DOI:
10.1039/C4QI00125G
(Research Article)
Inorg. Chem. Front., 2015,
2, 263-272
Inorganic anion-assisted supramolecular assemblies of bent dipyridines: effects of anionic geometries on hydrogen-bonding networks†
Received
1st September 2014
, Accepted 14th January 2015
First published on 14th January 2015
Abstract
A series of inorganic acids were introduced into the self-assembly with the bent 2,5-bis(4-pyridyl)-1,3,4-oxadiazole (4-bpo), yielding seven anion-assisted supramolecular salts, i.e. [(4-H2bpo2+)·(NO3−)2]·2H2O (1), (4-H2bpo2+)·(HPO42−) (2), [(4-H2bpo2+)2·(4-Hbpo+)·(I3−)3·(I−)2]·2H2O (3), [(4-Hbpo+)2·(PF6−)2]·H2O (4), [(4-H2bpo2+)·(BF4−)2]·H2O (5), [(4-Hbpo+)·(BF4−)] (6) and (4-H2bph2+)·(SO42−) (7). Structural analyses indicate that different inorganic anions (e.g. spherical, linear, trigonal planar, tetrahedral and octahedral) can induce the formation of diverse supramolecular architectures, influencing the crystallization ratio, the protonated number and the final structure. The anions are hydrogen bonded to the angular dipyridine, which offers a sufficient driving force for the directed assembly of supramolecular hydrogen-bonding frameworks. Thereto, various hydrogen-bonding motifs are observed in all these cases (1–7) and the nitrogen atoms of pyridines are protonated apart from salt 6. Among them, salts 1, 3, 4 and 5 crystallize with water molecules but the others do not. Interestingly, appealing substructures have been generated by anions and water molecules in 1, 3 and 5 but not in 4. HPO42− dimers form in 2 despite there being no assistance from solvent water. BF4− anions facilitate the formation of the helical chain in 6. Unexpectedly, the oxadiazole ring opened via the in situ hydrolysis under hydrothermal conditions during crystallization with H2SO4, producing salt 7.
Introduction
Inspired by the fundamental and vital roles of ubiquitous anions in the areas of chemistry, biology, medicine and environmental issues, an upsurge in anion supramolecular chemistry has been witnessed in the past few decades.1–10 However, since the crystallization process will be complicated by its high charge density,11 and the formation of hydrogen bonds and anion⋯π interactions is unforseeable,12 the strategy of using inorganic anions for the synthesis of supramolecular architectures remains underdeveloped.1,10,13–21 By serendipitous discoveries, anions have proven their ability to control supramolecular assemblies by interacting with their receptors, such as through hydrogen-bonding, electrostatic and anion⋯π interactions.11,22–27
Therefore, worldwide chemists endeavor to tackle the current challenges posed by anions and systematic research on the structural variations and physicochemical properties of supramolecular arrays is increasingly being pursued based on inorganic anions and their receptors.28–35 From a structural point of view, anions may play various roles in dynamic crystallization processes, by acting as, for instance, a structure-directing agent, a spectator merely for charge balance, a building unit or a secondary building unit (SBU).11 The final structure can be effectively influenced by the nature and geometry of the inorganic anions, i.e. spherical (halides), linear (triiodide), coplanar (carboxylate), trigonal planar (nitrate), tetrahedral (sulfate) and octahedral (hexafluorophosphate).6,12,13
Intermolecular non-covalent interactions facilitate the process of supramolecular assembly between anions and their receptors with functional groups. Currently, multifarious novel artificial anion receptors have emerged, ranging from amide/urea, pyrrole/indole, guanidinium, polyammonium, calix[n]phyrins, imidazolium to pyridine/pyridinium.36,37 Among them, we have reported supramolecular salts based on bent dipyridyl 2,2′-dipyridylamine (dpa) as a proton sponge38 and have an ongoing interest in the appealing angular dipyridyl 2,5-bis(4-pyridyl)-1,3,4-oxadiazole (4-bpo). The relatively flexible conformation can induce higher-dimensional supramolecular architectures more easily compared with linear dipyridyl derivatives.39 Furthermore, protonation or quaternization occurs readily in the pyridine heterocycles, resulting in pyridinium groups which can interact with anions through a range of noncovalent interactions such as electrostatics, increasingly polarized hydrogen bonds (including CH⋯A¬) or electrostatic aryl–aryl stacking interactions.36
Although Du et al. introduced 4-bpo to a series of organic acids to construct supramolecular crystalline materials,39–43 systematic research has not been carried out on the system of inorganic acids except for two discrete ionic salts.44,45 Herein, we synthesized seven inorganic anion-assisted supramolecular salts (Scheme 1), namely [(4-H2bpo2+)·(NO3−)2]·2H2O (1), (4-H2bpo2+)·(HPO42−) (2), [(4-H2bpo2+)2·(4-Hbpo+)·(I3−)3·(I−)2]·2H2O (3), [(4-Hbpo+)2·(PF6−)2]·H2O (4), [(4-H2bpo2+)·(BF4−)2]·H2O (5), [(4-Hbpo+)·(BF4−)] (6) and (4-H2bph2+)·(SO42−) (7).
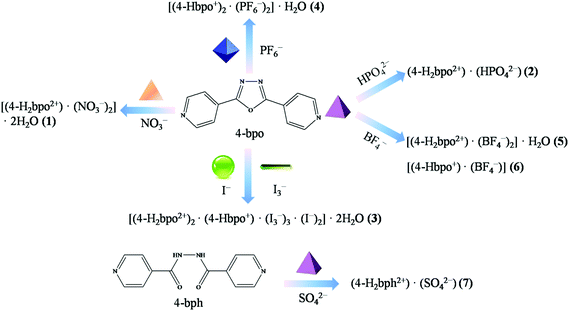 |
| Scheme 1 Synthesized proton-transfer supramolecular salts (1–7), induced by different geometrical anions (spherical, linear, trigonal planar, tetrahedral and octahedral). | |
The variation of anions has directed entirely different supramolecular architectures, diverse hydrogen-bonding motifs and interesting substructures with water. Meanwhile, hydrothermal conditions show control over the crystallization process, such as the change of the protonated site in salt 6 and the hydrolysis of 4-bpo in 7. It is extremely rare that the oxadiazole is protonated except in the one case46 when diazole and pyridine rings coexist according to the Cambridge Structure Database (CSD).
Experimental
Syntheses of the compounds 1–7
4-Bpo was prepared according to the literature procedures,47 and other chemical reagents and solvents (analysis grade) were commercially available (Sigma-Aldrich) and used without further purification.
[(4-H2bpo2+)·(NO3−)2]·2H2O (1).
To a methanol solution (5 mL) containing 4-bpo (0.1 mmol) an aqueous solution (5 mL) of HNO3 (0.2 mmol) was added with constant stirring for 30 min. The resultant clear solution was allowed to evaporate under ambient conditions, affording colorless needle-shaped crystals after one week. The crystals were separated from the mother liquor by filtration, washed with cool methanol solution, and dried under vacuum.
(4-H2bpo2+)·(HPO42−) (2).
4-Bpo (0.1 mmol) in methanol (5 mL) and H3PO4 (0.1 mmol) in water (5 mL) were mixed with stirring according to the procedure for 1. Upon slow evaporation of the solvents, good-quality colorless rodlike crystals of 2 were obtained after two weeks.
[(4-H2bpo2+)2·(4-Hbpo+)·(I3−)3·(I−)2]·2H2O (3).
The same synthetic procedure for 1 was used except that HNO3 was replaced by HI, giving yellow lamellar single crystals suitable for X-ray analysis over a period of ten days.
[(4-Hbpo+)2·(PF6−)2]·H2O (4).
Well-shaped colorless rodlike crystals of 4 were isolated after about one week by a similar procedure as described for 1, except with the addition of HPF6 instead of HNO3.
[(4-H2bpo2+)·(BF4−)2]·H2O (5).
Similarly to 1, a methanol–water (v
:
v = 1
:
1, 10 mL) mixture of 4-bpo (0.1 mmol) and HBF4 (0.2 mmol) was allowed to evaporate under ambient conditions, affording colorless rodlike crystals of 5 after two weeks.
[(4-Hbpo+)·(BF4−)] (6).
The mixture of 4-bpo (0.1 mmol) and HBF4 (0.1 mmol) in DMF (10 mL) was placed in a Parr Teflon-lined stainless steel vessel (20 mL) under autogenous pressure, which was heated to 120 °C for 48 h and subsequently cooled to room temperature at a rate of 10 °C h−1. Colorless block crystals of 6 were obtained.
(4-H2bph2+)·(SO42−) (7).
A water (10 mL) solution containing 4-bpo (0.1 mmol) and H2SO4 (0.1 mmol) was placed in a Parr Teflon-lined stainless steel vessel (20 mL) under autogenous pressure, which was heated to 140 °C for 72 h and subsequently cooled to room temperature at a rate of 5 °C h−1. Colorless block crystalline products were collected.
Single crystal X-ray diffraction
Crystal data of 1–7 were collected using a Bruker SMART APEX diffractometer employing graphite-monochromated Mo Kα radiation (λ = 0.71073 Å) at 293 K. The data integration and reduction were undertaken with SAINT. The structure was solved by the direct method using SHELXS-97 and all the non-hydrogen atoms were refined anisotropically on F2 by the full-matrix least-squares technique using SHELXL-97. Non-H atoms were refined anisotropically using all reflections with I > 2σ(I). All H atoms (except for those bound to the N atoms on the pyridine and oxadiazole rings) were placed in calculated positions with fixed isotropic thermal parameters. The hydrogen atoms bonded to the N atoms of the pyridine and oxadiazole rings were located from difference maps. The packing views and the asymmetric units were drawn with DIAMOND (Brandenburg and Putz, 2004) Visual Crystal Structure Information System Software. Crystallographic data and structure refinement are listed in Table 1.
Table 1 Crystallographic data for complexes 1–7
Compounds |
1
|
2
|
3
|
4
|
5
|
6
|
7
|
Formula |
C12H14N6O9 |
C12H11N4O5P |
C36H33I11N12O5 |
C24H20F12N8O3P2 |
C12H12B2F8N4O2 |
C12H9BF4N4O |
C12H12N4O6S |
Formula weight |
386.29 |
322.22 |
2109.64 |
758.42 |
417.88 |
312.04 |
340.32 |
Crystal system |
Monoclinic |
Monoclinic |
Triclinic |
Triclinic |
Monoclinic |
Triclinic |
Monoclinic |
Space group |
P21/c |
C2/c |
P![[1 with combining macron]](https://www.rsc.org/images/entities/char_0031_0304.gif) |
P![[1 with combining macron]](https://www.rsc.org/images/entities/char_0031_0304.gif) |
P21/n |
P![[1 with combining macron]](https://www.rsc.org/images/entities/char_0031_0304.gif) |
P21/c |
a (Å) |
7.4845 (15) |
14.716 (3) |
10.637 (2) |
10.491 (2) |
5.4030 (11) |
8.4767 (17) |
7.2667(15) |
b (Å) |
18.648 (4) |
17.249 (3) |
13.967 (3) |
10.784 (2) |
19.358 (4) |
8.9502 (18) |
13.421(3) |
c (Å) |
11.768 (2) |
10.967 (2) |
21.237 (4) |
14.359 (3) |
16.265 (3) |
9.2925 (19) |
14.883(3) |
α (°) |
90.00 |
90.00 |
82.92 (3) |
73.30 (3) |
90.00 |
80.43 (3) |
90.00 |
β (°) |
90.46(3) |
94.24(3) |
76.08 (3) |
76.73 (3) |
95.94(3) |
78.98 (3) |
104.05(3) |
γ (°) |
90.00 |
90.00 |
69.64 (3) |
80.13 (3) |
90.00 |
80.97 (3) |
90.00 |
V (Å3) |
1642.4(6) |
2776.2(10) |
2868.4 (10) |
1504.7 (5) |
1692.0(6) |
676.6 (3) |
1408.1(5) |
D
x
(Mg m−3) |
1.562 |
1.542 |
2.443 |
1.674 |
1.640 |
1.532 |
1.605 |
μ (mm−1) |
0.14 |
0.23 |
5.99 |
0.26 |
0.17 |
0.14 |
0.27 |
Z
|
4 |
8 |
2 |
2 |
4 |
2 |
4 |
T (K) |
293 |
293 |
293 |
293 |
293 |
293 |
293 |
F (000) |
800 |
1328 |
1912 |
764 |
840 |
316 |
704 |
θ range for data collection (°) |
3.4–27.5 |
3.0–27.5 |
3.0–27.5 |
3.0–25.0 |
3.3–27.5 |
3.0–27.5 |
3.0–27.5 |
Index ranges |
−9 ≤ h ≤ 9 |
−19 ≤ h ≤ 19 |
−13 ≤ h ≤ 13 |
−12 ≤ h ≤ 12 |
−6 ≤ h ≤ 7 |
−11 ≤ h ≤ 10 |
−9 ≤ h ≤ 9 |
−24 ≤ k ≤ 24 |
−22 ≤ k ≤ 22 |
−18 ≤ k ≤ 18 |
−12 ≤ k ≤ 12 |
−25 ≤ k ≤ 25 |
−11 ≤ k ≤ 11 |
−17 ≤ k ≤ 17 |
−15 ≤ l ≤ 15 |
−14 ≤ l ≤ 14 |
−27 ≤ l ≤ 27 |
−17 ≤ l ≤ 17 |
−21 ≤ l ≤ 21 |
−12 ≤ l ≤ 12 |
−19 ≤ l ≤ 19 |
Measured reflections |
16 990 |
14 250 |
29 862 |
13 015 |
17 285 |
7084 |
14 393 |
Independent reflections |
3759 |
3180 |
13137 |
5303 |
3859 |
3093 |
3233 |
Reflections with I > 2σ(I) |
2199 |
1801 |
7606 |
3404 |
1650 |
2110 |
2767 |
R
int
|
0.084 |
0.080 |
0.056 |
0.054 |
0.130 |
0.036 |
0.034 |
R
1 [I > 2σ(I)] |
0.065 |
0.059 |
0.053 |
0.077 |
0.092 |
0.065 |
0.039 |
wR2 (all data) |
0.166 |
0.167 |
0.106 |
0.234 |
0.261 |
0.206 |
0.107 |
S
|
1.02 |
1.04 |
1.02 |
1.04 |
1.03 |
1.05 |
1.11 |
Results and discussion
All the one-step reactions were carried out either in a 1
:
2 or 1
:
1 molar ratio between the bent dipyridine-based synthon 4-bpo and the inorganic acid (HNO3, H3PO4, HI, HPF6, HBF4 and H2SO4, respectively), affording seven proton-transfer supramolecular salts 1–7. For the preparation of 1–5, the inorganic acid was mixed directly with 4-bpo in a methanol–water (v
:
v = 1
:
1, 10 mL) solution, which was allowed to evaporate under ambient conditions and yielded the final crystalline product. During crystallization, protons are transferred from the acid to the nitrogen atoms of the pyridine rings. However, the nitrogen of oxadiazole was protonated by HBF4 in 6 under solvothermal conditions. Interestingly, besides different protonated sites, these salts were obtained as different two-component ratios. In addition, salts 1, 3, 4 and 5 crystallized with water molecules while others did not contain solvent molecules. For compound 7, hydrothermal reactions made the oxadiazole ring open and produced a new organic compound N,N′-bis(4-picolinoyl) hydrazine (4-bph) from the in situ hydrolysis of 4-bpo during crystallization with H2SO4. The schematic representations of salts 1–7 are summarized in Scheme 1. Hydrogen-bonding geometries and weak intermolecular interactions are listed in Tables S1 and S2,† respectively.
Structure description of compound 1
Salt 1 crystallizes in a monoclinic space group P21/c with one diprotonated 4-H2bpo2+ cation, two trigonal planar NO3− anions and two water molecules in the asymmetric unit (Fig. S1a†). Two NO3− anions both exhibit five hydrogen bonds with 4-H2bpo2+ cations and H2O molecules due to their three oxygen atoms which act as hydrogen-bond acceptors, and range from strong O–H⋯O, N–H⋯O to weak C–H⋯O hydrogen bonds (H⋯A, 1.88–2.56 Å; ∠D–H⋯A, 126–175°) (Fig. 1a and Table S1†). Moreover, the N6-containing nitrate anion interacts with the N1-containing pyridine and oxadiazole ring in the same 4-H2bpo2+ molecule through anion⋯π interactions, where the distances are 3.766 and 3.228 Å between the closest oxygen atom and the centroid of the aromatic ring, respectively (Table S2†).
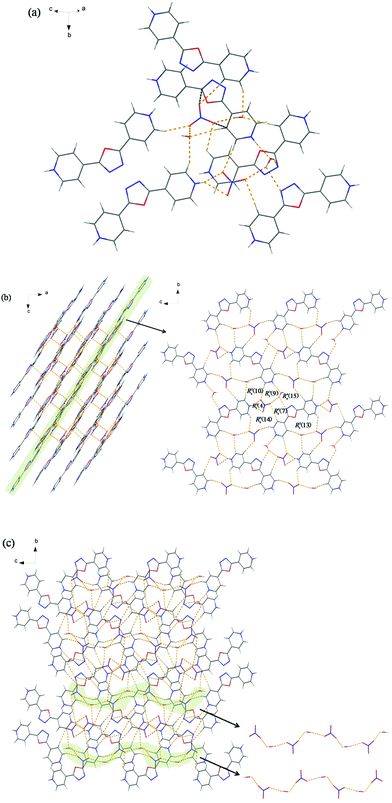 |
| Fig. 1 (a) The coordination sphere of NO3− anions in 1; (b) the 3D hydrogen-bonding network parallel to the ac-plane and a side view of the middle chain in pale green viewed along the a-axis; (c) the 3D crystal stacking along the a-axis and separate molecular structure of the NO3− anions together with water molecules. Hydrogen bonds and anion⋯π interactions are represented by light orange and black dotted lines, respectively. | |
Robust and extensive hydrogen-bonding interactions play a crucial role in crystal packing. Viewed along the b-axis, the 4-H2bpo–nitrate hydrogen-bonding network of 1 is three-dimensional (3D), where parallel chains are connected by O9–H14⋯O7 and O8–H12⋯N2 hydrogen bonds (Fig. 1b). The middle one in pale green has been separated and found as a two-dimensional framework viewed along the a-axis, which comprises various ring motifs through robust hydrogen-bonding interactions, i.e. R12(4), R22(7), R33(9), R42(10), R32(13), R44(14) and R44(15). Thereto, 4-H2bpo2+ cations are homo-orientated and parallel to each other in the same layer and every two such layers recur.
When viewed along the a-axis, the 3D hydrogen-bonding framework seems not to be in good order since 4-H2bpo2+ cations are interweaved by NO3− anions and water molecules (Fig. 1c). Anions and water are separated in the pale green background sections and chainlike substructures are observed in an antiparallel arrangement. After anions and water molecules have been removed for clarity, we are surprised that no hydrogen-bonding interactions exist among the 4-H2bpo2+ cations. But they seem to be wavelike quasi-chains, which in light blue, nearly show mirror symmetry to the adjacent one (Fig. S1b†). Even though direct hydrogen-bonding interactions are not involved, cations are guided by NO3− anions and H2O molecules, resulting in the regular structure. It's not hard to see that the NO3− anion greatly influences the formation of the crystal structure.
Structure description of compound 2
Single-crystal diffraction analysis reveals that 2 crystallizes in the monoclinic space group C2/c and its molecular structure comprises one diprotonated 4-H2bpo2+ cation and a HPO42− anion (Fig. S2a†). As shown in Fig. 2a, one HPO42− anion is bound to four 4-H2bpo2+ cations through N–H⋯O and C–H⋯O hydrogen bonds. Anion⋯π interaction is found between the O3 atom and the oxadiazole ring with the distance of 3.440 Å while π⋯π interactions are among the 4-H2bpo2+ cations (Cg1⋯Cg1 and Cg2⋯Cg3). Notably, an O2 atom can serve as a hydrogen-bonding donor as well as an acceptor, which facilitates the formation of a HPO42− dimer via strong hydrogen bonds O2–H11⋯O5, notated as R22(8) (Fig. 2b). Such dimers are linked by 4-H2bpo2+ cations via strong intermolecular N1–H3⋯O3 and N4–H8⋯O4 hydrogen bonds, offering a large ring R66(40). The four pairs of anions and cations look like a quasi-hexagon viewed along the b-axis, which extends to a 3D framework on the ac-plane by hydrogen-bonding interactions.
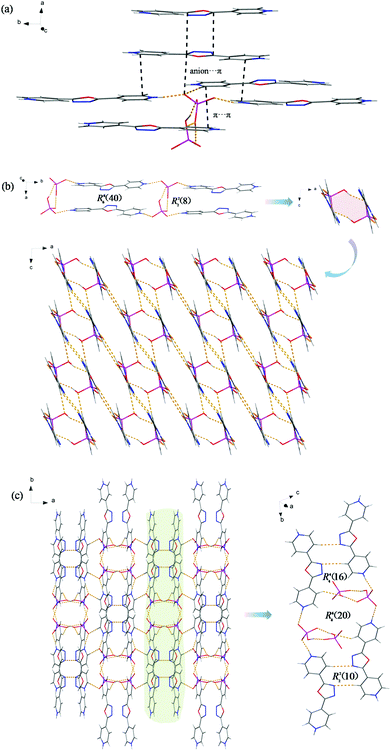 |
| Fig. 2 (a) The coordination sphere of the HPO42− anion in 2; (b) a moiety of molecular structure and its stretch on the ac-plane into a 3D framework through hydrogen bonds; (c) the 3D crystal stacking along the c-axis and a side view of molecular structure in pale green. Hydrogen bonds are represented by light orange dashed lines while anion⋯π and π⋯π interactions are black. | |
From another perspective, it has a different structure. Indeed, as depicted in Fig. 2c, the 4-H2bpo2+–HPO42− hydrogen-bonding framework consists of orderly chains through the weak C2–H2⋯O3 hydrogen bond (H2⋯O3, 2.53 Å; ∠C2–H2⋯O3, 174°). This indicates that weak hydrogen-bonding interactions also play an important role in the supramolecular assembly. Two groups of HPO42− dimers interweave the 4-H2bpo2+ cations to show an oval while the 4-H2bpo2+ cations are packed to produce a little grid. For clarity, the moiety can be disentangled in pale green and the side view is given on the right. We find the grid to be a ten-membered ring between two cations owing to C4–H5⋯N2 hydrogen bonds. Another two kinds of large hydrogen-bonding motifs are afforded between anions and cations with the graph-sets of R44(16) and R66(20).
Structure description of compound 3
Components in 3 seem slightly richer, which contain two diprotonated 4-H2bpo2+ cations, one monoprotonated 4-Hbpo+ cation, three linear I3− anions, two spherical I− anions and two water molecules in the asymmetric unit (Fig. S3a†). As illustrated in Fig. 3a, I10− and I11− anions both connect three cations and one H2O molecule through intermolecular interactions (O–H⋯I, N–H⋯I and C–H⋯I) while three I3− anions interact with each other by short I⋯I contacts (I3⋯I9, 3.837 Å; I1⋯I6, 3.922 Å), significantly below the sum of the van der Waals radii of iodine. For the cations, π⋯π interactions appear (Cg2⋯Cg1, 3.589 Å; Cg7⋯Cg4i, 3.400 Å).
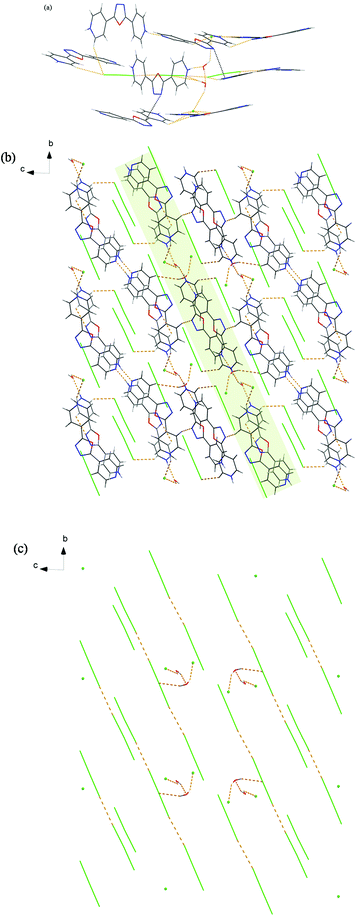 |
| Fig. 3 (a) The coordination sphere of the iodide anion in 3 where a ball-and-stick model was used for I− ions for clarity; (b) the 3D hydrogen-bonding network parallel to the bc-plane; (c) the structure with cations deleted through hydrogen bonds and short contacts. Hydrogen bonds and π⋯π interactions are represented by light orange and black dotted lines, respectively. | |
Besides the above X–H⋯I (X = O, N, C) interactions, short I⋯I contacts and π⋯π interactions, some hydrogen bonds not involved in iodide ions codetermine the supramolecular network, ranging from O–H⋯O, N–H⋯Y (Y = N, O) to C–H⋯N (Fig. 3b). Declining cationic chains are surrounded by bilateral linear I3− anions while spherical I− anions always accompany H2O molecules and appear near the protonated pyridine rings. In order for better observation of the packing of iodine ions and water molecules, the cations have been deleted in Fig. 3c. The triiodides are in perfect alignment while I10 and I11 are hydrogen-bonded to two H2O molecules, one of which is linked with and I2 atom from the centre of the triiodide trimer producing an appealing subunit.
Packing parallel to the ac-plane, we can see that the supramolecular architecture is composed of independent substructures (Fig. S3b†). Diprotonated 4-H2bpo2+ cations alternate with monoprotonated 4-Hbpo+ ones in the first and second layer and the two types of molecules show a certain inclination angle. Throughout the two adjacent layers, molecules of the same kind are arranged face to face with their diagonal ones. Somewhat differently, only diprotonated 4-H2bpo2+ cations appear in the third layer and molecules in the ortho-position are antiparallel to each other. The above independent substructures appeal to us, one of which has been selected in the pale green background. As shown in Fig. S3c,† the subunit was rotated a little with triiodide ions deleted, and then the side view was observed where bent chains are not connected with each other. Among them, the chain in pale green is viewed along the c-axis and three types of hydrogen-bonding ring motifs unfold before us, namely intramolecular S(5) and intermolecular large ring R106(36) as well as R86(56).
Structure description of compound 4
Although it possesses the same triclinic space group, P
, as 3, compound 4 exhibits quite a different structure. The molecular structure of 4 consists of two monoprotonated 4-Hbpo+ cations, two octahedral PF6− anions and one H2O molecule, where fluorine atoms are in disorder (Fig. 4a and S4a†). 4-Hbpo+ cations and PF6− anions are interlinked to each other through C–H⋯F hydrogen bonds (H⋯F, 2.41–2.51 Å; ∠C–H⋯F, 135–177°) and anion⋯π interactions (anion–centroid distance, 3.051–3.722 Å). Adjacent 4-Hbpo+ cations interact with each other through hydrogen-bonding X–H⋯N (X = N, C) and π⋯π interactions with a distance of 3.418 Å.
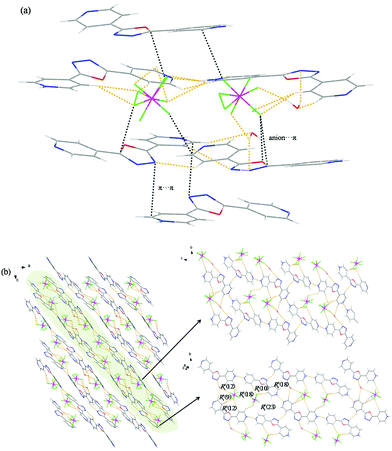 |
| Fig. 4 (a) The coordination sphere of the PF6− anion in 4; (b) the 3D hydrogen-bonding network parallel to the ac-plane and the twin chains in pale green viewed along the a-axis. Hydrogen bonds are represented by light orange dashed lines while anion⋯π and π⋯π interactions are black. | |
These intermolecular interactions act as the driving force in the crystal packing. Running parallel to the ac-plane as shown in Fig. 4b, discrete chains are spread over the whole space and the twin chains are antiparallel to each other with the PF6− anions sandwiched between them. Thereto, such a pair of chains was chosen as the further object of study in the pale green background. 2D supramolecular arrays appear before us on the right, which comprise multifarious ring motifs with graph set notation R33(9), R21(10), R22(12), R33(12), R44(18), R55(18) and R55(23). Packing parallel to the ab-plane (Fig. S4b†), the supramolecular architecture seems terribly disorganized. In fact, 4-Hbpo+ cations of random stacking circle relatively regular PF6− anions, just like in metal–organic frameworks (MOFs).
Structure description of compound 5
For 5, one bent dipyridine was diprotonated by two HBF4 molecules and the coordination sphere of two tetrahedral BF4− anions has been illustrated in Fig. 5a. The three components (4-H2bpo2+–BF4−–H2O) are closely connected to each other due to a wide variety of hydrogen bonds (O–H⋯F, N–H⋯X (X = O, F), C–H⋯Y (Y = N, F)), anion⋯π interactions and π⋯π interactions. They extend on the ac-plane to form a 3D supramolecular architecture, where cationic and anionic layers emerge alternately and seem to be sandwiched by each other (Fig. 5b). In the 4-H2bpo2+ layer, two cations of a pair are arrayed face to face and connected with another couple by the C12–H10⋯N3 hydrogen bond.
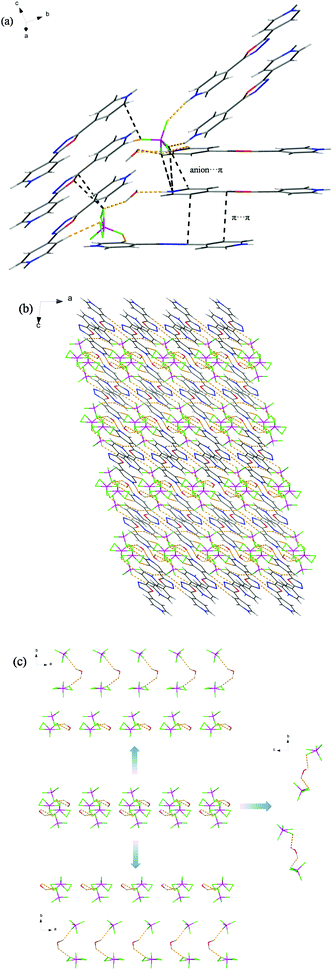 |
| Fig. 5 (a) The coordination sphere of the BF4− anion in 5; (b) the 3D hydrogen-bonding network parallel to the ac-plane; (c) the BF4− anionic substructures. Hydrogen bonds are represented by light orange dashed lines while anion⋯π and π⋯π interactions are black. | |
We find that BF4− ions in the anionic layer are packed like an independent scarecrow together with H2O molecules, several of which resemble soldiers standing in a line (Fig. 5c). Such a BF4− layer changes to two groups of discontinuous three-component substructures viewed along the a-axis so that we guess the above scarecrow-like structure may not be uniform. Indeed, two sets of symmetrical subunits are observed. Viewed along the c-axis, the v-shaped structures align, where a H2O molecule bridges the BF4− anions on both ends through the hydrogen-bonding interactions O2–H11⋯F4 and O2–H12⋯F5′. When stacking along the a-axis, the three components in a staggered arrangement are weaved into a supramolecular framework, offering seven types of different hydrogen-bonding rings, i.e. R12(4), R32(7), R22(10), R44(14), R44(15), R44(16) and R44(19) (Fig. S5b†).
Structure description of compound 6
Although the same reactants were adopted in comparison with 5, the different reaction ratio (1
:
1) and conditions lead to a disparate crystal structure and space group (triclinic P
) in 6. Structure determination revealed that one monoprotonated 4-Hbpo+ cation counters one tetrahedral BF4− anion without a disordered fluorine atom and the protonated nitrogen atom comes from oxadiazole rather than pyridine, which is extremely rare for the coexistence of diazole and pyridine rings. Every BF4− anion attaches to five nearby 4-Hbpo+ cations via C–H⋯F hydrogen bonds, anion⋯π and N–H⋯π interactions (Fig. 6a). It is worth mentioning that the BF4− anions in 6 play a decisive role in the crystal packing since almost all hydrogen bonds take fluorine atoms as acceptors except hydrogen bond C9–H5⋯N3. Specifically, their F2 and F4 atoms are employed to bridge two neighbouring columns of 4-Hbpo+ cations through hydrogen-bonding interactions, facilitating the 3D supramolecular architecture (Fig. S6b†).
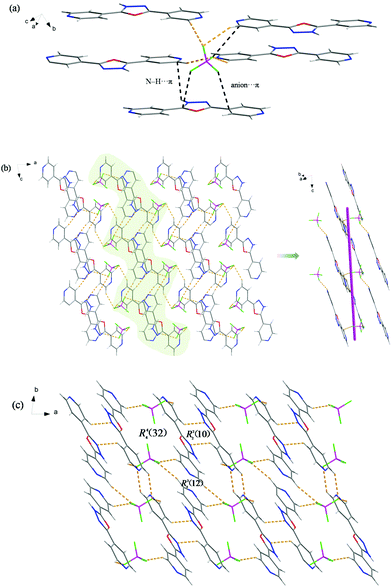 |
| Fig. 6 (a) The coordination sphere of the BF4− anion in 6; (b) the 3D hydrogen-bonding network parallel to the ac-plane and the helical structure from the side view of the zigzag ribbon-like chain in the pale green background; (c) the 3D hydrogen-bonding network parallel to the ab-plane. Hydrogen bonds are represented by light orange dashed lines while anion⋯π and N–H⋯π interactions are black. | |
Salt 6 just exhibits weak hydrogen-bonding interactions (C–H⋯N and C–H⋯F), anion⋯π and N–H⋯π interactions, but that does not impede the 3D supramolecular assembly. Running parallel to the ac-plane, 4-Hbpo+ cations and BF4− anions locate alternately, producing a zigzag ribbon-like chain in the pale green background (Fig. 6b). From its side view on the right, the helical chain was observed and linked by BF4− anions. Weak C10–H6⋯F2 interactions help to maintain the linkages between these chains that align parallel to each other. Viewed along the c-axis, three types of hydrogen-bonding patterns are found to extend on the ab-plane, marked as R22(10), R42(12), and R44(32) (Fig. 6c).
Structure description of compound 7
Different from the above six salts, hydrothermal conditions induce the oxadiazole ring in 4-bpo to hydrolyze in situ during crystallization with H2SO4, yielding a new organic compound N,N′-bis(4-picolinoyl) hydrazine (4-bph). Salt 7 crystallizes in the same space group, P21/c, as 1 and one diprotonated 4-H2bph2+ cation counterbalances one tetrahedral SO42− anion in the asymmetric unit (Fig. S7a†). Compared with the oxadiazole functional group in the above study, the amide (N–H) and carbonyl group (C
O) in 4-bph are strong hydrogen-bonding participators, which make it a more abundant hydrogen-bonding system.
All oxygen atoms (O1–O6) are used as hydrogen-bonding acceptors, resulting in multifarious hydrogen bonds from strong N–H⋯O to weak C–H⋯O. The four oxygen atoms of the SO42− anion all participate in the formation of hydrogen bonds with its surrounding 4-H2bph2+ cations, as shown in Fig. 7a. Among them, the O4 and O5 atoms are bound to cations through anion⋯π interactions. Seven kinds of hydrogen-bonding motifs are generated with the graph-sets R12(4), R21(7), R22(9), R32(10), R33(12), R33(13) and R22(18). Further analysis of the crystal packing indicates that grid-like ribbons or ladder-like chains are discrete and pack antiparallel to the adjacent ones (Fig. 7b and c).
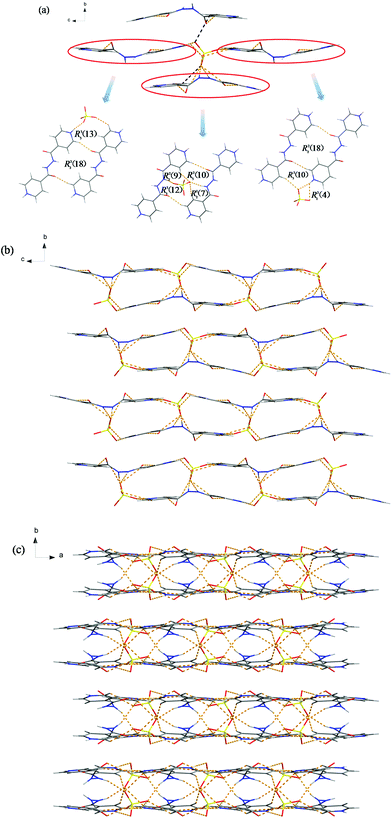 |
| Fig. 7 (a) The coordination sphere of the SO42− anion in 7 and the perspective view of the subunit between one SO42− anion and its surrounding 4-H2bph2+ cations; (b) the 3D hydrogen-bonding network parallel to the bc-plane; (c) the 3D hydrogen-bonding network parallel to the ab-plane. Hydrogen bonds and anion⋯π interactions are represented by light orange and black dotted lines, respectively. | |
Structural diversity influenced by the inorganic anions
To explore the influence of inorganic anions on the angular dipyridine (4-bpo), we have obtained a series of supramolecular salts 1–7, assisted by NO3−, HPO42−, I3−/I−, PF6−, BF4−, and SO42−. Inorganic anions act as the dominant factor in the formation of supramolecular assemblies by impacting the crystallization ratio, the protonated number and the final structures, which are attributed to their nature and geometry (spherical, linear, trigonal planar, tetrahedral and octahedral).
A detailed analysis of the surrounding environments of the anions has been carried out, and it was found that they all participate in the hydrogen-bonding interactions and anion⋯π interactions are widely utilized, except in salt 3. With the assistance of water molecules, several anions result in appealing substructures. For instance, trigonal planar NO3− is interlinked to H2O molecules in 1, offering a wavelike chain. In 3, linear triiodide trimers are generated by short I⋯I contacts while two spherical iodine atoms are hydrogen-bonded to two H2O molecules. The central I2 atom is bridged to one H2O molecule, producing an appealing subunit. As for compound 5, the H2O molecule is bound by two BF4− anions on both ends, forming the V-shaped structure that is packed to afford independent scarecrow-like substructures in a line. In spite of no assistance from water molecules, dimers arise from HPO42− anions in 2 due to the coexistence of potential hydrogen-bonding donor and acceptor oxygen atoms.
In comparison, salts 4, 6 and 7 do not maintain such a close relationship with solvent water in appearance. Though compound 4 crystallizes together with H2O, the water molecules are locked by nearby 4-Hbpo+ cations through hydrogen-bonding interactions (O3–H20⋯N3 and C2–H2⋯O3), which may lower the possibility of anion–water substructures. For compounds 6 and 7, they do not contain crystallizing H2O molecules. The hydrothermal conditions show control over the crystallization process, changing the protonated site of 4-bpo in 6 and inducing the hydrolysis of 4-bpo in 7.
Overall, these salts (1–7) exhibit various supramolecular architectures, a wide variety of hydrogen-bonding ring motifs and interesting substructures. From the view of intermolecular interactions, hydrogen bonds (Table S1†) and weak forces (anion⋯π and π⋯π interactions, Table S2†) codetermine the whole crystal packing and show both competitive and complementary roles in the formation of the crystal structure. Once strong hydrogen bonds are quenched, the C–H bond is a little acidic in nature and can be used as a donor to interact with hydrogen-bonding acceptors. For example, weak hydrogen-bonding interactions (C–H⋯F and C–H⋯N) are exploited to construct supramolecular frameworks and BF4− anions facilitate the formation of helical chains in 6.
In addition, two salts based on 4-bpo, [(4-H2bpo2+)(ClO4−)2]·H2O44 and [(4-H2bpo2+)(Br−)2]·H2O,45 have been reported previously. Structural analysis indicates that the former shows great similarity to compound 5 in the supramolecular networks, where V-shaped subunits are formed between the ClO4− anions and H2O molecules (Fig. S5b and S8†). This may be accounted for by the similar tetrahedral ClO4− and BF4− anions. The latter bromide is the counterpart of salt 1, in which 4-H2bpo2+ cations are interweaved by spherical Br− anions and water molecules to generate a similar wavelike chain (Fig. 1c and S9†). Although 3 contains spherical I− anions, bilateral linear I3− ions limit their activities.
It is clear from our present study that the nature and geometry of inorganic anions play a vital role in the crystal packing. Crystal structures here provide a wealth of information about the rational design of inorganic anion-assisted supramolecular assemblies and the application of hydrogen bonds and weak forces in crystal engineering and supramolecular chemistry.
Conclusion
Seven ionic salts have been obtained by the self-assembly of bent dipyridyl 4-bpo, assisted by different inorganic anions (NO3−, HPO42−, I3−/I−, PF6−, BF4−, and SO42−). 3D supramolecular architectures are observed in 1, 2, 5 and 6 but discrete structures are observed in 3, 4 and 7. Such anions can effectively influence supramolecular architectures through extensive intermolecular interactions, ranging from hydrogen bonds, to anion⋯π and π⋯π interactions. The examples here should help to develop supramolecular assemblies assisted by anions and expand the understanding of the vital role of anions in the construction of supermolecules. Additionally, our research here allows us to better understand both competitive and complementary effects between strong hydrogen bonds and weak interactions in crystal engineering and supramolecular chemistry.
Acknowledgements
We gratefully acknowledge financial support from the Natural Science Foundations of China (51173082), from Jiangsu Province and PAPD (13KJB150028, BM2012010, BK20141425 and YX03001) and the Program for Postgraduates Research Innovation in University of Jiangsu Province (CXZZ12-0456).
References
-
J. L. Sessler, P. A. Gale and W. S. Cho, Anion receptor chemistry, Royal Society of Chemistry, 2006 Search PubMed.
- P. A. Gale, Acc. Chem. Res., 2006, 39, 465–475 CrossRef CAS PubMed.
- P. A. Gale, S. E. García-Garrido and J. Garric, Chem. Soc. Rev., 2008, 37, 151–190 RSC.
- J. Pérez and L. Riera, Chem. Soc. Rev., 2008, 37, 2658–2667 RSC.
- J. W. Steed, Chem. Soc. Rev., 2009, 38, 506–519 RSC.
- Z. Zhang and P. R. Schreiner, Chem. Soc. Rev., 2009, 38, 1187–1198 RSC.
- Y. Hua and A. H. Flood, Chem. Soc. Rev., 2010, 39, 1262–1271 RSC.
- S. O. Kang, J. M. Llinares, V. W. Day and K. Bowman-James, Chem. Soc. Rev., 2010, 39, 3980–4003 RSC.
-
R. Custelcean, in Constitutional Dynamic Chemistry, ed. M. Barboiu, Springer-Verlag Berlin, Berlin, 2012, 322, 193–216 Search PubMed.
- A. Caballero, F. Zapata and P. D. Beer, Coord. Chem. Rev., 2013, 257, 2434–2455 CrossRef CAS PubMed.
- R. Custelcean, Chem. Soc. Rev., 2010, 39, 3675–3685 RSC.
- Z. Y. Zhang, Z. P. Deng, L. H. Huo, H. Zhao and S. Gao, CrystengComm, 2013, 15, 5261–5274 RSC.
- P. D. Beer and P. A. Gale, Angew. Chem., Int. Ed., 2001, 40, 486–516 CrossRef CAS.
- N. Gimeno and R. Vilar, Coord. Chem. Rev., 2006, 250, 3161–3189 CrossRef CAS PubMed.
- M. S. Vickers and P. D. Beer, Chem. Soc. Rev., 2007, 36, 211–225 RSC.
- M. D. Lankshear and P. D. Beer, Acc. Chem. Res., 2007, 40, 657–668 CrossRef CAS PubMed.
-
R. Vilar, in Recognition of Anions, ed. R. Vilar, Springer-Verlag Berlin, Berlin, 2008, 129, 175–206 Search PubMed.
- R. Vilar, Eur. J. Inorg. Chem., 2008, 357–367 CrossRef CAS.
- R. Custelcean, D.-e. Jiang, B. P. Hay, W. Luo and B. Gu, Cryst. Growth Des., 2008, 8, 1909–1915 CAS.
- K. Uzarevic, I. Dilovic, N. Bregovic, V. Tomisic, D. Matkovic-Calogovic and M. Cindric, Chem. – Eur. J., 2011, 17, 10889–10897 CrossRef CAS PubMed.
- G. T. Spence and P. D. Beer, Acc. Chem. Res., 2013, 46, 571–586 CrossRef CAS PubMed.
- L. A. Joyce, S. H. Shabbir and E. V. Anslyn, Chem. Soc. Rev., 2010, 39, 3621–3632 RSC.
- J. L. Gulbransen and C. M. Fitchett, CrystEngComm, 2012, 14, 5394–5397 RSC.
- H. T. Chifotides, B. L. Schottel and K. R. Dunbar, Angew. Chem., Int. Ed., 2010, 49, 7202–7207 CrossRef CAS PubMed.
- H. T. Chifotides and K. R. Dunbar, Acc. Chem. Res., 2013, 46, 894–906 CrossRef CAS PubMed.
- A. Caballero, F. Zapata, L. Gonzalez, P. Molina, I. Alkorta and J. Elguero, Chem. Commun., 2014, 50, 4680–4682 RSC.
- P. Gamez, Inorg. Chem. Front., 2014, 1, 35–43 RSC.
- C. Jia, B. Wu, S. Li, Z. Yang, Q. Zhao, J. Liang, Q.-S. Li and X.-J. Yang, Chem. Commun., 2010, 46, 5376–5378 RSC.
- C. Jia, B. Wu, S. Li, X. Huang and X.-J. Yang, Org. Lett., 2010, 12, 5612–5615 CrossRef CAS PubMed.
- C. Jia, B. Wu, S. Li, X. Huang, Q. Zhao, Q. S. Li and X. J. Yang, Angew. Chem., Int. Ed., 2011, 50, 486–490 CrossRef CAS PubMed.
- S. G. Li, C. D. Jia, B. Wu, Q. Luo, X. J. Huang, Z. W. Yang, Q. S. Li and X. J. Yang, Angew. Chem., Int. Ed., 2011, 50, 5720–5723 Search PubMed.
- Z.-P. Deng, H.-L. Qi, L.-H. Huo, H. Zhao and S. Gao, CrystEngComm, 2011, 13, 6632–6642 RSC.
- R. Li, Y. Zhao, S. Li, P. Yang, X. Huang, X.-J. Yang and B. Wu, Inorg. Chem., 2013, 52, 5851–5860 CrossRef CAS PubMed.
- B. Wu, F. J. Cui, Y. B. Lei, S. G. Li, N. D. Amadeu, C. Janiak, Y. J. Lin, L. H. Weng, Y. Y. Wang and X. J. Yang, Angew. Chem., Int. Ed., 2013, 52, 5096–5100 CrossRef CAS PubMed.
- T.-P. Liu, L.-H. Huo, Z.-P. Deng, H. Zhao and S. Gao, RSC Adv., 2014, 4, 40693–40710 RSC.
-
P. A. Gale, W. Dehaen and E. Alcade, Anion Recognition in Supramolecular Chemistry, Springer, 2010 Search PubMed.
- M. Arunachalam and P. Ghosh, Chem. Commun., 2011, 47, 8477–8492 RSC.
- X. H. Ding, Y. H. Li, S. Wang, X. A. Li and W. Huang, J. Mol. Struct., 2013, 1051, 124–131 CrossRef CAS PubMed.
- M. Du, Z. H. Zhang, X. J. Zhao and H. Cai, Cryst. Growth Des., 2006, 6, 114–121 CAS.
- M. Du, Z.-H. Zhang and X.-J. Zhao, Cryst. Growth Des., 2005, 5, 1199–1208 CAS.
- M. Du, Z.-H. Zhang and X.-J. Zhao, Cryst. Growth Des., 2005, 5, 1247–1254 CAS.
- M. Du, Z.-H. Zhang and X.-J. Zhao, Cryst. Growth Des., 2006, 6, 390–396 CAS.
- L. Wang, L. Zhao, Y. J. Hu, W. Q. Wang, R. X. Chen and Y. Yang, CrystEngComm, 2013, 15, 2835–2852 RSC.
- Y.-T. Wang, G.-M. Tang, D.-W. Qin, H.-D. Duan and S. W. Ng, Acta Crystallogr., Sect. E: Struct. Rep. Online, 2006, 62, O3094–O3095 CAS.
- M. T. Han and Y. Zhang, Acta Crystallogr., Sect. E: Struct. Rep. Online, 2010, 66, O3212–U1031 CAS.
- M. Kubicki and P. W. Codding, Acta Crystallogr., Sect. E: Struct. Rep. Online, 2001, 57, o122–o124 CAS.
- F. Bentiss and M. Lagrenee, J. Heterocycl. Chem., 1999, 36, 1029–1032 CrossRef CAS.
Footnote |
† Electronic supplementary information (ESI) available. CCDC 1003925–1003931. For ESI and crystallographic data in CIF or other electronic format see DOI: 10.1039/c4qi00125g |
|
This journal is © the Partner Organisations 2015 |
Click here to see how this site uses Cookies. View our privacy policy here.