DOI:
10.1039/C5QO00154D
(Tutorial Account)
Org. Chem. Front., 2015,
2, 990-994
The remarkable role of solvent in reaction mechanism studies by electrospray mass spectrometry
Received
12th May 2015
, Accepted 11th June 2015
First published on 12th June 2015
Abstract
The solvent requirements for an organic reaction are different from those of electrospray mass spectrometry (ESI-MS) analysis; therefore, some reaction solutions in non-ESI friendly solvents cannot be directly studied using ESI-MS. This mini account discusses some of our recent contributions in solving the challenging problems in the characterization of reactive intermediates in reaction solutions in non-ESI friendly solvents by using ESI-based mass spectrometry.
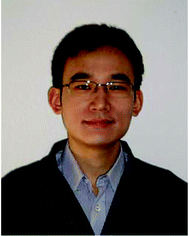 Hao-Yang Wang | Hao-Yang Wang was born in 1978 in Henan Province, China. He received his B.S. degree in 2000 from China Pharmaceutical University and completed his PhD in 2006 at Shanghai Institute of Organic Chemistry (SIOC). After two years of postdoctoral research at University Oldenburg in Germany, he got his associate professor position at SIOC in 2008. His research interests focus on developing new soft ionization mass spectrometry technologies to investigate transient reactive intermediates for reaction mechanism studies. |
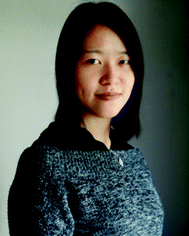 Jun-Ting Zhang | Jun-Ting Zhang was born in 1990 in Anhui Province, China. She received her B.S. degree in 2011 from Anhui Normal University, and now is a PhD student at Shanghai Institute of Organic Chemistry (SIOC) under the supervision of Prof. Yin-Long Guo. Her research interests are mainly on developing new ionization mass spectrometry technologies to study reaction mechanisms. |
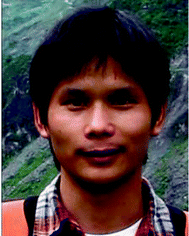 Shu-Sheng Zhang | Shu-Sheng Zhang was born in 1983 in Hubei Province, China. He received his B.S. degree in 2005 from Wuhan University, and a PhD in 2012 from Shanghai Institute of Organic Chemistry (SIOC) under the supervision of Prof. Guo-Qiang Lin. Subsequently, he joined the research group of Prof. Yin-Long Guo (SIOC). His research interests include the development of asymmetric catalysis and mechanistic studies using mass spectrometry. |
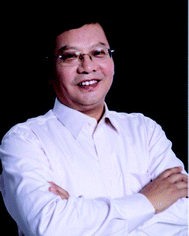 Yin-Long Guo | Yin-Long Guo was born in 1962 in Henan Province, China. He received his B.S. degree in 1984 from China Pharmaceutical University and completed his PhD in 1993 at China Pharmaceutical University. He joined the faculty of SIOC from 1997. He is currently a full professor of the analytical chemistry laboratory. His research interests focus on the development of novel and efficient mass spectrometry technologies for solving challenging problems in analytical chemistry, organic chemistry and biological research. |
Introduction
As a valuable tool for weighing ions, mass spectrometry has important and wide applications in scientific research.1 The excellent soft ionization mode of electrospray ionization and subsequent tandem mass spectrometry(ESI-MS/MS) make it an ideal method for reaction mechanism studies by monitoring reactants and products, as well as investigating reactive intermediates at low concentration levels and the related reactive species involved in the reaction.2 Modern electrospray mass spectrometry, with characteristics of sensitivity, speed, specificity and stoichiometry, provides more insightful information for exploring and understanding the mechanism of chemical reactions. The key issues of reaction mechanism studies by mass spectrometry are the interception, identification and characterization of the reactive intermediates. Such a task is always a big challenge because the highly reactive species normally have short life-times; their concentrations are typically low, and sometimes they are not stable and could easily transform into the resting states and the decomposed complexes.
Screening and selection of a suitable solvent is not only important for optimization of the reaction conditions to get high yields and good selectivity, but is also critical for the successful characterization of the reactive intermediates by soft-ionization mass spectrometry. For organic reactions, the change of solvent could have a big influence on the reaction process and might even change the reaction pathways. Meanwhile, for ESI-MS analysis, both the ionic species formation step and the following step of transferring the ionic species to the gas phase environment are seriously dependent on the properties of the solvent. How to make the reaction solution more compatible with the following ESI-MS analysis should be taken into consideration. The solvent applied for the ESI-MS analysis has significant influence on the aggregation effect of ions, the solubility and the ionization efficiency of the target species in the ionization process.3 Some other factors, such as intermediate stability, reaction time scale and ESI-MS detection sensitivity in different solvent systems should also be considered and tested. Therefore, scientists like to use the word “fishing” to describe how to intercept and study the intermediates in the reaction solution by using ESI-MS. Undoubtedly, the solvents used in chemical reactions and ESI-MS studies play important roles in sustaining the “fish” (the reactive intermediate) and catching the “fish”.4
Nowadays, ESI-MS analysis methods based on on-line rapid mixing and fast droplet contact provide more options for the flexible application of assistant solvents for transient reactive species detection in ESI-MS analysis. We are increasingly aware of the importance of solvent assistance strategies in reaction mechanism studies by using ESI-based mass spectrometry. Here, we briefly summarize the solvent assistance methods applied in our mechanistic studies by ESI-MS: from the traditional snapshot ESI-MS monitoring by interval sampling, to ESI-MS methods coupled with a micro-reactor device and finally up-to-date ESI-based ambient ionization methods, such as the solvent-assisted electrospray ionization (SAESI, Fig. 1) method developed recently by our group. In such an ionization method, the sample solution in “non-ESI-friendly” solvents is well ionized with an assistant polar solvent with two separated sprayers meeting at the spray end tip (Fig. 1).5 We hope this short account could help scientists in designing their “fishing” methods for reaction mechanism studies by applying ESI-based mass spectrometry methods.
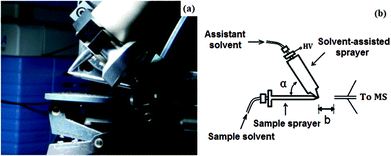 |
| Fig. 1 (a) Photographic image of solvent-assisted electrospray ionization (SAESI) apparatus. (b) Schematic representation of the SAESI-MS method. | |
Results and discussion
Snapshot monitoring by interval ESI-MS analysis
When the solvent of a homogeneous organic reaction is “ESI-friendly” and suitable for ESI-MS analysis, and the reaction time scale is compatible for monitoring the reaction solution by interval sampling, the reaction solution can be directly diluted, injected and studied using ESI-MS or ESI-MS/MS. As a pioneer, Canary studied the catalytic intermediates in the Suzuki-reaction by electrospray mass spectrometry.6 By snapshot monitoring of the diluted reaction solution, we have used ESI-MS/MS to intercept and characterize the reactive organometallic intermediates in the Pd-catalyzed addition reaction of organoboronic acids to allenes in the presence of AcOH7 and the Pd(0)-catalyzed three-component tandem double addition–cyclization.8 In a later study, the mechanism was confirmed to involve carbopalladation with 2-(2,3-allenyl)malonate, yielding the π-allyl-Pd-intermediate.8 Furthermore, the reaction mechanisms of Pd-catalyzed cycloisomerization of trifluoromethyl-substituted enynols and the selenohydroxylation reaction of 1,2-allenyl phosphine oxides with PhSeCl were also studied using the ESI-MS/MS method.9 Recently, ESI-MS methods have been successfully applied in our studies of the Pd(II)–Pd(IV) catalytic cycle in related reactions.10 Xu studied the Pd-catalyzed acetoxylation and methoxylation of carbon–hydrogen bonds with PhI(OAc)2 as an oxidant and the reaction mechanisms were proposed to be Pd(II)–Pd(IV) or Pd(II)–Pd(III) redox cycles depending on the sigma-donor ligand and the solvent polarity.11 Besides the metal-catalyzed reactions, we also used ESI-MS/MS methods to monitor the diluted homogeneous reaction solutions of Selectfluor with triphenylethylene.12 The transient radical cationic intermediates can be successfully intercepted and studied by ESI-MS.
Studying transient species only existing in solution
For some reactive species generated in situ, such as hypervalent iodine complexes, which are hard to separate and crystallize, ESI-MS/MS is a valuable tool to explore their possible structures and study their intrinsic reactivity. Recently, we studied the reactive transient α-λ3-iodanyl-acetophenone complex in the α-acetoxylation reaction of acetophenone, λ3-phenyl(trifluoromethyl)iodonium and λ3-2,5-xylylfluoroiodonium using ESI-MS/MS.13 Because the difluoroiodoarene compounds (Aryl-IF2) are very hygroscopic and extremely water-sensitive, the anhydrous bromobenzene was found to be a better solvent than acetonitrile for such ESI-MS analysis, showing less signals of hydrolyzed products. We also found that the benzene and toluene solutions could not give a stable spray and good signals in ESI-MS due to their low dielectric constants. Anhydrous bromobenzene and fluorobenzene are good substitute solvents in studying air/water-sensitive reactions, and their reaction solutions could give more stable signals in ESI-MS analysis.
If the addition of extra assistant solvent has no significant influence on the reaction or does not cause the decomposition of intermediates, mixing the reaction solution in non-ESI-friendly solvent with a suitable diluting ESI-friendly solvent would give better ESI-MS analysis results. By diluting the toluene reaction solution with acetonitrile directly, the reaction mechanism of the Pd–diene-catalyzed asymmetric Suzuki–Miyaura reaction was studied.14 The key Pd-intermediates with the diene ligand corresponding to each catalytic step were captured and characterized by ESI-MS/MS. In the gas phase, the Cs adduct ions of the Pd–diene-intermediates in the transmetalation step could transform into product, induced by collision in the MS/MS, which was consistent with the product-yielding process and supported their catalytic activity.
ESI-based micro-reactor method
The snapshot monitoring method would no longer meet the requirements for the interception and characterization of short-lived and highly reactive intermediates in certain fast reactions and at the beginning stage of the reaction. By mixing two different reactant solutions in the mixing tee coupled directly to the ESI-MS ion source, the micro-reactor device allows the investigation of the reaction in a reaction time window of approximately 1–30 seconds by using a common injection speed.15 Such a device is an ideal method for online mixing and diluting of reaction solutions with the same or different solvents. In situ exchange of neutral PCy3 by phosphine with a cationic tag in such a device allowed the direct characterization of the active 14-electron Ru-intermediates in the fast Ru–carbene catalyzed metathesis reaction solution in dichlormethane.16 ESI-MS combined with a micro-reactor or similar device provides a more detailed picture for the mechanistic studies at the beginning stage of a reaction, as well as for rapid reactions.
When using different solvents in the ESI-based micro-reactor device, scientists might get some surprising results. Mixing solutions of 1 equivalent of the Grubbs-catalyst dissolved in dichlormethane with 5 equivalents of alkali metal chloride salts dissolved in methanol using a micro-reactor could simultaneously result in intense signals of the stable alkali metal cationized Ru–carbene complexes.17 Based on this method, many kinds of intermediates in different olefin metathesis reactions were intercepted and the reactivity of these alkali metal cationized Ru–carbene intermediates was studied using ion-molecule reaction or MS/MS.17 We also took advantage of the micro-reactor method to investigate the transient intermediates in the acetonitrile-assisted fast decomposition reactions of 1st generation Grubbs catalyst18 and the rapid cyclopropenium-activated chlorination reaction of alcohols on the reaction time scale of several minutes.19 Abliz and Ding successfully used the cold-spray ionization method coupled with a micro-reactor device to monitor the dynamic structures of labile noncovalent titanium complexes in solution.20 The pressurized sample infusion (PSI) system developed by the McIndoe group is also a practical device to monitor the reaction and study some oxygen/water sensitive reactions by ESI-MS.21
Solvent-assisted electrospray ionization method
The most common situations chemists meet during reaction mechanism studies using ESI-MS are that the optimal conditions for chemical reactions are in low/non-polar solvents, such as dichloromethane, hexane, benzene or toluene, which are incompatible with typical ESI-MS. Dilution with polar solvents or ionic liquids directly or application through an on-line mixing device is a general method to solve the problems. However, sometimes these methods do not work because such operations might change and disturb the experimental conditions and finally compromise the intermediate detection by ESI-MS. Nowadays, some new ESI-based ambient MS methods have been developed and reported for the analysis of a sample solution in “non-ESI-friendly” solvents. The outstanding point of SAESI-MS is that a very short time scale in the mixing event at the spray tip during SAESI-MS ionization would cause little interference with the original state of the reaction solution.
The extractive electrospray ionization mass spectrometry (EESI-MS) developed by Chen and Cooks has some similarities with the SAESI-MS method and EESI-MS is also an important method for studying the transient reactive intermediates of some fast reactions on the millisecond timescale.22 Unlike the experimental setup with two separated sprayers in EESI-MS, the dual sprayers contact each other at the spray tip with a 30°–75° angle in SAESI-MS. Based on some experiments, the ionization process in SAESI was suggested to occur in the Taylor cone before nebulization of the sample solution, which is different from the EESI-MS process. The sample introduction is independent from the ionization process in SAESI, which allows the online-monitoring reaction solution in a less polar solvent, such as the cycloisomerization of phenyl propargyl ether catalyzed by Ph3PAuNTf2 and the Morita–Baylis–Hillman (MBH) reaction in dichloromethane. Most of the intermediates can be ionized and detected in their original states due to the extremely short mixing time with the assistant solvent and some chemical reagents can be added to the assistant solvent to enhance the ionization efficiency of the target analytes.5 SAESI is also an outstanding MS interface with high tolerance to low/non-polar compounds or complexes, improving the ionization efficiency and decreasing the matrix suppression effect. SAESI can be applied to analyze polymers in GPC eluents and chiral drugs separated by normal phase liquid chromatography and to perform online H/D exchange. By developing and using such a method, we can now perform ESI-MS studies on complicated sample solutions that were incompatible with ESI-MS analysis previously.
Experimental
General cautions in studying reaction solutions using ESI-MS
Some operations are recommended herein for the reaction mechanism studies using ESI-MS: (1) using high quality solvent and performing control runs of the solvents to check the solvent purity and the background signals; (2) in the analysis of water or air sensitive compounds/complexes, the solvent should be dried and bubbled with argon and acetonitrile could be changed to bromobenzene or fluorobenzene; (3) concentrated solutions of metal-catalyzed reactions must first be filtered using filter membranes (pore size of 0.45 μm with organic solvent tolerance) to get rid of tiny or invisible particles in the reaction solution, which could cause a terrible jam in the spray capillary in the ESI ion source; (4) after the ESI-MS measurements, the ion source has to be cleaned immediately to remove the contamination of residuals from the reaction solution.
Micro-reactor device
An aliquot of solution (a) was mixed with an aliquot of solution (b) in the same or different solvents by using two independent syringes into a mixing tee coupled directly to the ESI-MS ion source. The injection speed of both solutions and the dead volume of the mixing tee and pipe line to the ESI-MS ion source determined the time scale of such a device for studying the reaction.
SAESI device
The SAESI device includes two independent sprayers: a sample solution sprayer and a solvent-assisted sprayer with a high-voltage power supply. The dual sprayers contact with each other at the spray tip at a 30°–75°angle (Fig. 1). Such a design allows the charged solvent droplets and the sample solution droplets to meet simultaneously at the tips of the spray needles to form a continuous and stable spray plume. Normally, the polar solvent was selected and used as the assistant solution based on the experimental results.
Conclusions
In this short account, we preliminarily discussed the solvent assistance strategies and technologies developed recently and their applications in “fishing” the reactive intermediates from complicated reaction solutions. In particular, the SAESI-MS method was highly recommended due to its simplicity, flexibility and good performance for the analysis of a reaction solution in a non-ESI friendly solvent system. These advantages make SAESI-MS a prospective strategy in reaction mechanism studies using ESI-based mass spectrometry.
Acknowledgements
We are grateful for financial support from the National Natural Science Foundation of China (Grant No. 21172250, 21475145 and 21472228) and Youth Innovation Promotion Association CAS.
Notes and references
-
(a) J. B. Fenn, M. Mann, C. K. Meng, S. F. Wong and C. M. Whitehouse, Science, 1989, 246, 64–71 CAS;
(b)
L. S. Santos, Reactive Intermediates: MS Investigations in Solution, Weinheim, Wiley-VCH, 2009 Search PubMed;
(c) P. Chen, Angew. Chem., Int. Ed., 2003, 42, 2832–2847 CrossRef CAS PubMed;
(d) L. S. Santos, Eur. J. Org. Chem., 2008, 235–253 CrossRef CAS PubMed;
(e) Z. X. Zhao, H. Y. Wang and Y. L. Guo, Curr. Org. Chem., 2011, 15, 3734–3749 CrossRef CAS;
(f) L. P. E. Yunker, R. L. Stoddard and J. S. McIndoe, J. Mass Spectrom., 2014, 49, 1–8 CrossRef CAS PubMed.
-
(a) H. L. Bao, J. Zhou, Z. Wang, Y. L. Guo, T. P. You and K. L. Ding, J. Am. Chem. Soc., 2008, 130, 10116–10127 CrossRef CAS PubMed;
(b) B. Gao, Y. H. Wen, Z. G. Yang, X. Huang, X. H. Liu and X. M. Feng, Adv. Synth. Catal., 2008, 350, 385–390 CrossRef CAS PubMed;
(c) S. F. Qiu, T. Xu, J. Zhou, Y. L. Guo and G. S. Liu, J. Am. Chem. Soc., 2010, 132, 2856–2857 CrossRef CAS PubMed;
(d) H. Z. Sun, Y. F. Chai, L. Wang, K. Z. Jiang and Y. J. Pan, Organometallics, 2013, 32, 3385–3390 CrossRef CAS;
(e) Y. F. Chai, S. S. Shen, G. F. Weng and Y. J. Pan, Chem. Commun., 2014, 50, 11668–11671 RSC;
(f) X. Yan, E. Sokol, X. Li, G. T. Li, S. Q. Xu and R. G. Cooks, Angew. Chem., Int. Ed., 2014, 53, 5931–5935 CrossRef CAS PubMed.
-
(a) J. Pape, K. L. Vikse, E. Janusson, N. Taylor and J. S. McIndoe, Int. J. Mass Spectrom., 2014, 373, 66–71 CrossRef CAS PubMed;
(b) A. Badu-Tawiah, C. Bland, D. I. Campbell and R. G. Cooks, J. Am. Soc. Mass Spectrom., 2010, 21, 572–579 CrossRef CAS PubMed;
(c) A. Kruve, K. Kaupmees, J. Liigand and L. Leito, Anal. Chem., 2014, 86, 4822–4830 CrossRef CAS PubMed;
(d) B. A. Huffman, M. L. Poltash and C. A. Hughey, Anal. Chem., 2012, 84, 9942–9950 CrossRef CAS PubMed.
-
(a) C. Adlhart and P. Chen, Helv. Chim. Acta, 2000, 83, 2192–2196 CrossRef CAS;
(b) F. Coelho and M. N. Eberlin, Angew. Chem., Int. Ed., 2011, 50, 5261–5263 CrossRef CAS PubMed.
- J. T. Zhang, H. Y. Wang, W. Zhu, T. T. Cai and Y. L. Guo, Anal. Chem., 2014, 86, 8937–8942 CrossRef CAS PubMed.
- A. O. Aliprantis and J. W. Canary, J. Am. Chem. Soc., 1994, 116, 6985–6986 CrossRef CAS.
- R. Qian, H. Guo, Y. X. Liao, Y. L. Guo and S. M. Ma, Angew. Chem., Int. Ed., 2005, 44, 4771–4774 CrossRef CAS PubMed.
- H. Guo, R. Qian, Y. X. Liao, S. M. Ma and Y. L. Guo, J. Am. Chem. Soc., 2005, 127, 13060–13064 CrossRef CAS PubMed.
-
(a) R. Qian, Y. L. Guo and L. Lu, Chin. J. Chem., 2009, 27, 123–129 CrossRef CAS PubMed;
(b) G. K. He, H. Guo, R. Qian, Y. L. Guo, C. L. Fu and S. M. Ma, Tetrahedron, 2009, 65, 4877–4889 CrossRef CAS PubMed.
-
(a) X. Mu, T. Wu, H. Y. Wang, Y. L. Guo and G. Liu, J. Am. Chem. Soc., 2012, 134, 878–881 CrossRef CAS PubMed;
(b) H. Peng, Z. Yuan, H. Y. Wang, Y. L. Guo and G. Liu, Chem. Sci., 2013, 4, 3172–3178 RSC;
(c) W. Zhu, H. Wang, H. Peng, G. Liu and Y. Guo, Chin. J. Chem., 2013, 31, 371–376 CrossRef CAS PubMed;
(d) Z. Yuan, H. Y. Wang, X. Mu, P. Chen, Y. L. Guo and G. Liu, J. Am. Chem. Soc., 2015, 137, 2468–2471 CrossRef CAS PubMed.
- L. J. Guo, Y. Xu, X. J. Wang, W. J. Liu and D. P. Lu, Organometallics, 2013, 32, 3780–3783 CrossRef CAS.
-
(a) X. Zhang, Y. X. Liao, R. Qian, H. Y. Wang and Y. L. Guo, Org. Lett., 2005, 7, 3877–3880 CrossRef CAS PubMed;
(b) X. Zhang, H. Y. Wang and Y. L. Guo, Rapid Commun. Mass Spectrom., 2006, 20, 1877–1882 CrossRef CAS PubMed.
-
(a) H. Y. Wang, J. Zhou and Y. L. Guo, Rapid Commun. Mass Spectrom., 2012, 26, 616–620 CrossRef CAS PubMed;
(b) H. Y. Wang, Z. Xiang, G. S. Liu and Y. L. Guo, J. Am. Soc. Mass Spectrom., 2013, 24, 761–767 CrossRef CAS PubMed;
(c) Y. Gao, H. Y. Wang, X. Zhang, J. S. Cheng, F. Zhang and Y. L. Guo, J. Mass Spectrom., 2014, 49, 481–489 CrossRef CAS PubMed.
- X. He, S. Zhang, Y. Guo, H. Wang and G. Lin, Organometallics, 2012, 31, 2945–2948 CrossRef CAS.
- L. S. Santos and J. O. Metzger, Angew. Chem., Int. Ed., 2006, 45, 977–981 CrossRef PubMed.
- H. Wang and J. O. Metzger, Organometallics, 2008, 27, 2761–2766 CrossRef CAS.
-
(a) H. Y. Wang, W. L. Yim, T. Kluener and J. O. Metzger, Chem. – Eur. J., 2009, 15, 10948–10959 CrossRef CAS PubMed;
(b) H. Y. Wang, W. L. Yim, Y. L. Guo and J. O. Metzger, Organometallics, 2012, 31, 1627–1634 CrossRef CAS.
- Z. X. Zhao, H. Y. Wang and Y. L. Guo, Rapid Commun. Mass Spectrom., 2011, 25, 3401–3410 CrossRef CAS PubMed.
- Z. X. Zhao, H. Y. Wang and Y. L. Guo, J. Mass Spectrom., 2011, 46, 856–858 CrossRef CAS PubMed.
- J. M. He, Z. Abliz, R. P. Zhang, Y. X. Liang and K. L. Ding, Anal. Chem., 2006, 78, 4737–4740 CrossRef CAS PubMed.
- A. T. Lubben, J. S. McIndoe and A. S. Weller, Organometallics, 2008, 27, 3303–3306 CrossRef CAS.
-
(a) H. W. Chen, A. Venter and R. G. Cooks, Chem. Commun., 2006, 2042–2044 RSC;
(b) W. S. Law, R. Wang, B. Hu, C. Berchtold, L. Meier, H. W. Chen and R. Zenobi, Anal. Chem., 2010, 82, 4494–4500 CrossRef CAS PubMed;
(c) C. A. Marquez, H. Wang, F. Fabbretti and J. O. Metzger, J. Am. Chem. Soc., 2008, 130, 17208–17209 CrossRef CAS PubMed.
|
This journal is © the Partner Organisations 2015 |
Click here to see how this site uses Cookies. View our privacy policy here.