DOI:
10.1039/C5QO00208G
(Research Article)
Org. Chem. Front., 2015,
2, 1356-1360
Metal-free direct difunctionalization of alkenes with I2O5 and P(O)–H compounds leading to β-iodophosphates†
Received
1st July 2015
, Accepted 5th August 2015
First published on 6th August 2015
Abstract
A novel and efficient procedure for direct difunctionalization of alkenes with I2O5 and P(O)–H compounds has been developed under metal-free conditions. The present methodology produces a series of substituted β-iodophosphates in moderate to good yields with high regioselectivity and favorable functional group tolerance.
Alkenes are inexpensive and readily available chemical feedstocks and organic reactants, in which difunctionalization represents a class of reactions with significant synthetic potential accessing many useful and fascinating compounds.1 In recent years, many transition-metal-catalyzed difunctionalizations of alkenes have been developed, such as diamination,2 dioxygenation,3 aminooxygenation,4 oxyphosphorylation,5 and aminohalogenation.6 Nevertheless, the cost, toxicity and environmental impact of these metal-catalysts might thereby limit their applications on a large scale in the field of organic synthesis and pharmaceutical chemistry. The development of a convenient and efficient strategy for difunctionalization of alkenes via a metal-free process has become a challenging but highly attractive target. Despite great efforts being made in this field over the past several years, successful metal-free strategies are considerably fewer than their transition metal-based counterparts.7 With our continuous interest in metal-free difunctionalization of alkenes,8 here, we seek to develop a novel and convenient procedure for direct iodophosphorylation of alkenes with I2O5 and P(O)–H compounds leading to β-iodophosphates under metal-free conditions. | 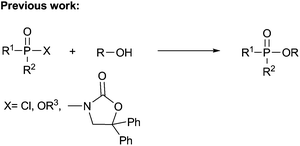 | (1) |
|  | (2) |
| 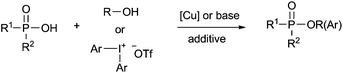 | (3) |
|  | (4) |
| 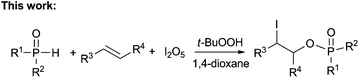 | (5) |
Organophosphates have attracted great interest from chemists and physiologists because they play significant roles in drug discovery9 and many major physiological processes such as energy transfer and regulation of ion release.10 They have also been extensively studied in various organic transformations11 and many agrochemicals such as insecticides and herbicides.12 Due to the prominent importance of these compounds in synthetic chemistry and molecular biology, various synthesis methods have been developed,13–21 most known are the methods using the nucleophilic substitution reactions of alcohols with highly air-sensitive and hazardous P(O)–Cl compounds in the presence of a base14 or transesterification of phosphate esters15 or phosphorylation of alcohols with N-phosphoryloxazolidinones16 (eqn (1)). Alternative preparation methods such as the reactions of phosphoramidites with the requisite alcohol followed by subsequent oxidation,17 base mediated phospha-Brook rearrangement,18 I2/H2O2 mediated phosphorylation of alcohols with P(O)–H compounds (eqn (2)),19 and copper-catalyzed aerobic oxidative esterification of P(O)–OH compounds with alcohols or diaryliodonium triflates20 (eqn (3)) have also been developed. However, most of them could suffer from some limitations such as inaccessible starting materials, relatively harsh reaction conditions, poor substrate scope, and use of a large amount of promoters or transition-metal catalysts. Recently, Tang and co-workers described a metal-free Bu4NI-catalyzed phosphorylation of benzyl C–H bonds leading to phosphate esters using TBHP as an oxidant (eqn (4)).21 Nevertheless, the substrate scope of this well developed reaction could be limited to toluene derivatives only. In the present work, a convenient and metal-free procedure has been developed for the synthesis of various β-iodophosphates from diverse and readily-available alkenes and P(O)–H compounds with high regioselectivity and favorable functional group tolerance (eqn (5)).
In an initial experiment, styrene 1a and diphenylphosphine oxide 2a were chosen as model substrates to optimize the reaction conditions in air. To our delight, among the various iodine reagents tested, I2O5 was found to be the optimal iodine source for the formation of the desired 3aa (80% yield) in the presence of TBHP (Table 1, entries 1–5), which was further demonstrated to be the best oxidant (Table 1, entries 5–10). Moreover, the screening of solvents showed that 1,4-dioxane was more effective than the others such as THF, DME, DCE, and CH3CN (Table 1, entries 5, 11–14). Interestingly, no conversion was observed when the reaction was performed in toluene, DMF, and DMSO (Table 1, entries 15–17). Also, the reaction efficiency was obviously low with the decreasing of I2O5 loading and reaction temperature (Table 1, entries 18–20). In addition, no product was detected when the reaction was conducted in the absence of I2O5 or at room temperature (Table 1, entries 18 and 21). After an extensive screening of the reaction parameters, the best yield of 3aa (80%) was obtained by employing 1.0 equiv. of I2O5 and 1.2 equiv. of TBHP in 1,4-dioxane at 80 °C (Table 1, entry 5).
Table 1 Optimization of the reaction conditionsa

|
Entry |
Iodine reagent |
Oxidant |
Solvent |
Yieldb (%) |
Reaction conditions: 1a (0.25 mmol), 2a (0.5 mmol), iodine reagent (0.25 mmol), oxidant (0.3 mmol), solvent (2 mL), 80 °C, 16 h. TBAI = (n-Bu)4NI; DME: 1,2-dimethoxyethane, DCE: 1,2-dichloroethane; TBHP: tert-butyl hydroperoxide, 5.5 M in decane, DTBP: di-tert-butyl peroxide, oxone: (2KHSO5·KHSO4·K2SO4).
Isolated yields based on 1a.
I2O5 (0.5 equiv.).
I2O5 (1.5 equiv.).
25 °C.
60 °C.
|
1 |
KI |
TBHP |
1,4-Dioxane |
0 |
2 |
NaI |
TBHP |
1,4-Dioxane |
0 |
3 |
I2 |
TBHP |
1,4-Dioxane |
0 |
4 |
TBAI |
TBHP |
1,4-Dioxane |
0 |
5 |
I2O5 |
TBHP |
1,4-Dioxane |
80 |
6 |
I2O5 |
DTBP |
1,4-Dioxane |
68 |
7 |
I2O5 |
K2S2O8 |
1,4-Dioxane |
23 |
8 |
I2O5 |
(NH4)S2O8 |
1,4-Dioxane |
Trace |
9 |
I2O5 |
Na2S2O8 |
1,4-Dioxane |
Trace |
10 |
I2O5 |
Oxone |
1,4-Dioxane |
43 |
11 |
I2O5 |
TBHP |
THF (reflux) |
58 |
12 |
I2O5 |
TBHP |
DME |
40 |
13 |
I2O5 |
TBHP |
DCE |
26 |
14 |
I2O5 |
TBHP |
CH3CN |
42 |
15 |
I2O5 |
TBHP |
Toluene |
0 |
16 |
I2O5 |
TBHP |
DMF |
0 |
17 |
I2O5 |
TBHP |
DMSO |
0 |
18 |
— |
TBHP |
1,4-Dioxane |
0 |
19 |
I2O5 |
TBHP |
1,4-Dioxane |
61c |
20 |
I2O5 |
TBHP |
1,4-Dioxane |
79d |
21 |
I2O5 |
TBHP |
1,4-Dioxane |
0e |
22 |
I2O5 |
TBHP |
1,4-Dioxane |
71f |
With the optimized conditions in hand, the scope and generality of this reaction was investigated. As shown in Table 2, both electron-rich and electron-deficient aromatic alkenes were suitable for this reaction, of which the corresponding products were obtained in moderate to good yields (3aa–3la). The reaction was not significantly affected by the steric effect. The ortho-, meta- or para-position of the phenyl moieties was compatible with this reaction (3ba–3ga). Moreover, various functionalities including halogen, chloromethyl, nitro, and cyano groups were also tolerated in this reaction leading to the products 3ea–3la, which could be employed for further transformations. 2-Vinylnaphthalene was also used to give the desired product 3ma in 71% yield. Notably, an internal aromatic alkene such as (E)-prop-1-enylbenzene and aliphatic alkenes such as 1-octene, cyclohexene, ethyl acrylate and N,N-diallyl-4-methylbenzenesulfonamide were also suitable for this protocol to generate the corresponding products (3na–3ra) in moderate to good yields. In addition to diphenylphosphine oxide, substituted diphenylphosphine oxide, diethyl phosphonates, and dibutyl phosphonates were all suitable substrates, with the corresponding products 3ab–3ad in moderate to good yields.
Table 2 Results for metal-free difunctionalization of alkenes with I2O5 and P(O)–H compoundsa,b
Reaction conditions: 1 (0.25 mmol), 2 (0.5 mmol), I2O5 (0.25 mmol), TBHP (0.3 mmol), 1,4-dioxane (2 mL), 80 °C, 16–24 h.
Isolated yields based on 1.
2 (0.75 mmol).
|
|
In order to obtain further insights into this reaction, several control experiments were conducted as demonstrated in eqn (6)–(9). When the reaction of diethyl phosphonate 2d with I2O5 was conducted in the absence of styrene, the corresponding diethyl hydrogen phosphate 4d was obtained in 48% yield (eqn (6)). Meanwhile, the formation of molecular iodine was confirmed by observation of an obvious color change from gray to deep blue when starch was added into the above reaction system.22 When the reaction of styrene 1a with 2d was conducted in the I2/TBHP system, the desired product 3ad was not detected (eqn (7)). Furthermore, the desired product 3ad was isolated in 62% yield, when the reaction of styrene 1a with 4d was performed in the presence of the I2/TBHP system (eqn (8)). The above results indicated that I2O5 played a key role in the formation of diethyl hydrogen phosphate 4d, which was the key intermediate in this difunctionalization reaction. Moreover, the iodophosphorylation reaction was completely inhibited when 2,2,6,6-tetramethyl-1-piperidinyloxy (TEMPO, a well-known radical scavenger) was added in this reaction system, and a TEMPO-trapped complex (Ph2P(O)–O-Tempo) was detected by LC-MS analysis (see the ESI†). This result suggested that a P(O)–O radical might exist in this reaction system and the present reaction should proceed through a radical pathway (eqn (9)).
| 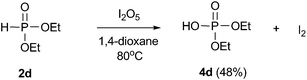 | (6) |
| 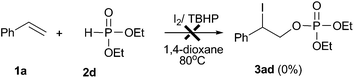 | (7) |
| 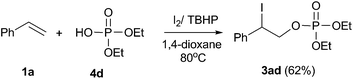 | (8) |
| 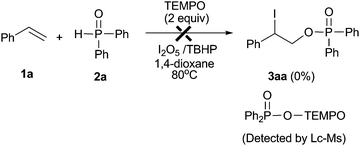 | (9) |
According to the aforementioned information and based on some previous reports,22–24 a possible reaction pathway for this transformation is outlined in Scheme 1. Initially, the oxidation of R2P(O)–H compound 2 with I2O5 would produce the corresponding R2P(O)–OH 4 and I2. Subsequently, a radical intermediate 5 was formed by the interaction of R2P(O)–OH 4 with an alkoxyl radical and a hydroxyl radical, which were generated from a homolytic cleavage of tert-butyl hydroperoxide.23,24 Next, selective addition of radical 5 to alkene 1 afforded alkyl radical 6, which further interacted with molecular iodine leading to the formation of the desired product 3.
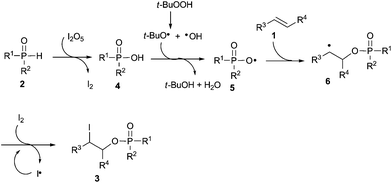 |
| Scheme 1 Tentative reaction pathway. | |
In conclusion, we have developed a novel and efficient metal-free synthesis method for the construction of β-iodophosphates via the direct difunctionalization of alkenes with I2O5 and P(O)–H compounds. A variety of biologically important phosphate esters could be obtained in moderate to good yields from readily-available starting materials of alkenes with high regioselectivity and excellent functional group tolerance. This simple and metal-free reaction system is expected to extend the potential applications of functionalized organophosphates in the synthetic and pharmaceutical chemistry.
This work was supported by the National Natural Science Foundation of China (no. 21302109, 21302110, and 21375075), the Taishan Scholar Foundation of Shandong Province, the Natural Science Foundation of Shandong Province (ZR2015JL004), and the Excellent Middle-Aged and Young Scientist Award Foundation of Shandong Province (BS2013YY019).
References
- For selected reviews, see:
(a)
P. A. Sibbald, Palladium-catalyzed oxidative difunctionalization of alkenes: New reactivity and new mechanisms, ProQuest UMI Dissertation Publishing, Ann Arbor, MI, 2011 Search PubMed;
(b)
B. Jacques and K. Muinz, in Catalyzed carbon-heteroatom bond formation, ed. A. K. Yudin, Wiley, VCH, Weinheim, Germany, 2011 Search PubMed;
(c) R. I. McDonald, G. Liu and S. S. Stahl, Chem. Rev., 2011, 111, 2981 CrossRef CAS PubMed;
(d) K. H. Jensen and M. S. Sigman, Org. Biomol. Chem., 2008, 6, 4083 RSC.
- For selected diamination, see:
(a) G. L. J. Bar, G. C. Lloyd-Jones and K. I. Booker-Milbum, J. Am. Chem. Soc., 2005, 127, 7308 CrossRef CAS PubMed;
(b) H. Du, B. Zhao and Y. A. Shi, J. Am. Chem. Soc., 2007, 129, 762 CrossRef CAS PubMed;
(c) H. Du, W. Yuan, B. G. Zhao and Y. A. Shi, J. Am. Chem. Soc., 2007, 129, 11688 CrossRef CAS PubMed;
(d) P. A. Sibbald and F. E. Michael, Org. Lett., 2009, 11, 1147 CrossRef CAS PubMed.
- For selected dioxygenation, see:
(a) C. J. R. Bataille and T. J. Donohoe, Chem. Soc. Rev., 2011, 40, 114 RSC;
(b) Q. Xue, J. Xie, P. Xu, K. Hu, Y. Cheng and C. Zhu, ACS Catal., 2013, 3, 1365 CrossRef CAS;
(c) V. A. Schmidt and E. J. Alexanian, Angew. Chem., Int. Ed., 2010, 49, 4491 CrossRef CAS PubMed;
(d) B. C. Giglio, V. A. Schmidt and E. J. Alexanian, J. Am. Chem. Soc., 2011, 133, 13320 CrossRef CAS PubMed;
(e) A. Wang, H. Jiang and H. Chen, J. Am. Chem. Soc., 2009, 131, 3846 CrossRef CAS PubMed;
(f) G. A. Abeykoon, S. Chatterjee and J. S. Chen, Org. Lett., 2014, 16, 3248 CrossRef CAS PubMed;
(g) X.-F. Xia, S.-L. Zhu, Z. Gu, H. Wang, W. Li, X. Liu and Y.-M. Liang, J. Org. Chem., 2015, 80, 5572 CrossRef CAS PubMed.
-
(a) G. Liu and S. S. Stahl, J. Am. Chem. Soc., 2006, 128, 7179 CrossRef CAS PubMed;
(b) P. H. Fuller, J.-W. Kim and S. R. Chemler, J. Am. Chem. Soc., 2008, 130, 17638 CrossRef CAS PubMed;
(c) M. C. Paderes, J. B. Keister and S. R. Chemler, J. Org. Chem., 2013, 78, 506 CrossRef CAS PubMed.
-
(a) W. Wei and J. Ji, Angew. Chem., Int. Ed., 2011, 50, 9097 CrossRef CAS PubMed;
(b) S.-F. Zhou, D.-P. Li, K. Liu, J.-P. Zou and O. T. J. Asekun, Org. Chem., 2015, 80, 1214 CrossRef CAS PubMed.
-
(a) T. Wu, G. Yin and G. Liu, J. Am. Chem. Soc., 2009, 131, 16354 CrossRef CAS PubMed;
(b) S. Qiu, T. Xu, J. Zhou, Y. Guo and G. Liu, J. Am. Chem. Soc., 2010, 132, 2856 CrossRef CAS PubMed;
(c) S. R. Chemler and M. T. Bovino, ACS Catal., 2013, 3, 1076 CrossRef CAS;
(d) X. Ji, H. Huang, W. Wu and H. Jiang, J. Am. Chem. Soc., 2013, 135, 5286 CrossRef CAS PubMed.
-
(a) R. N. Reddi, P. K. Prasad and A. Sudalai, Org. Lett., 2014, 16, 5674 CrossRef CAS PubMed;
(b) Q. Xue, J. Xie, P. Xu, K. Hu, Y. Cheng and C. Zhu, ACS Catal., 2013, 3, 1365 CrossRef CAS;
(c) L. Huang, S.-C. Zheng, B. Tan and X.-Y. Liu, Org. Lett., 2015, 17, 1589 CrossRef CAS PubMed;
(d) B. Zhang and A. Studer, Org. Lett., 2013, 15, 4548 CrossRef CAS PubMed;
(e) B. C. Giglio, V. A. Schmidt and E. J. Alexanian, J. Am. Chem. Soc., 2011, 133, 13320 CrossRef CAS PubMed;
(f) Y. Li, M. Hartmann, C. G. Daniliuc and A. Studer, Chem. Commun., 2015, 51, 5706 RSC;
(g) Y.-M. Li, X.-H. Wei, X.-A. Li and S.-D. Yang, Chem. Commun., 2013, 49, 11701 RSC;
(h) V. A. Schmidt and E. J. Alexanian, Chem. Sci., 2012, 3, 1672 RSC;
(i) V. A. Schmidt and E. J. Alexanian, J. Am. Chem. Soc., 2011, 133, 11402 CrossRef CAS PubMed;
(j) K. M. Jones and N. C. O. Tomkinson, J. Org. Chem., 2012, 77, 921 CrossRef CAS PubMed;
(k) Q. Liu, Q. Y. Zhao, J. Liu, P. Wu, H. Yi and A. Lei, Chem. Commun., 2012, 48, 3239 RSC;
(l) Y. Kang and L. H. Gade, J. Am. Chem. Soc., 2011, 133, 3658 CrossRef CAS PubMed.
-
(a) W. Wei, J. Wen, D. Yang, J. Du, J. You and H. Wang, Green Chem., 2014, 16, 2988 RSC;
(b) W. Wei, J. Wen, D. Yang, X. Liu, M. Guo, R. Dong and H. Wang, J. Org. Chem., 2014, 79, 4225 CrossRef CAS PubMed;
(c) W. Wei, X. Liu, D. Yang, R. Dong, Y. Cui, F. Yuan and H. Wang, Tetrahedron Lett., 2015, 56, 1808 CrossRef CAS PubMed.
-
(a) C. Schultz, Bioorg. Med. Chem., 2003, 11, 885 CrossRef CAS;
(b) C. Anastasi, G. Quelever, S. Burlet, C. Garino, F. Souard and J. L. Kraus, Curr. Med. Chem., 2003, 10, 1825 CrossRef CAS;
(c) C. Ducho, U. Görbig, S. Jessel, N. Gisch, J. Balzarini and C. Meier, J. Med. Chem., 2007, 50, 1335 CrossRef CAS PubMed;
(d) S. J. Hecker and M. D. Erion, J. Med. Chem., 2008, 51, 2328 CrossRef CAS PubMed;
(e) M. S. Markoulides and A. C. Regan, Tetrahedron Lett., 2011, 52, 2954 CrossRef CAS PubMed;
(f) U. Pradere, E. C. Garnier-Amblard, S. J. Coats, F. Amblard and R. F. Schinazi, Chem. Rev., 2014, 114, 9154 CrossRef CAS PubMed.
-
(a)
L. D. Quin, in A Guide to Organophosphorus Chemistry, Wiley-Interscience, New York, 2000, pp. 351–357 Search PubMed;
(b)
J. C. Tebby, D. W. Allen, D. Loakes and R. Pajkert, Organophoshorus Chemistry, RSC, Cambridge, 2010, vol. 39 Search PubMed;
(c) H. F. Westheimer, Science, 1987, 235, 1173 Search PubMed;
(d) S. J. Hecker and M. D. Erion, J. Med. Chem., 2008, 51, 2328 CrossRef CAS PubMed;
(e) J. Rautio, H. Kumpulainen, T. Heimbach, R. Oliyai, D. Oh, T. Järvinen and J. Savolainen, Nat. Rev. Drug Discovery, 2008, 7, 255 CrossRef CAS PubMed.
-
(a) S. Protti and M. Fagnoni, Chem. Commun., 2008, 3611 RSC. Cross coupling reactions:
(b) M. McLaughlin, Org. Lett., 2005, 7, 4875 CrossRef CAS PubMed;
(c) C. C. Kofink and P. Knochel, Org. Lett., 2006, 8, 4121 CrossRef CAS PubMed;
(d) P. Zhang, J. Xu, Y. Gao, X. Li, G. Tang and Y. Zhao, Synlett, 2014, 2928 CAS. Alcohol to azide:
(e) C. Yu, B. Liu and L. Hu, Org. Lett., 2000, 2, 1959 CrossRef CAS PubMed;
(f) Q. Zhu and M. S. Tremblay, Bioorg. Med. Chem. Lett., 2006, 16, 6170 CrossRef CAS PubMed;
(g) S.-Y. Ji, Y.-M. Sun, H. Zhang, Q.-G. Hou and C.-Q. Zhao, Tetrahedron Lett., 2014, 55, 5742 CrossRef CAS PubMed.
-
(a) R. Neumann and H. H. Peter, Experientia, 1987, 43, 1235 CrossRef CAS;
(b) W. H. He, Phosphorus, Sulfur Silicon Relat. Elem., 2008, 183, 266 CrossRef PubMed;
(c) J. Wink, F. R. Schmidt, G. Seibert and W. Aretz, J. Antibiot., 2002, 55, 472 CrossRef CAS;
(d) L. Vìrtesy, B. Beck, M. Brönstrup, K. Ehrlich, M. Kurz, G. Mûller, D. Schummer and G. Seibert, J. Antibiot., 2002, 55, 480 CrossRef;
(e)
T. Kiely, D. Donaldson and A. Grube, EPA-733-R-04-001, Office of Pesticide Programs, U.S. Environmental Protection Agency, Washington, DC, 2004.
-
(a)
L. D. Quin, in A Guide to Organophosphorus Chemistry, Wiley-Interscience, New York, 2000, p. 351 Search PubMed;
(b)
J. C. Tebby, D. W. Allen, D. Loakes and R. Pajkert, Organophoshorus Chemistry, RSC, Cambridge, 2010, vol. 39 Search PubMed;
(c) L. A. Slotin, Synthesis, 1977, 737 CrossRef CAS;
(d) T. Wang, S. Chen, A. Shao, M. Gao, Y. Huang and A. Lei, Org. Lett., 2015, 17, 118 CrossRef CAS PubMed;
(e) J. Ke, Y. Tang, H. Yi, Y. Li, Y. Cheng, C. Liu and A. Lei, Angew. Chem., Int. Ed., 2015, 54, 6604 CrossRef CAS PubMed.
-
(a) K. A. Pollart and H. J. Harwood, J. Org. Chem., 1962, 27, 4444 CrossRef CAS;
(b) Y. Segall, E. Shirin and I. Granoth, Phosphorus, Sulfur Silicon Relat. Elem., 1980, 8, 243 CrossRef CAS PubMed;
(c) M. J. P. Harger and S. Westlake, Tetrahedron, 1982, 38, 1511 CrossRef CAS;
(d) M. Hatano, T. Mizuno and K. Ishihara, Chem. Commun., 2010, 46, 5443 RSC;
(e) M. Sathe, A. K. Gupta and M. P. Kaushik, Tetrahedron Lett., 2006, 47, 3107 CrossRef CAS PubMed;
(f) S. Pisarek, H. Bednarski and D. Gryko, Synlett, 2012, 2667 CAS.
- R. Singh and S. P. Nolan, Chem. Commun., 2005, 5456 RSC.
- S. Jones and C. Smanmoo, Org. Lett., 2006, 7, 3271 CrossRef PubMed.
-
(a) J. W. Perich and R. B. Johns, Tetrahedron Lett., 1987, 28, 101 CrossRef CAS;
(b) J. K. Stowell and T. S. Widlanski, Tetrahedron Lett., 1995, 36, 1825 CrossRef CAS;
(c) V. B. Oza and R. C. Corcoran, J. Org. Chem., 1995, 60, 3680 CrossRef CAS.
- G. Pallikonda, R. Santosh, S. Ghosal and M. Chakravarty, Tetrahedron Lett., 2015, 56, 3796 CrossRef CAS PubMed and references in.
- J. Dhineshkumar and K. R. Prabhu, Org. Lett., 2013, 15, 6062 CrossRef CAS PubMed.
- B. Xiong, X. Feng, L. Zhu, T. Chen, Y. Zhou, C.-T. Au and S.-F. Yin, ACS Catal., 2015, 5, 537 CrossRef CAS.
- J. Xu, P. Zhang, X. Li, Y. Gao, J. Wu, G. Tang and Y. Zhao, Adv. Synth. Catal., 2014, 356, 3331 CrossRef CAS PubMed.
-
(a) K. C. Nicolaou, T. Montagnon and P. S. Baran, Angew. Chem., Int. Ed., 2002, 41, 1386 CrossRef CAS;
(b) L. Chai, Y. Zhao, Q. Sheng and Z.-Q. Liu, Tetrahedron Lett., 2006, 47, 9283 CrossRef CAS PubMed;
(c) Z.-Q. Liu, Y. Zhao, H. Luo, L. Chai and Q. Sheng, Tetrahedron Lett., 2007, 48, 3017 CrossRef CAS PubMed;
(d) Z. Hang, Z. Li and Z.-Q. Liu, Org. Lett., 2014, 16, 3648 CrossRef CAS PubMed;
(e) L. Zhang, Z. Li and Z.-Q. Liu, Org. Lett., 2014, 16, 3688 CrossRef CAS PubMed;
(f) J. Wen, W. Wei, S. Xue, D. Yang, Y. Lou, C. Gao and H. Wang, J. Org. Chem., 2015, 80, 4966 CrossRef CAS PubMed.
-
(a) L. S. Silbert, J. Am. Oil Chem. Soc., 1969, 46, 615 CrossRef CAS;
(b) E. Shi, S. Chen, S. Chen, H. Hu, S. Chen, J. Zhang and X. Wan, Org. Lett., 2012, 14, 3384 CrossRef CAS PubMed;
(c) Q. Xue, J. Xie, P. Xu, K. Hu, Y. Cheng and C. Zhu, ACS Catal., 2013, 3, 1365 CrossRef CAS;
(d) S. Tang, Y. Wu, W. Liao, R. Bai, C. Liu and A. Lei, Chem. Commun., 2014, 50, 4496 RSC;
(e) D. Liu and A. Lei, Chem. – Asian J., 2015, 10, 806 CrossRef CAS PubMed;
(f) S. Tang, K. Liu, Y. Long, X. Qi, Y. Lan and A. Lei, Chem. Commun., 2015, 51, 8769 RSC.
-
(a) T. He, L. Yu, L. Zhang, L. Wang and M. Wang, Org. Lett., 2011, 13, 5016 CrossRef CAS PubMed;
(b) Z.-Q. Liu, L. Sun, J.-G. Wang, J. Han, Y.-K. Zhao and B. Zhou, Org. Lett., 2009, 11, 1437 CrossRef CAS PubMed;
(c) D. Liu, C. Liu, H. Li and A. Lei, Angew. Chem., Int. Ed., 2013, 52, 4453 CrossRef CAS PubMed;
(d) D. Liu, C. Liu, H. Li and A. Lei, Chem. Commun., 2014, 50, 3623 RSC.
Footnotes |
† Electronic supplementary information (ESI) available: Experimental details. See DOI: 10.1039/c5qo00208g |
‡ Authors with equal contribution. |
|
This journal is © the Partner Organisations 2015 |