2006, 8, 3717–3719 CrossRef CAS PubMed;
(c) Z. Zhang, X. Wang and R. A. Widenhoefer, Chem. Commun., 2006, 3717–3719 RSC;
(d) M. Rueping, B. J. Nachtsheim and W. Ieawsuwan, Adv. Synth. Catal., 2006, 348, 1033–1037 CrossRef CAS;
(e) M.-Z. Wang, M.-K. Wong and C.-M. Che, Chem.–Eur. J., 2008, 14, 8353–8364 CrossRef CAS PubMed;
(f) T. Mukai, K. Hirano, T. Satoh and M. Miura, J. Org. Chem., 2009, 74, 6410–6413 CrossRef CAS PubMed;
(g) Y. Nakao, N. Kashihara, K. S. Kanyiva and T. Hiyama, Angew. Chem., Int. Ed., 2010, 49, 4451–4454 CrossRef CAS PubMed;
(h) W.-C. Shih, W.-C. Chen, Y.-C. Lai, M.-S. Yu, J.-J. Ho, G. P. A. Yap and T.-G. Ong, Org. Lett., 2012, 14, 2046–2049 CrossRef CAS PubMed.
-
(a) A. E. Cherian, G. J. Domski, J. M. Rose, E. B. Lobkovsky and G. W. Coates, Org. Lett., 2005, 7, 5135–5137 CrossRef CAS PubMed;
(b) X. Zhao, Z. Yu, T. Xu, P. Wu and H. Yu, Org. Lett., 2007, 9, 5263–5266 CrossRef CAS PubMed;
(c) T. P. Pathak, J. G. Osiak, R. M. Vaden, B. E. Welm and M. S. Sigman, Tetrahedron, 2012, 68, 5203–5208 CrossRef CAS PubMed.
-
(a) J. Le Bras and J. Muzart, Tetrahedron, 2007, 63, 7942–7948 CrossRef CAS PubMed;
(b) M. Barbero, S. Cadamuro, S. Dughera, M. Rucci, G. Spano and P. Venturello, Tetrahedron, 2014, 70, 1818–1826 CrossRef CAS PubMed.
-
(a) For review, see: T. P. Pathak and M. S. Sigman, J. Org. Chem., 2011, 76, 9210–9215 CrossRef CAS PubMed;
(b) For recent examples, see: J. C. Green, E. R. Brown and T. R. R. Pettus, Org. Lett., 2012, 14, 2929–2931 CrossRef CAS PubMed;
(c) M.-W. Chen, L.-L. Cao, Z.-S. Ye, G.-F. Jiang and Y.-G. Zhou, Chem. Commun., 2013, 49, 1660–1662 RSC;
(d) V. A. Osyanin, D. V. Osipov and Y. N. Klimochkin, J. Org. Chem., 2013, 78, 5505–5520 CrossRef CAS PubMed;
(e) T. H. Jepsen, S. B. Thomas, Y. Lin, C. I. Stathakis, I. de Miguel and S. A. Snyder, Angew. Chem., Int. Ed., 2014, 53, 6747–6751 CrossRef CAS PubMed;
(f) A. K. Shaikh and G. Varvounis, Org. Lett., 2014, 16, 1478–1481 CrossRef CAS PubMed.
- M. Rueping, B. J. Nachtsheim and B. J. Beilstein, J. Org. Chem., 2010, 6, 6 Search PubMed.
- For preliminary tests, see ESI.†.
- For reviews on asymmetric Brønsted acid catalysis, see:
(a) T. Akiyama, Chem. Rev., 2007, 107, 5744–5758 CrossRef CAS PubMed;
(b)
D. Kampen, C. M. Reisinger and B. List, in Asymmetric Organocatalysis, ed. B. List, 2009, Springer Verlag, pp. 395–456 Search PubMed;
(c) M. Terada, Synthesis, 2010, 1929–1982 CrossRef CAS PubMed;
(d) A. Zamfir, S. Schenker, M. Freund and S. B. Tsogoeva, Org. Biomol. Chem., 2010, 8, 5262–5276 CAS.
- Appeared during the preparation of the manuscript: O. El-Sepelgy, S. Haseloff, S. K. Alamsetti and C. Schneider, Angew. Chem., Int. Ed., 2014, 53, 7923 CrossRef CAS PubMed.
Footnote |
† Electronic supplementary information (ESI) available: Details of experimental procedures and physical properties of new compounds. CCDC 1016204. For ESI and crystallographic data in CIF or other electronic format see DOI: 10.1039/c4ra13647k |
|
This journal is © The Royal Society of Chemistry 2015 |