DOI:
10.1039/C5QO00169B
(Research Article)
Org. Chem. Front., 2015,
2, 1059-1065
Kinetic resolution of racemic 5-alkylcyclohexenones via Pd(II)-catalyzed 1,4-additions of arylboronic acids – access to trans 3-alkyl-5-arylcyclohexanones†
Received
27th May 2015
, Accepted 2nd July 2015
First published on 3rd July 2015
Abstract
The kinetic resolution of racemic 5-substituted alkylcyclohexenones via 1, 4-conjugate addition with a variety of arylboronic acids was achieved by utilizing a ferrocenyl phosphapalladacycle catalyst. The addition reaction proceeded smoothly to afford trans-3-alkyl-5-arylcyclohexanones in good yields and selectivities.
Introduction
Optically active multi-substituted cycloalkanones are valuable precursors for a myriad of synthetic chiral building blocks and biologically relevant compounds.1
The preparation of these molecular motifs typically entails the conjugate addition of C nucleophiles to enantiomerically pure cycloalkenones.2 Such scalemic cycloalkenones in turn lack an extensive natural library and thus require laborious synthetic protocols for their procurement.1a,2e,3 Therefore, much focus in this field converges upon the kinetic resolution of readily available racemic mono-substituted cycloalkenones via catalyzed 1,4-additions.4
Of particular interest amongst this class of compounds are 3,5-disubstituted cyclohexanones by virtue of their utility in further synthetic manipulations that yield linear chiral building blocks as well as spiro-oxindole skeletons of interest (Scheme 1).1a,c,d
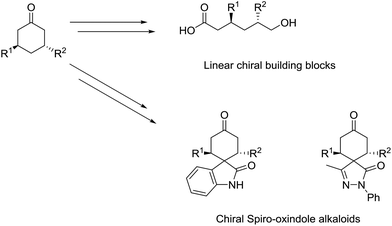 |
| Scheme 1 Further elaborations based on 3,5-disubstituted cyclohexenones. | |
Seminal endeavors by Tomioka4a and Feringa4bet al. have established the remarkably efficient resolution of 5-substituted cyclohexenones using Cu(I)/(II) phosphoramidite complexes with Zn C nucleophiles. However, ensuing advances employing Rh(I) systems on similar substrates are plagued with low diastereoselectivity in spite of excellent enantioselectivity due to the predominance of catalyst control.4a,4c Similarly, the utilization of Cu systems in which substrate control predominates suffers from moderate enantioselectivities.4b,4e These examinations are also limited to the collegial reliance on either thermal activation, or the administration of moisture sensitive organo-alkylzinc reagents.
The propensity of 3,6-disubstituted cycloalkanones to undergo subsequent epimerization yielding thermodynamically stable trans products justifies the use of the aforementioned Rh(I)/Cu protocols. The kinetic resolution of 6-monosubstituted cyclohexenones thus yields trans-2,5-disubstituted cycloalkanones exclusively upon subsequent treatment with bases.4g–j
It is thus prudent to develop a methodology which allows facile access to optically active 3,5-disubstituted cycloalkanones, specifically 3-alkyl-5-aryl cycloalkanones utilizing moisture/air stable reagents.
The preference of Pd catalysts for promoting the competitive and (in this instance) undesirable Heck reaction pathway have contributed to their relative lack of prominence in the field of Michael additions.5 As part of our continuing interest in the development and application of palladacycles in C–C6 and C–X7 (X = P, N) bond formation reactions, we have recently reported their efficacy for the 1,4-addition of arylboronic acids to acyclic enones8 as well as a preliminary study on the catalytic potential of a novel ferrocenyl phosphapalladacycle for the addition of (p-methoxy)phenyl boronic acid to cyclohexenone.9 Encouraged by these preliminary results, we sought to evaluate a series of palladacycles for the kinetic resolution of racemic 5-alkylcyclohexenones via arylboronic acid additions that provides facile access to optically active 3,5-disubstituted cycloalkanones, specifically 3-alkyl-5-aryl cycloalkanones utilizing moisture/air stable reagents.
Results and discussion
Adopting prior optimized reaction conditions, we screened a series of palladacycles for the kinetic resolution of racemic 5-methyl cyclohexenone (1a) with 0.6 equivalents of phenylboronic acid (2a) in toluene (Table 1). To our delight, the resolution proceeded smoothly when the ferrocenyl phosphapalladacycle (RcSpl)-C4 was employed, affording trans-(3R, 5R)-3-methyl-5-phenylcyclohexanone (3a) as the major adduct in 48% isolated yield, 95
:
5 dr and 70% ee10 after 18 hours at RT. (Table 1, entry 1) The palladacycles C1 and C2 only furnished trace products even after extended reaction times (48 h) while C3 afforded 19% conversion after 18 hours. Further examination revealed K3PO4 (5 M in H2O) to be the base of choice. In an attempt to enhance selectivity, a series of low temperature studies were conducted (Table 1, entries 7–9) and trans-(3R, 5R)-3a was attained in 45% isolated yield, with accompanying 97
:
3 dr and 81% ee when the addition was conducted at 0 °C. In addition, (S)-1a was recovered in a 47% yield and 71% ee, thus ruling out a simultaneous parallel kinetic resolution.
Table 1 Kinetic resolution of racemic 5-methylcyclohexenone 1a with phenylboronic acid 2aa
Having now determined the optimized conditions (Table 1, entry 7) we next concerted our efforts toward the addition of various functionalized arylboronic acid derivatives onto monosubstituted racemic cyclohexenones and these results are displayed in Table 2.
Table 2 Kinetic Resolution of racemic monosubstituted cyclohexenones with functionalized arylboronic acidsa
In general, satisfactory conversions were achieved with excellent dr (up to >99
:
1) and moderate to good ee values (up to 87%). The influence of the aryl fragment (Table 2, entries 1–10) on yields and selectivities is typically homogenous, with deviations observed due to increased sterics (Table 2, entry 2: o-tolylboronic acid with 5-methyl cyclohexenone; entry 11: phenylboronic acid with 4-methyl cyclohexenone) and when p-hydroxyphenylboronic acid was employed. Interestingly, both electron-withdrawing and donating groups are well tolerated in this protocol and yield similar results (Table 2, entries 8 & 9). The poor result obtained when p-hydroxylphenyl boronic acid was used (Table 2, entry 10) could be attributed to its poor solubility in toluene, thus requiring MeOH as a co-solvent and the addition to be conducted at RT. Increasing the bulkiness of the 5-substituted position led to lower yields, improved de and slightly diminished ee when phenylboronic acid was used (Table 2, entry 1 vs. entries 12–14).
Intrigued by the efficacy of this protocol, we decided to investigate the basis of the observed catalyst–substrate selectivity control by computational studies. The mechanism of Pd-catalyzed conjugate addition of arylboronic acids to enones has been comprehensively examined,11 and is widely acknowledged to involve transmetalation, carbopalladation (β-insertion) and protonation steps. Although no report concerning phosphapalladacycles has been established, we postulate that an analogous catalytic cycle is followed by our system. We base our supposition on the following: (i) A recent publication by Fairlamb et al. substantiates the hypothesis that the aryl moiety attunes itself to the position cis to the carbon ligand after transmetalation.11b (ii) The β-insertion step involves a square-planar four-membered cyclic transition state. With these fundamental requirements in mind, there are four possible isomeric alkene insertion transition states. The 3D structures of these reaction intermediates and transition states are shown in Scheme 2.
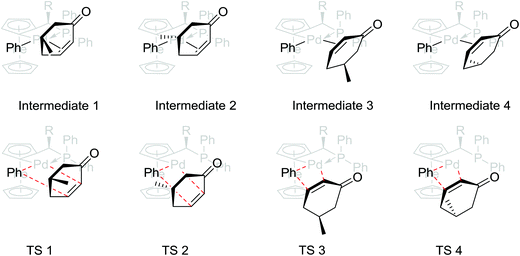 |
| Scheme 2 Reaction intermediates and transition states. | |
In all the transition states, the aryl group is situated cis to the carbon ligand. The discrimination of substrate by the catalyst is due to the steric hindrance that exists between the sp3 carbons of cyclohexenone (TS 3 and 4) and the ferrocene scaffold or between the alkyl pendant and the ferrocene sandwich (TS 2). The combination of these two steric effects thus favours the addition to proceed preferentially via TS 1, with the lowest computed activation barrier of 9.10 kcal mol−1, leading to a product with 3R, 5R configurations as seen in our studies.
In summary, we have developed a kinetic resolution of racemic 5-alkylcyclohexenones via arylboronic acid additions that provides facile access to optically active trans-3-alkyl-5-aryl cycloalkanones utilizing moisture/air stable reagents with excellent diastereomeric ratios and good enantiocontrol. This is the first instance in the literature that a desirable balance between catalyst control and substrate control has been achieved using a Pd(II) catalyst at ambient temperature for this synthetic protocol. Examination of possible transition states revealed the source of the diastereo-/enantioselectivity control, which is in agreement with experimental observations.
Experimental section
General information
Thin layer chromatography was performed on Merck silica gel 60 F254 aluminum backed plates and visualized under UV. Flash chromatography was performed using Merck silica gel 60. NMR spectra were recorded on a Bruker AV 300 spectrometer (1H at 300 MHz, 13C at 75 MHz, 31P at 121 MHz, 19F {1H} at 282 MHz), Bruker AV 400 spectrometer (1H at 400 MHz, 13C at 100 MHz, 31P at 162 MHz) or Bruker AV 500 spectrometer (1H at 500 MHz, 13C at 125 MHz, 31P at 202 MHz). 1H spectra are referenced to an internal SiMe4 standard at δ 0 ppm or to CDCl3 at δ 7.26 ppm. Optical rotations were measured on the specified solution in a 0.1 dm cell at 20 °C with a Perkin-Elmer 341 polarimeter. Melting points were documented on a SRS Optimelt MPA 100 point system. Mass spectrometry was obtained in ESI mode on a Thermo Finnigan LCQ Deca XP MAX system. HRMS (ESI) were recorded on a time-of-flight (TOF) LC/MS instrument.
General procedure for the synthesis of 3-alkyl-5-arylcyclohexanones
To a 5 ml vial were added boronic acid (0.1 mmol, 0.6 eq.) and catalyst (4.17 × 10−3 mmol, 2.5 mol%). The orange solution was allowed to stir for 10 min at 0 °C before racemic cyclohexenone (0.17 mmol, 1 eq.) and 5M K3PO4 (0.1 mmol, 0.6 eq.) were sequentially added. Upon stirring for 18 or 24 h, the resulting solution was passed through a short plug of silica gel (5n-hexanes
:
1EA) to yield both 3-aryl, 5-alkyl cyclohexanone (Rf: 0.40) and 5-alkyl cyclohexenone (Rf: 0.45) as colorless oils.
3-Methyl-5-phenyl cyclohexanone (3a)
Colorless oil. 3a was determined to be trans by comparison with previous literature.4c The 1H and 13C NMR spectra are in accordance with similar transformations in literature.4c1H NMR (CDCl3, 400 MHz): δ 1.03 (d, 3H, –CH3, 3J = 5.6 Hz), 1.84–1.89 (m, 1H), 2.05–2.10 (m, 1H), 2.15–2.19 (m, 1H), 2.26–2.29 (m, 1H), 2.54–2.64 (m, 3H), 3.36–3.40 (m, 1H, –CHPh), 7.20–7.24 (m, 3H, –CHPh), 7.30–7.33 (m, 2H, –CHPh) 13C NMR (CDCl3, 100 MHz): δ 20.3, 29.3, 39.4, 39.6, 47.2, 48.5, 126.5, 127.0, 128.6, 144.4, 211.6 MS (m/z) 189.07 HRMS (ESI) calcd for C13H17O [M + H]+ 189.1279, found 189.1282 [α]D = −22.32 (c 0.50, DCM) The diastereomeric and enantiomeric ratios were determined via HPLC using a chiral column (Daicel Chiralpak IC), n-hexanes
:
IPA = 98
:
2, 0.5 ml min−1, 210 nm, 28.9 min (major), 32.2 (minor), 33.3 (minor), 35.4 (minor).
For 1a:
[α]D = 66.2 (c 0.50, CHCl3) for 71% ee, lit2h [α]D = −74.6 (R) (c 0.5, CHCl3, 80% ee).
3-Methyl-5-(o-tolyl)cyclohexanone (3b)
Colorless oil. 3b was determined to be trans by comparison with previous literature.4c The 1H and 13C NMR spectra are in accordance with similar transformations in literature.4c1H NMR (CDCl3, 400 MHz): δ 1.05 (d, 3H, –CH3, 3J = 6.8 Hz), 1.76–1.82 (m, 1H), 1.95–2.02 (m, 1H), 2.18–2.22 (m, 1H), 2.34 (s, 3H, –ArMe), 2.47–2.63 (m, 3H), 3.53–3.60 (m, 1H, –CHAr), 7.11–7.21 (m, 4H, –Ar) 13C NMR (CDCl3, 100 MHz): δ 19.3, 20.1, 29.4, 35.2, 38.1, 47.0, 48.4, 125.7, 126.3, 126.4, 130.7, 135.2, 142.4, 211.9 MS (m/z) 203.05 HRMS (ESI) calcd for C14H19O [M + H]+ 203.1436, found 203.1433 [α]D = −25.99 (c 0.4, DCM) The diastereomeric and enantiomeric ratios were determined via HPLC using a chiral column (Daicel Chiralpak IC), n-hexanes
:
IPA = 98
:
2, 210 nm, 0.5 ml min−1, 23.1 min (major), 26.4 min (minor).
For 1a:
[α]D = 47.6 (c 0.50, CHCl3) for 51% ee, lit2h [α]D = −74.6 (R) (c 0.5, CHCl3, 80% ee).
3-Methyl-5-(m-tolyl)cyclohexanone (3c)
Colorless oil. 1H NMR (CDCl3, 400 MHz): δ 1.01 (d, 3H, –CH3, J = 7.2 Hz), 1.81–1.86 (m, 1H), 2.02–2.09 (m, 1H), 2.12–2.17 (m, 1H), 2.25–2.31 (m, 1H), 2.33 (s, 3H, –ArMe), 2.52–2.62 (m, 3H), 3.29–3.36 (m, 1H, –CHAr), 6.99–7.03 (m, 3H, –Ar), 7.17–7.21 (m, 1H, –Ar) 13C NMR (CDCl3, 100 MHz): δ 20.3, 21.5, 29.3, 39.5, 47.3, 48.4, 124.0, 127.3, 127.8, 128.5, 138.2, 144.4, 211.7 MS (m/z) 203.03 HRMS (ESI) calcd for C14H19O [M + H]+ 203.1436, found 203.1437 [α]D = −20.04 (c 0.3, DCM) The diastereomeric and enantiomeric ratios were determined via HPLC using a chiral column (Daicel Chiralpak IC), n-hexanes
:
IPA = 98
:
2, 0.5 ml min−1, 210 nm, 21.5 min (major), 22.8 min (minor), 24.4 min (minor), 24.8 min (minor).
For 1a:
[α]D = 59.7 (c 0.50, CHCl3) for 64% ee, lit2h [α]D = −74.6 (R) (c 0.5, CHCl3, 80% ee).
3-Methyl-5-(p-tolyl)cyclohexanone (3d)
Colorless oil. 1H NMR (CDCl3, 400 MHz): δ 1.02 (d, 3H, –CH3, J = 6.92 Hz), 1.85–1.87 (m, 1H), 2.02–2.09 (m, 1H), 2.13–2.18 (m, 1H), 2.23–2.29 (m, 1H), 2.33 (s, 3H, –ArMe), 2.52–2.60 (m, 3H), 3.31–3.38 (m, 1H, –CHAr), 7.09–7.14 (m, 4H, –Ar) 13C NMR (CDCl3, 100 MHz): δ 20.3, 21.0, 29.3, 39.2, 39.5, 47.3, 48.5, 76.7, 77.0, 77.4, 126.8, 129.3, 136.1, 141.4, 211.7 MS (m/z) 203.03 HRMS (ESI) calcd for C14H19O [M + H]+ 203.1436, found 203.1443 [α]D = −16.65 (c 0.4, DCM) The diastereomeric and enantiomeric ratios were determined via HPLC using a chiral column (Daicel Chiralpak IC), n-hexanes
:
IPA = 98
:
2, 0.5 ml min−1, 210 nm, 24.5 min (major), 27.2 min (minor), 28.5 min (minor), 31.1 min (minor).
For 1a:
[α]D = 70.9 (c 0.50, CHCl3) for 76% ee, lit2h [α]D = −74.6 (R) (c 0.5, CHCl3, 80% ee).
3-Methyl-5-(1-naphthalene)cyclohexanone (3e)
Colorless oil. 1H NMR (CDCl3, 400 MHz): δ 1.04 (d, 1H, –CH3, J = 6.4 Hz), 1.94–2.00 (m, 1H), 2.13–2.23 (m, 3H), 2.56–2.62 (m, 1H), 2.74 (d, 2H, J = 6.8 Hz), 4.19–4.52 (m, 1H, –CHAr), 7.28 (d, 1H, –Ar, J = 7.2 Hz), 7.41 (t, 1H, –Ar, J = 7.8 Hz), 7.46–7.54 (m, 2H, –Ar), 7.72 (d, 1H, –Ar, J = 8.0 Hz), 7.85 (d, 1H, –Ar, J = 8.0 Hz), 8.04 (d, 1H, –Ar, J = 8.4 Hz) 13C NMR (CDCl3, 100 MHz): δ 20.6, 29.2, 34.9, 38.6, 46.7, 48.9, 122.9, 123.5, 125.4, 125.6, 126.2, 127.3, 129.2, 131.0, 134.0, 140.1, 212.0 MS (m/z) 237.21 HRMS (ESI) calcd for C17H19O [M + H]+ 239.1436, found 239.1443 [α]D = −19.74 (c 1.0, DCM) The diastereomeric and enantiomeric ratios were determined via HPLC using a chiral column (Daicel Chiralpak IC), n-hexanes
:
IPA = 98
:
2, 0.5 ml min−1, 254 nm, 34.1 min (major), 37.0 min (minor), 40.7 min (minor), 42.9 min (minor).
For 1a:
[α]D = 78.3 (c 0.50, CHCl3) for 84% ee, lit2h [α]D = −74.6 (R) (c 0.5, CHCl3, 80% ee).
3-Methyl-5-(2-naphthalene)cyclohexanone (3f)
Colorless oil. 1H NMR (CDCl3, 400 MHz): δ 1.05 (d, 3H, –CH3, J = 6.8 Hz), 1.91–1.97 (m, 1H), 2.15–2.31 (m, 3H), 2.56–2.78 (m, 3H), 3.52–3.59 (m, –CHAr), 7.36 (dd, 1H, J = 1.6 Hz, 8.4 Hz, –Ar), 7.43–7.49 (m, 2H, –Ar), 7.63 (s, 1H, –Ar), 7.81 (d, 3H, J = 6.8 Hz, –Ar) 13C NMR (CDCl3, 100 MHz): δ 20.4, 29.2, 39.3, 39.7, 47.0, 48.6, 125.2, 125.6, 125.7, 126.2, 127.6, 127.8, 128.3, 132.2, 133.5, 141.8, 211.5 MS (m/z) 239.04 HRMS (ESI) calcd for C17H19O [M + H]+ 239.1436, found 239.1441 [α]D = −5.42 (c 0.7, DCM) The diastereomeric and enantiomeric ratios were determined via HPLC using a chiral column (Daicel Chiralpak IC), n-hexanes
:
IPA = 98
:
2, 0.5 ml min−1, 254 nm, 37.9 min (major), 39.8 min (minor), 45.7 min (minor), 48.6 min (minor).
For 1a:
[α]D = 73.7 (c 0.50, CHCl3) for 79% ee, lit2h [α]D = −74.6 (R) (c 0.5, CHCl3, 80% ee).
3-Methyl-5-(biphenyl-4)cyclohexanone (3g)
White solid. 1H NMR (CDCl3, 400 MHz): δ 1.04 (d, 3H, –CH3, J = 6.8 Hz), 1.87–1.93 (m, 1H), 2.08–2.21 (m, 2H), 2.28–2.32 (m, 1H), 2.55–2.65 (m, 3H), 3.39–3.46 (m, 1H, –CHAr), 7.25–7.35 (m, 3H, –Ar), 7.41–7.45 (m, 2H, –Ar), 7.53–7.58 (m, 4H, –Ar) 13C NMR (CDCl3, 125 MHz): δ 20.4, 29.3, 39.3, 39.4, 47.2, 48.5, 127.0, 127.2, 127.3, 127.4, 128.8, 139.5, 140.8, 143.4, 211.5 MS (m/z) 265.11 HRMS (ESI) calcd for C19H21O [M + H]+ 265.1592, found 254.1581 [α]D = −10.99 (c 0.9, DCM) The diastereomeric and enantiomeric ratios were determined via HPLC using a chiral column (Daicel Chiralpak IC), n-hexanes
:
IPA = 95
:
5, 270 nm, 0.5 ml min−1, 30.6 min (major), 31.5 min (minor), 33.5 min (minor), 34.1 min (minor).
For 1a:
[α]D = 46.6 (c 0.50, CHCl3) for 50% ee, lit2h [α]D = −74.6 (R) (c 0.5, CHCl3, 80% ee).
3-Methyl-5-p-methoxyphenylcyclohexanone (3h)
Colorless oil. 1H NMR (CDCl3, 400 MHz): δ 1.01 (d, 3H, –CH3, J = 6.8 Hz), 1.80–1.86 (m, 1H), 2.00–2.06 (m, 1H), 2.12–2.17 (m, 1H), 2.22–2.29 (m, 1H), 2.51–2.57 (m, 3H), 3.30–3.36 (m, 1H, -CHAr), 3.78 (s, 3H, –ArOMe), 6.85 (d, 2H, –Ar, J = 8.4 Hz), 7.12 (d, 2H, –Ar, J = 8.8 Hz) 13C NMR (CDCl3, 100 MHz): δ 20.3, 29.2, 38.8, 39.6, 47.5, 48.5, 55.3, 113.9, 127.9, 136.5, 158.2, 211.7 MS (m/z) 218.90 HRMS (ESI) calcd for C14H19O2 [M + H]+ 219.1385, found 219.1395 [α]D = −20.00 (c 0.3, DCM) The diastereomeric and enantiomeric ratios were determined via HPLC using a chiral column (Daicel Chiralpak IC), n-hexanes
:
IPA = 90
:
10, 280 nm, 0.5 ml min−1, 24.7 min (major), 27.1 min (minor), 28.8 min (minor), 29.9 min (minor).
For 1a:
[α]D = 78.4 (c 0.50, CHCl3) for 84% ee, lit2h [α]D = −74.6 (R) (c 0.5, CHCl3, 80% ee).
3-Methyl-5-p-fluorophenylcyclohexanone (3i)
Colorless oil. 1H NMR (CDCl3, 400 MHz): δ 1.00 (d, 3H, –CH3, J = 6.9 Hz), 1.83–1.86 (m, 1H), 1.98–2.05 (m, 1H), 2.12–2.17 (m, 1H), 2.20–2.26 (m, 1H), 2.50–2.56 (m, 3H), 3.31–3.38 (m, 1H, –CHAr), 6.96–6.70 (m, 2H, –Ar), 7.13–7.17 (m, 2H, –Ar) 13C NMR (CDCl3, 100 MHz): δ 20.3, 29.2, 38.9, 39.5, 47.3, 48.4, 115.2, 115.5, 128.3, 128.4, 140.0, 140.0, 160.3, 162.7, 211.3 19F NMR (CDCl3, 300 MHz): δ – 116.6 MS (m/z) 207.08 HRMS (ESI) calcd for C13H16OF [M + H]+ 207.1185, found 207.1188 [α]D = −18.04 (c 0.5, DCM) The diastereomeric and enantiomeric ratios were determined via HPLC using a chiral column (Daicel Chiralpak IC), n-hexanes
:
IPA = 99
:
1, 280 nm, 0.5 ml/min, 40.9 min (major), 47.5 min (minor), 49.3 min (minor).
For 1a:
[α]D = 37.3 (c 0.50, CHCl3) for 40% ee, lit2h [α]D = −74.6 (R) (c 0.5, CHCl3, 80% ee).
3-Methyl-5-p-hydroxylphenylcyclohexanone (3j)
Colorless oil. 1H NMR (CDCl3, 400 MHz): δ 1.00 (d, 3H, –CH3, J = 6.8 Hz), 1.79–1.85 (m, 1H), 1.98–2.05 (m, 1H), 2.12–2.25 (m, 2H), 2.51–2.58 (m, 3H), 3.29–3.36 (m, 1H, –CHAr), 5.46 (s, 1H, –OH), 6.78 (d, –Ar, J = 8.4 Hz), 7.05 (d, –Ar, J = 8.4 Hz) 13C NMR (CDCl3, 100 MHz): δ 20.4, 29.2, 38.8, 39.5, 47.4, 48.5, 115.4, 128.1, 136.3, 154.3, 212.6 MS (m/z) 203.00 HRMS (ESI) calcd for C13H17O2 [M + H]+ 205.1229, found 205.1239 [α]D = −12.19 (c 0.4, DCM) The diastereomeric and enantiomeric ratios were determined via HPLC using a chiral column (Daicel Chiralpak IC), n-hexanes
:
IPA = 90
:
10, 280 nm, 0.5 ml min−1, 33.6 (major), 35.6 min (minor), 40.1 min (minor), 45.4 min (minor).
For 1a:
[α]D = 35.4 (c 0.50, CHCl3) for 38% ee, lit2h [α]D = −74.6 (R) (c 0.5, CHCl3, 80% ee).
3-Methyl-4-phenylcyclohexanone (3k)
Colorless oil. 3k was determined to be trans by comparison with previous literature.4c The 1H and 13C NMR spectra are in accordance with similar transformations in literature.4c1H NMR (CDCl3, 500 MHz): δ 0.78 (d, 3H, –CH3, 6.5 Hz), 1.50–1.59 (m, 2H), 2.09 (m, 1H), 2.13–2.18 (m, 1H), 2.45–2.58 (m, 5H), 7.17–7.27 (m, 3H, –Ar), 7.32–7.35 (m, 2H, –Ar) 13C NMR (CDCl3, 100 MHz): δ 19.3, 34.6, 36.8, 14.4, 49.2, 52.1, 126.7, 127.2, 129.8, 143.6, 211.0 MS (m/z) 189.03 HRMS (ESI) calcd for C13H17O [M + H]+ 189.1279, found 189.1282 [α]D = 36.76 (c 0.1, DCM) The diastereomeric and enantiomeric ratios were determined via HPLC using a chiral column (Daicel Chiralpak IC), n-hexanes
:
IPA = 95
:
5, 210 nm, 0.5 ml min−1, 25.5 (major), 27.0 (minor).
For 1b:
[α]D = 54.9 (c 0.60, CHCl3) for 44% ee, lit12a [α]D = −74.6 (S) (c 0.6, CHCl3, 93% ee).
3-Ethyl-5-phenylcyclohexanone (3l)
Colorless oil. 3l was determined to be trans by comparison with previous literature.4a The 1H and 13C NMR spectra are in accordance with similar transformations in literature.4a1H NMR (CDCl3, 400 MHz): δ 0.88–0.91 (m, 3H), 1.36–1.40 (m, 2H), 1.93–2.08 (m, 3H), 2.21–2.26 (m, 1H), 2.53–2.62 (m, 3H), 3.31–3.33 (m, 1H, –CHAr), 7.20–7.24 (m, 3H, –Ar), 7.30–7.34 (m, 2H, –Ar) 13C NMR (CDCl3, 100 MHz): δ 11.6, 27.0, 36.1, 37.0, 39.5, 46.5, 47.4, 126.5, 126.9, 128.6, 144.4, 211.7 MS (m/z) 203.07 HRMS (ESI) calcd for C14H19O [M + H]+ 203.1436, found 203.1433 [α]D = −19.39 (c 0.1, DCM) The diastereomeric and enantiomeric ratios were determined via HPLC using a chiral column (Daicel Chiralpak IC), n-hexanes
:
IPA = 98
:
2, 210 nm, 0.5 ml min−1, 25.3 min (major), 28.2 min (minor), 29.4 min (minor), 30.8 min (minor).
For 1c:
[α]D = 28.4 (c 0.50, CHCl3) for 62% ee, lit2h [α]D = −43.1 (R) (c 0.6, CHCl3, 94% ee).
3-Propyl-5-phenylcyclohexanone (3m)
Colorless oil. 1H NMR (CDCl3, 400 MHz): δ 0.85–0.88 (m, 3H), 1.30–1.31 (m, 4H), 1.88–1.94 (m, 1H), 2.00–2.10 (m, 2H), 2.19–2.24 (m, 1H), 2.51–2.60 (m, 3H), 3.27–3.34 (m, 1H, –CHAr), 7.19–7.25 (m, 3H, –Ar), 7.29–7.33 (m, 2H, –Ar) 13C NMR (CDCl3, 100 MHz): δ 14.0, 20.2, 34.1, 36.3, 37.3, 39.5, 46.7, 47.5, 126.5, 126.9, 128.6, 144.4, 211.7 MS (m/z) 217.07 HRMS (ESI) calcd for C15H21O [M + H]+ 217.1592, found 217.1600 [α]D = 2.04 (c 0.1, DCM) The diastereomeric and enantiomeric ratios were determined via HPLC using a chiral column (Daicel Chiralpak IC), n-hexanes
:
IPA = 98
:
2, 210 nm, 0.5 ml min−1, 21.4 min (major), 24.4 min (minor), 26.3 min (minor), 28.4 min (minor).
3-Butyl-5-phenylcyclohexanone (3n)
Colorless oil. 3n was determined to be trans by comparison with previous literature.12b The 1H and 13C NMR spectra are in accordance with similar transformations in literature.12b1H NMR 0.86–0.89 (m, 3H), 1.26–1.34 (m, 6H), 1.92–1.96 (m, 1H), 2.02–2.08 (m, 2H), 2.21–2.26 (m, 1H), 2.53–2.61 (m, 3H), 3.28–3.35 (m, 1H, –CHAr), 7.20–7.24 (m, 3H, –Ar), 7.30–7.34 (m, 2H, –Ar) 13C NMR (CDCl3, 100 MHz): δ 14.0, 14.1, 22.7, 22.7, 29.3, 31.6, 33.8, 34.4, 37.3, 39.5, 46.8, 47.5, 126.5, 126.9, 128.6, 144.4, 211.7 MS (m/z) 231.09 HRMS (ESI) calcd for C16H23O [M + H]+ 231.1749, found 231.1756 [α]D = −1.60 (c 0.5, DCM) The diastereomeric and enantiomeric ratios were determined via HPLC using a chiral column (Daicel Chiralpak IC), n-hexanes
:
IPA = 98
:
2, 210 nm, 0.5 ml min−1, 20.0 min (major), 22.7 min (minor), 25.0 min (minor), 27.1 min (minor).
For 1e:
[α]D = 24.4 (c 0.50, CHCl3) for 50% ee, lit2h [α]D = −44.9 (R) (c 0.5, CHCl3, 92% ee).
3-Ethyl-5-p-methoxyphenylcyclohexanone (3o)
Colorless oil. 1H NMR (CDCl3, 400 MHz): δ 0.89 (t, 3H, J = 7.4 Hz), 1.33–1.40 (m, 2H), 1.89–2.04 (m, 3H), 2.19–2.24 (m, 1H), 2.51–2.58 (m, 3H), 3.24–3.31 (m, 1H, –CHAr), 3.789 (s, 3H, –ArOMe), 6.84–6.86 (m, 2H, –Ar), 7.11–7.14 (m, 2H, –Ar) 13C NMR (CDCl3, 100 MHz): δ 11.6, 27.1, 36.0, 37.2, 38.7, 46.5, 47.7, 55.3, 114.0, 127.8, 136.5, 158.2, 211.8 MS (m/z) 233.02 HRMS (ESI) calcd for C15H21O2 [M + H]+ 233.1542, found 233.1545 [α]D = −13.15 (c 0.5, DCM) The diastereomeric and enantiomeric ratios were determined via HPLC using a chiral column (Daicel Chiralpak IC), n-hexanes
:
IPA = 95
:
5, 280 nm, 0.5 ml min−1, 30.0 min (major), 33.5 min (minor), 36.6 (minor), 37.9 (minor).
For 1c:
[α]D = 28.5 (c 0.50, CHCl3) for 62% ee, lit2h [α]D = −43.1 (R) (c 0.6, CHCl3, 94% ee).
3-Propyl-5-p-methoxyphenylcyclohexanone (3p)
Colorless oil. 1H NMR (CDCl3, 500 MHz): δ 0.88 (m, 3H), 1.31 (m, 4H), 1.87–1.92 (m, 1H), 1.98–2.04 (m, 1H), 2.07–2.08 (m, 1H), 2.18–2.23 (m, 1H), 2.51–2.58 (m, 3H), 3.25–3.30 (m, 1H, –CHAr), 3.79 (s, 3H, –ArOMe), 6.85–6.86 (m, 2H, –Ar), 7.12–7.14 (m, 2H, –Ar) 13C NMR: (CDCl3, 100 MHz): δ 14.0, 20.2, 34.1, 36.4, 37.5, 38.8, 46.7, 47.8, 55.3, 114.0, 127.8, 136.5, 158.2, 211.8. MS (m/z) 247.01 HRMS (ESI) calcd for C16H23O2 [M + H]+ 247.1698, found 247.1694 [α]D = −5.80 (c 0.3, DCM) The diastereomeric and enantiomeric ratios were determined via HPLC using a chiral column (Daicel Chiralpak IC), n-hexanes
:
IPA = 95
:
5, 280 nm, 0.5 ml min−1, 26.8 min (major), 30.7 min (minor), 34.7 min (minor), 35.6 min (minor).
3-Butyl-5-p-methoxyphenylcyclohexanone (3q)
Colorless oil. 1H NMR (CDCl3, 500 MHz): δ 0.86–0.89 (m, 3H), 1.27–1.33 (m, 6H), 1.89–1.92 (m, 1H), 1.98–2.06 (m, 2H), 2.19–2.24 (m, 1H), 2.51–2.57 (m, 3H), 3.28–3.29 (m, 1H, –CHAr), 3.79 (s, 3H, –ArOMe), 6.85–6.86 (m, 2H, –Ar), 7.12–7.14 (m, 2H, –Ar) 13C NMR (CDCl3, 125 MHz): δ 14.0, 22.7, 29.3, 33.9, 34.4, 37.5, 38.8, 46.8, 47.8, 55.3, 114.0, 127.8, 136.5, 158.2, 211.8. MS (m/z) 261.01 HRMS (ESI) calcd for C17H25O2 [M + H]+ 261.1855, found 261.1849 [α]D = −1.00 (c 0.5, DCM) The diastereomeric and enantiomeric ratios were determined via HPLC using a chiral column (Daicel Chiralpak IC), n-hexanes
:
IPA = 95
:
5, 280 nm, 0.5 ml min−1, 24.0 min (major), 27.1 min (minor), 33.8 min (minor), 34.4 min (minor).
For 1e:
[α]D = 22.9 (c 0.50, CHCl3) for 47% ee, lit2h [α]D = −44.9 (R) (c 0.5, CHCl3, 92% ee).
Acknowledgements
We gratefully thank the Ministry of Education, Singapore (MOE AcRF Tier 1 RG 12/12) for financial support and Nanyang Technological University for a research scholarship to Kennard. We also thank Nanyang Technological University for a URECA programme offered to Sadeer.
Notes and references
-
(a) G. P. J. Hareau, M. Koiwa, S. Hikichi and F. Sato, J. Am. Chem. Soc., 1999, 121, 3640–3650 CrossRef CAS;
(b) K. Mori, Tetrahedron, 1989, 45, 3233–3298 CrossRef CAS;
(c) R. B. Jagt, R. Imbos, R. Naasz, A. J. Minnaard and B. L. Feringa, Isr. J. Chem., 2001, 41, 221–230 CrossRef CAS;
(d)
E. Sorenson and K. Nicolauo, Classics in Total Synthesis, VCH, NY, 1996 Search PubMed;
(e)
K. Mori and J. ApSimon, The Total Synthesis of Natural Products, 1992, p. 9 Search PubMed;
(f) B. Koutek, L. Streinz and M. Romaňuk, Collect. Czech. Chem. Comm., 1998, 63, 899–954 CrossRef CAS;
(g) Y.-B. Lan, H. Zhao, Z.-M. Liu, G.-G. Liu, J.-C. Tao and X.-W. Wang, Org. Lett., 2011, 13, 4866–4869 CrossRef CAS PubMed.
-
(a)
T.-L. Ho, Enantioselective Synthesis: Natural Products from Chiral Terpenes, Wiley, NY, 1992 Search PubMed;
(b) A. Srikrishna, S. J. Gharpure and P. Praveen Kumar, Tetrahedron Lett., 2000, 41, 3177–3180 CrossRef CAS;
(c) T. M. Meulemans and G. A. Stork, J. Org. Chem., 1999, 64, 9178–9188 CrossRef CAS;
(d) H. W. Lee, S. K. Ji, I.-Y. C. Lee and J. H. Lee, J. Org. Chem., 1996, 61, 2542–2543 CrossRef CAS;
(e) K. Ogasawara, Pure Appl. Chem., 1994, 66, 2119–2122 CrossRef CAS;
(f) M. Koiwa, G. P. J. Hareau, D. Morizono and F. Sato, Tetrahedron Lett., 1999, 40, 4199–4202 CrossRef CAS;
(g) T. Hanazawa, S. Okamoto and F. Sato, Tetrahedron Lett., 2001, 42, 5455–5457 CrossRef CAS;
(h) A. Carlone, M. Marigo, C. North, A. Landa and K. A. Jørgensen, Chem. Commun., 2006, 4928–4930 RSC.
-
(a)
K. Drauz and H. Waldmann, Enzyme Catalysis in Organic Synthesis, A Comprehensive Handbook, VCH, Weiheim, 1st edn, 1995, vol. 1 Search PubMed;
(b) K. Ogasawara, J. Synth. Org. Chem., 1999, 57, 957–969 CrossRef CAS;
(c) S. A. Kozmin and V. H. Rawal, J. Am. Chem. Soc., 1997, 119, 7165–7166 CrossRef CAS;
(d) F.-Y. Zhang and E. Corey, Org. Lett., 2000, 2(8), 1097–1100 CrossRef CAS;
(e) A. N. Weissfloch and R. J. Kazlauskas, J. Org. Chem., 1995, 60, 6959–6969 CrossRef CAS;
(f) S. Hashiguchi, A. Fujii, K. J. Haack, K. Matsumura, T. Ikariya and R. Noyori, Angew. Chem., Int. Ed. Engl., 1997, 36, 288–290 CrossRef CAS PubMed;
(g) G. Sarakinos and E. Corey, Org. Lett., 1999, 1, 1741–1744 CrossRef CAS.
- For resolutions of racemic 5-substituted cyclohexenone, see:
(a) T. Soeta, K. Selim, M. Kuriyama and K. Tomioka, Tetrahedron, 2007, 63, 6573–6576 CrossRef CAS PubMed;
(b) R. Naasz, L. A. Arnold, A. J. Minnaard and B. L. Feringa, Angew. Chem., Int. Ed., 2001, 113, 953–956 CrossRef;
(c) A. Kolb, S. Hirner, K. Harms and P. v. Zezschwitz, Org. Lett., 2012, 14, 4978–1981 CrossRef PubMed;
(d) R. Imbos, A. J. Minnaard and B. L. Feringa, Tetrahedron, 2001, 57, 2485–2489 CrossRef CAS;
(e) Q. Chen, M. Kuriyama, T. Soeta, X. Hao, K. Yamada and K. Tomioka, Org. Lett., 2005, 7, 4439–4441 CrossRef CAS PubMed;
(f) G. Hareau-Vittini, S. Hikicki and F. Sato, Angew. Chem., Int. Ed., 1998, 37, 2099–2101 CrossRef CAS. For resolutions of racemic 6-substituted cyclohexenones, see:
(g) K. Selim, T. Soeta, K. Yamada and K. Tomoika, Chem. – Asian J., 2008, 3, 342–350 CrossRef CAS PubMed;
(h) L. M. Urbaneja, A. Alexakis and N. Krause, Tetrahedron Lett., 2002, 43, 7887–7890 CrossRef;
(i) Q. Chen, T. Soeta, M. Kuriyama, K. Yamada and K. Tomoika, Adv. Synth. Catal., 2006, 348, 2604–2608 CrossRef CAS PubMed;
(j) L. M. Urbaneja and N. Krause, Tetrahedron: Asymmetry, 2006, 17, 494–496 CrossRef PubMed.
-
(a) I. P. Beletskaya and A. V. Cheprakov, Chem. Rev., 2000, 100, 3009 CrossRef CAS PubMed;
(b) M. L. Dounay and L. E. Overman, Chem. Rev., 2003, 103, 2945 CrossRef PubMed;
(c) L. F. Tietze, H. Ila and H. P. Bell, Chem. Rev., 2004, 104, 3453 CrossRef CAS PubMed.
-
(a) K. Chen, H. J. Chen, J. Wong, J. Yang and S. A. Pullarkat, ChemCatChem, 2013, 5, 3882–3888 CrossRef CAS PubMed;
(b) K. Chen, Y. Li and S. A. Pullarkat, Adv. Synth. Catal., 2012, 354, 83–87 CrossRef CAS PubMed.
-
(a) K. Chen and S. A. Pullarkat, Org. Biomol. Chem., 2012, 10, 6600–6606 RSC;
(b) Y. Huang, S. A. Pullarkat, Y. Li and P.-H. Leung, Chem. Commun., 2010, 46, 6950–6952 RSC;
(c) Y. Huang, R. T. J. Chew, S. A. Pullarkat, Y. Li and P.-H. Leung, J. Org. Chem., 2012, 77, 6849–6854 CrossRef CAS PubMed;
(d) X. Chang, J. H. Gan, F. Hennersdorf, Y. Li, S. A. Pullarkat and P.-H. Leung, Organometallics, 2012, 31, 3022–3026 CrossRef;
(e) Y. Huang, R. T. J. Chew, Y. Li, S. A. Pullarkat and P.-H. Leung, Org. Lett., 2011, 13, 5862–5865 CrossRef CAS PubMed;
(f) Y. Huang, S. A. Pullarkat, S. Teong, R. T. J. Chew, Y. Li and P.-H. Leung, Organometallics, 2012, 31, 4871–4875 CrossRef CAS.
- J. Wong, K. Gan, H. J. Chen and S. A. Pullarkat, Adv. Synth. Catal., 2014, 356, 3391–3400 CrossRef CAS PubMed.
- K. Gan, A. Sadeer, X. Chang, Y. Li and S. A. Pullarkat, Organometallics, 2014, 33, 5074–5076 CrossRef CAS.
- Enantioselectivity corresponding to major trans product.
-
(a) J. C. Holder, L. Zou, A. N. Marziale, P. Liu, Y. Lan, M. Gatti, K. Kikushima, K. N. Houk and B. M. Stolz, J. Am. Chem. Soc., 2013, 135, 14996–15007 CrossRef CAS PubMed;
(b) A. R. Kapdi, G. Dhangar, J. L. Serrano, J. Pérez, L. Garcia and I. J. S. Fairlamb, Chem. Commun., 2014, 50, 9859–9861 RSC;
(c) Y. Suzuma, S. Hayashi, T. Yamamoto, Y. Oe, T. Ohta and Y. Ito, Tetrahedron: Asymmetry, 2009, 20, 2751–2758 CrossRef CAS PubMed;
(d) T. Nishikata, Y. Yamamoto and N. Miyaura, Angew. Chem., Int. Ed., 2003, 42, 2768–2770 CrossRef CAS PubMed.
-
(a) J. Evarts, E. Torres and P. L. Fuchs, J. Am. Chem. Soc., 2002, 124, 11093–11101 CrossRef CAS PubMed;
(b) B. H. Lipshutz, S. Huang, W. W.-Y. Leong, G. Zhong and N. A. Isley, J. Am. Chem. Soc., 2012, 134, 19985–19988 CrossRef CAS PubMed.
Footnote |
† Electronic supplementary information (ESI) available. See DOI: 10.1039/c5qo00169b |
|
This journal is © the Partner Organisations 2015 |
Click here to see how this site uses Cookies. View our privacy policy here.