DOI:
10.1039/C4MD00331D
(Concise Article)
Med. Chem. Commun., 2015,
6, 182-186
Poly-α,β-aspartyl-Arg-Gly-Asp-Phe: a novel polymeric nanomedicine†
Received
31st July 2014
, Accepted 13th October 2014
First published on 13th October 2014
Abstract
Thrombosis is a pathological condition and has been one of the most prominent causes of morbidity and mortality. Poly-α,β-aspartic acid is a biodegradable polymer, and RGD-tetrapeptides target thrombus. These led to the design of poly-α,β-aspartyl-Arg-Gly-Asp-Phe (PD-RGDF) as a novel polymeric therapeutic drug. In the solid state and ultrapure water PD-RGDF formed nanoparticles of substantially identical diameter. In vitro the nanoparticles inhibited GPIIb/IIIa expression of activated platelets, thereby making the in vitro anti-platelet aggregation efficacy greatly increase. In vivo the anti-thrombotic efficacy of PD-RGDF was 500-fold higher than that of RGDF.
Introduction
During the past three decades, polymeric therapeutics, i.e. biologically active polymeric drugs, such as the bioconjugates of polymer–drugs and polymer–proteins, the block copolymer micelles covalently bound to drugs and polymeric multilayer capsules, have been the topic of a numerous excellent reviews.1–7 For polymeric therapeutics, the aspects of nanoscience and nanobiotechnology have been deeply commented on, in particular.8–10 So far the polymeric therapeutics in the experimental, preclinical and clinical stages as well as on the market are mostly anticancer nanomedicines.8,11–15 In respect of the polymers for constructing nanomedicines, water soluble polymers, reactive polymers and polymer coatings have attracted special interest.16,17 Of the biodegradable polymers, poly-α,β-aspartic acid (PD) is highly susceptible to degradation by the lysosomal enzyme and produces monomeric aspartic acid as the product.18 The modification of PD with fatty acid side groups or amino acids have been used to construct polymeric micelles for encapsulating amphotericin B,19 to treat thrombus,20,21 and remove Pb(II) in vivo.22,23 These representative modifications ensure the utility of PD in preparing polymeric therapeutics.
Bioactive peptides, including receptor-targeted peptides, nuclear localization signaling peptides and cell penetrating peptides, function as chemical messengers, nerve transmitters and ligands of specific receptors capable of regulating physiological and/or pathological processes.24,25 The usual role of bioactive peptides in polymeric therapeutics is to introduce therapeutic or targeted functions.26 Of the integrin ligands, RGD-tetrapeptides plays an important role in a series of physiological and pathological processes. For instance, by inhibiting the fibrinogen binding GPIIb/IIIa, RGD-peptides can block the pathway of platelet aggregation and subsequently inhibit the formation of thrombus.27–31 The modified RGD-peptides have even higher anti-thrombotic activity,32,33 and anti-osteoporosis activity.34–36 Specifically, as a nanocarrier RGDV can target the thrombus,37 a pathological condition and one of the most prominent causes of morbidity and mortality.38,39
Based on the progress made in polymeric therapeutics, PD, RGD-tetrapeptides and nanomedicine, as well as those aimed at thrombus therapy, this work presents a novel nanosized polymeric therapeutic, poly-α,β-aspartyl-Arg-Gly-Asp-Phe (PD-RGDF), evaluated for in vitro anti-platelet aggregation activity and in vivo anti-thrombotic potency, and discusses their mechanism of action.
Results and discussion
Preparation of PD-RGDF
The preparation of polysuccimide and PD outlined in Scheme 1 of the ESI† were performed by following the well-known methods,40,41 in which thermal polycondensation resulted in a rational polysuccimide used for desirable ring opening by Arg-Gly-Asp-Phe to keep the PD and the backbone of PD-RGDF having 119 residues of Asp.20–23 The amino acid analysis of PD-RGDF gave a ratio of Asp
:
Phe
:
Gly
:
Arg = 2.0
:
1.0
:
0.8
:
0.7, and confirmed that RGDF was successfully introduced into PD. The HPLC purity of PD-RGDF was 98.4% (retention time 11.5 min).
Nano-images of PD-RGDF
The nano-structure of PD-RGDF in the solid state and in ultrapure water was imaged using scanning electron microscopy (SEM), transmission electron microscopy (TEM) and atomic force microscopy (AFM), and the images are shown in Fig. 1. The SEM images show that the powders of PD-RGDF obtained from the 10−6 nM aqueous solution are nanoparticles having a diameter of 66–77 nm (Fig. 1A). The TEM images show that in ultrapure water, 10−6 nM PD-RGDF forms nanoparticles having a diameter of 44–156 nm (Fig. 1B). The AFM images show that in rat plasma, 10−6 nM PD-RGDF forms nanoparticles of having a diameter of 50–152 nm (Fig. 1F). The diameter of 44–156 nm means that nanoparticles are suitable for escaping the entrapment of macrophages.42
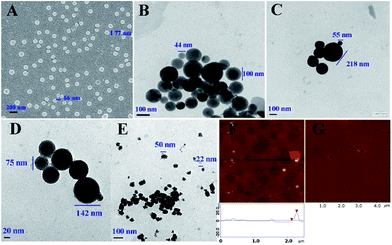 |
| Fig. 1 The nanoimages of PD-RGDF. (A) SEM image of PD-RGDF in the solid state; (B) TEM image of PD-RGDF in ultrapure water; (C) TEM image of PD-RGDF in an aqueous solution of pH 1.2; (d) TEM image of PD-RGDF in an aqueous solution of pH 7.4; (E) TEM image of PD-RGDF in an aqueous solution of pH 7.6; (F) AFM image of PD-RGDF in rat plasma (final concentration 10−6 nM); (G) AFM image of rat plasma alone. | |
The effect of pH on the particle size was further explained using the TEM images of PD-RGDF in an aqueous solution (10−6 M) at pH 1.2 (Fig. 1C), pH 7.4 (Fig. 1D) and pH 7.6 (Fig. 1E). As can be demonstrated, in the three pH environments the diameter of the particles ranged from 22 nm to 142 nm, which still benefited their delivery.
The effect of storage time on the particle size was clarified with 8 day particle size measurements, and the diameter of the particles ranged from 60 nm to 170 nm, which again benefited their delivery. The sizes with standard deviation and the zeta potentials of the particles stored for 12 days in an aqueous solution are also given in the ESI.†
PD-RGDF selectively inhibiting PAF-induced platelet aggregation
Using an in vitro platelet aggregation model, the IC50 values of PD-RGDF inhibition of platelet aggregation induced by platelet activating factor (PAF), adenosine diphosphate (ADP), arachidonic acid (AA) and thrombin (TH) were tested. Fig. 2 indicates that the IC50 value (∼10 nM) of PD-RGDF against PAF induced platelet aggregation was ∼7–35 fold lower than those of it against ADP, AA and TH induced platelet aggregation, suggesting PD-RGDF selectively blocked the action of PAF in inducing platelet aggregation. On the other hand, comparing the IC50 values of RGDF against PAF, ADP, AA and TH induced platelet aggregation the value of PD-RGDF was ∼7–400 fold lower. To know the reason of PD-RGDF having a greatly higher inhibition than RGDF, the SEM of 10−6 nM PD-RGDF treated AA activated rat platelets and the nanostructure of a 1 μM aqueous solution RGDF were measured. It was found that in a 1 μM aqueous solution, RGDF did not form nanoparticles. The SEM image (Fig. 2C) indicates that in the presence of NS, AA activated rat platelets have numerous pseudopods on the surface. The SEM image (Fig. 2D) indicates that in the presence of 10−6 nM PD-RGDF the surface of AA activated rat platelets have numerous nanoparticles, but not pseudopods, some of which are in the endocytosis course. The nanoparticles capable of adhering onto the surface and entering into the cytoplasm of the platelets should be responsible for the excellent in vitro anti-platelet aggregation activity.
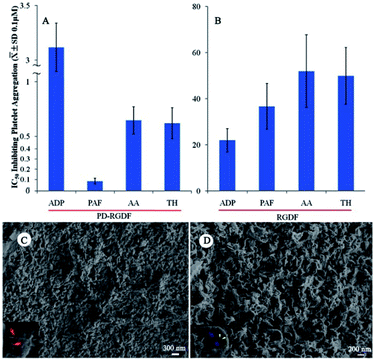 |
| Fig. 2
In vitro anti-platelet aggregation activity of PD-RGDF (A) and RGDF (B), n = 6, SEM image of AA activated rat platelets treated with NS; in the magnified image the red arrowheads point to the pseudopods on the surface of the platelets (C) and SEM image of AA activated rat platelets treated with PD-RGDF; in the magnified image the representative nanoparticles on the surface and in the endocytosis course are labeled with yellow arrowheads and blue arrowheads, respectively (D). | |
PD-RGDF inhibiting rats to develop thrombus
Using a rat model, the anti-thrombotic activity of PD-RGDF was evaluated using doses of 0.1, 1 and 10 nmol kg−1 and represented with thrombus weight. Fig. 3 indicates that PD-RGDF inhibits rats to develop thrombus in a dose dependent manner, and the effective dose is 0.1 nmol kg−1. Moreover, the anti-thrombotic efficacy of 1 nmol kg−1 and 10 nmol kg−1 PD-RGDF equal those of 167 μmol kg−1 aspirin and 5 μmol kg−1 RGDF, respectively. This implies that the effective dose of PD-RGDF is 167
000 and 500 fold lower than those of aspirin and RGDF, respectively. At a dose of 1 nmol kg−1 both PD and a mixture of PD and RGDF exhibit no anti-thrombotic activity, suggesting that the excellent anti-thrombotic activity of 1 nmol kg−1 PD-RGDF is a result of it being a polymeric therapeutic.
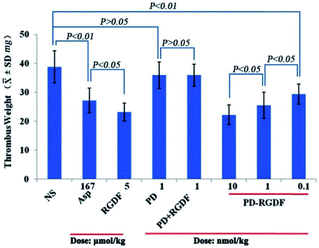 |
| Fig. 3 Effect of PD-RGDF and RGDF on rats developing thrombus, n = 10. | |
Effect of PD-RGDF on GPIIb/IIIa expression
To clarify the interaction of PD-RGDF with GPIIb/IIIa, the expression of GPIIb/IIIa on PD-RGDF and RGDF treated platelets activated by AA was examined using an ELISA experiment according to the procedure for the Rat Platelet Membrane Glycoprotein IIb/IIIa ELISA Kit (Shanghai Yuanye Biotechnology Co. China). Fig. 4 indicates that the level of GPIIb/IIIa expressed by 1 μM PD-RGDF treated platelets is significantly lower than that of GPIIb/IIIa expressed by NS treated platelets, suggesting that at 1 μM, PD-RGDF blocks the expression of GPIIb/IIIa. Moreover, no statistical difference was found between the levels of GPIIb/IIIa expressed by 10 μM RGDF and NS treated platelets, suggesting that at 10 μM, RGDF does not block the expression of GPIIb/IIIa. However, 1 mM RGDF effectively inhibits the expression of GPIIb/IIIa, and its potency equals that of 1 μM PD-RGDF. Therefore, in respect of inhibiting GPIIb/IIIa expression, the potency of PD-RGDF is 1000 fold higher than that of RGDF. The advantage of PD-RGDF to RGDF in inhibiting GPIIb/IIIa expression could also be attributed to PD-RGDF being able to form nanoparticles, which are capable of adhering onto the surface and entering into the cytoplasm of the platelets.
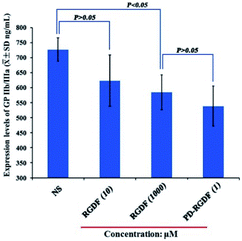 |
| Fig. 4 The level of GPIIb/IIIa expressed with 1 μM PD-RGDF, as well as 10 μM and 1 mM RGDF treated platelets. | |
Experimental
The chemicals, animals and equipment used herein, the synthetic route, the preparations and characteristics of polysuccimide, PD and PD-RGDF, the measurements of the TEM, SEM and AFM images, the in vitro anti-platelet aggregation assay, the in vivo anti-thrombotic assay, the SEM images of rat platelets, the GPIIb/IIIa expression assay, the stability of the nanoparticles and the zeta potentials are described in the ESI.†
Conclusions
Poly-α,β-aspartic acid, consisting of 119 Asp residues is highly susceptible to degradation by lysosomal enzymes and therefore could be used as a biodegradable polymer. To develop a polymeric therapeutic drug, Arg-Gly-Asp-Phe was covalently conjugated with poly-α,β-aspartic acid and gave poly-α,β-aspartyl-Arg-Gly-Asp-Phe as a novel polymeric therapeutic, capable of forming substantially identical nanoparticles in the solid state, ultrapure water and in rat plasma, effectively inhibiting the expression of GPIIb/IIIa and dramatically enhancing the anti-thrombotic efficacy in vivo.
Acknowledgements
This work was supported by Beijing Municipal Science & Technology Commission (Z141100002114049), the Project of Construction of Innovative Teams and Teacher Career Development for Universities and Colleges Under Beijing Municipality, the NSFC (81172930, 81273379, 81202412, 81373264), Beijing NOVA Programme (XX2013039) and the Beijing Natural Science Foundation (7122023).
Notes and references
- P. K. Dhal, S. C. Polomoscanik, L. Z. Avila, S. R. Holmes-Farley and R. J. Miller, Functional polymers as therapeutic agents: concept to market place, Adv. Drug Delivery Rev., 2009, 61, 1121–1130 CrossRef CAS PubMed.
- Y. Matsumura and K. Kataoka, Preclinical and clinical studies of anticancer agent-incorporating polymer micelles, Cancer Sci., 2009, 100, 572–579 CrossRef CAS PubMed.
- D. Edinger and E. Wagner, Bioresponsive polymers for the delivery of therapeutic nucleic acids, Wiley Interdiscip. Rev.: Nanomed. Nanobiotechnol., 2011, 3, 33–46 CrossRef CAS PubMed.
- R. Duncan, Polymer conjugates as anticancer nanomedicines, Nat. Rev. Cancer, 2006, 6, 688–701 CrossRef CAS PubMed.
- K. Jindřich, Polymer–drug conjugates: origins, progress to date and future directions, Adv. Drug Delivery Rev., 2013, 65, 49–59 CrossRef PubMed.
- S. De Koker, L. J. De Cock, P. Rivera-Gil, W. J. Parak, R. A. Velty, C. Vervaet, J. P. Remon, J. Grooten and B. G. De Geest, Polymeric multilayer capsules delivering biotherapeutics, Adv. Drug Delivery Rev., 2011, 63, 748–761 CrossRef CAS PubMed.
- R. Duncan, The dawning era of polymer therapeutics, Nat. Rev. Drug Discovery, 2003, 2, 347–360 CrossRef CAS PubMed.
- L. Kastl, D. Sasse, V. Wulf, R. Hartmann, J. Mircheski, C. Ranke, S. Carregal-Romero, J. A. Martínez-López, R. Fernández-Chacón, W. J. Parak, H. P. Elsasser and P. Rivera Gil, Multiple internalization pathways of polyelectrolyte multilayer capsules into mammalian cells, ACS Nano, 2013, 7, 6605–6618 CrossRef CAS PubMed.
- B. Pelaz, S. Jaber, D. J. de Aberasturi, V. Wulf, T. Aida, J. M. de la Fuente, J. Feldmann, H. E. Gaub, L. Josephson, C. R. Kagan, N. A. Kotov, L. M. Liz-Marzán, H. Mattoussi, P. Mulvaney, C. B. Murray, A. L. Rogach, P. S. Weiss, I. Willner and W. J. Parak, The state of nanoparticle-based nanoscience and biotechnology: progress, promises, and challenges, ACS Nano, 2012, 6, 8468–8483 CrossRef CAS PubMed.
- M. Mahmoudi, J. Meng, X. Xue, X. J. Liang, M. Rahman, C. Pfeiffer, R. Hartmann, P. R. Gil, B. Pelaz, W. J. Parak, P. del Pino, S. Carregal-Romero, A. G. Kanaras and S. T. Selvan, Interaction of stable colloidal nanoparticles with cellular membranes, Biotechnol. Adv., 2014, 32, 679–692 CrossRef CAS PubMed.
- R. M. England, E. Masiá, V. Giménez, R. Lucas and M. J. Vicent, Polyacetal-stilbene conjugates – the first examples of polymer therapeutics for the inhibition of HIF-1 in the treatment of solid tumours, J. Controlled Release, 2012, 164, 314–322 CrossRef CAS PubMed.
- R. Duncan and M. J. Vicent, Polymer therapeutics-prospects for 21st century: the end of the beginning, Adv. Drug Delivery Rev., 2013, 65, 60–70 CrossRef CAS PubMed.
- A. J. Gormley, N. Larson, S. Sadekar, R. Robinson, A. Ray and H. Ghandehari, Guided delivery of polymer therapeutics using plasmonic photothermal therapy, Nano Today, 2012, 7, 158–167 CrossRef CAS PubMed.
- R. Gaspar and R. Duncan, Polymeric carriers: preclinical safety and the regulatory implications for design and development of polymer therapeutics, Adv. Drug Delivery Rev., 2009, 61, 1220–1231 CrossRef CAS PubMed.
- R. Laga, R. Carlisle, M. Tangney, K. Ulbrich and L. W. Seymour, Polymer coatings for delivery of nucleic acid therapeutics, J. Controlled Release, 2012, 161, 537–553 CrossRef CAS PubMed.
- R. M. England, E. Masiá, V. Giménez, R. Lucas and M. J. Vicent, Polyacetal-stilbene conjugates – the first examples of polymer therapeutics for the inhibition of HIF-1 in the treatment of solid tumours, J. Controlled Release, 2012, 164, 314–322 CrossRef CAS PubMed.
- J. Yang and J. Kopeček, Macromolecular therapeutics, J. Controlled Release, 2014, 190, 288–303 CrossRef CAS PubMed.
- E. Markovsky, H. Baabur-Cohen, A. Eldar-Boock, L. Omer, G. Tiram, S. Ferber, P. Ofek, D. Polyak, A. Scomparin and R. Satchi-Fainaro, Administration, distribution, metabolism and elimination of polymer therapeutics, J. Controlled Release, 2012, 161, 446–460 CrossRef CAS PubMed.
- A. Lavasanifar, J. Samuel, S. Sattari and G. S. Kwon, Block copolymer micelles for the encapsulation and delivery of amphotericin B, Pharm. Res., 2002, 19, 418–422 CrossRef CAS.
- L. Gui, M. Zhao, Y. Wang, Y. Qin, J. Liu and S. Peng, Synthesis, nano-features, in vitro thrombus lysis activity and in vivo thrombolytic activity of poly-α,β-DL-aspartyl-L-alanine, Nanomedicine, 2010, 5, 703–714 CrossRef CAS PubMed.
- L. Gui, M. Zhao, Y. Wang, Y. Wang, Y. Qin, L. Li and S. Peng, Synthesis, nano-features, ex vivo anti-platelet aggregation and in vivo anti-thrombotic activities of poly-α,β-DL-aspartyl-L-arginine, Med. Chem. Commun., 2012, 3, 102–108 RSC.
- L. Li, J. Wu, M. Zhao, Y. Wang, H. Zhang, X. Zhang, L. Gui, J. Liu, N. Mair and S. Peng, Poly-a,β-DL-aspartyl-L-cysteine: a novel nano-material having porous structure, special complexation capability for Pb(II) in vitro and selectivity of removing Pb(II) in vivo, Chem. Res. Toxicol., 2012, 25, 1948–1954 CrossRef CAS PubMed.
- H. Zhang, Y. Wang, M. Zhao, J. Wu, X. Zhang, L. Gui, M. Zheng, L. Li, J. Liu and S. Peng, Synthesis and In vivo lead detoxification evaluation of poly-α,β-DL-aspartyl-L-methionine, Chem. Res. Toxicol., 2012, 25, 471–477 CrossRef PubMed.
- S. Lee, J. Xie and X. Chen, Peptide-based probes for targeted molecular imaging, Biochemistry, 2010, 49, 1364–1371 CrossRef CAS PubMed.
- K. M. Stewart, K. L. Horton and S. O. Kelley, Cell-penetrating peptides as delivery vehicles for biology and medicine, Org. Biomol. Chem., 2008, 6, 2242–2245 CAS.
- J. Nicolas, S. Mura, D. Brambilla, N. Mackiewicz and P. Couvreur, Design, functionalization strategies and biomedical applications of targeted biodegradable/bio-compatible polymer-based nanocarriers for drug delivery, Chem. Soc. Rev., 2013, 42, 1147–1235 RSC.
- C. S. Cierniewski, T. Byzova, M. Papierak, T. A. Haas, J. Niewiarowska, L. Zhang, M. Cieslak and E. F. Plow, Peptide ligands can bind to distinct sites in integrin alphaIIbbeta3 and elicit different functional responses, J. Biol. Chem., 1999, 274, 16923–16932 CrossRef CAS PubMed.
- R. Pytela, M. Pierschbacher, M. Ginsberg, E. Plow and E. Ruoslahti, Platelet membrane glycoprotein IIb/IIIa: member of a family of Arg-Gly-Asp – specific adhesion receptors, Science, 1986, 231, 1559–1562 CAS.
- F. D. Suvire, A. M. Rodríguez, M. L. Mak, J. G. Papp and R. D. Enriz, Binding mechanism of RGD and its mimetics to receptor GPIIb/IIIa. A theoretical study, J. Mol. Struct.: THEOCHEM, 2001, 540, 257–270 CrossRef CAS.
- A. McNicol and S. J. Israels, Platelets and anti-platelet therapy, J. Pharmacol. Sci., 2003, 93, 381–396 CrossRef CAS.
- Y. X. Li, Q. Sun, H. Zhang, S.-T. Ren, Y.-R. Liao, Y. Wang, X. L. Shen and B. Wang, A novel anti-platelet peptide (Z4A5) potential for glycoprotein IIb/IIIa inhibits platelet aggregation, Thromb. Res., 2012, 129, e217–222 CrossRef CAS PubMed.
- G. Yang, H. Zhu, M. Zhao, J. Wu, Y. Wang, Y. Wang, M. Zheng, M. Chen, J. Liu and S. Peng, The application of tetrahydroisoquinoline-3-carbonyl-TARGD(F)F as anti-thrombotic agent having dual mechanisms of action, Mol. BioSyst., 2012, 8, 2672–2679 RSC.
- N. Li, G. Kang, L. Gui, M. Zhao, W. Wang, J. Zhang, Y. Yue and S. Peng, Novel Cu(II)-RGD-octapeptides: synthesis, coordination mode, in vitro anti-platelet aggregation/in vivo anti-thrombotic evaluation and correlation of sequence with nano-structure, Nanomed.: Nanotechnol., Biol. Med., 2011, 7, 403–409 CrossRef CAS PubMed.
- G. Kang, Y. Wang, J. Liu, J. Wu, M. Zhao, G. Li, N. Li, L. Peng, X. Zhang, L. Li, N. Mair and S. Peng, Development of three-component conjugates: to get nano-globes with porous surface, high in vivo anti-osteoporosis activity and minimal side effects, J. Mater. Chem., 2012, 22, 21740–21748 RSC.
- Y. Wang, J. Wu, G. Kang, M. Zhao, L. Gui, N. Li, L. Peng, X. Zhang, L. Li and S. Peng, Novel nano-materials, RGD-tetrapeptide-modified 17β-amino-11α-hydroxyandrost-1,4-diene-3-one: synthesis, self-assembly based nano-images and in vivo anti-osteoporosis evaluation, J. Mater. Chem., 2012, 22, 4652–4659 RSC.
- J. Liu, X. Zhang, M. Zhao and S. Peng, Synthesis, evaluation and 3D QSAR analysis of novel estradiol–RGD octapeptide conjugates with oral anti-osteoporosis activity, Eur. J. Med. Chem., 2009, 44, 1689–1704 CrossRef CAS PubMed.
- S. Jin, Y. Wang, H. Zhu, Y. Wang, S. Zhao, M. Zhao, J. Liu, J. Wu, W. Gao and S. Peng, Nano-sized aspirin-Arg-Gly-Asp-Val: delivery of aspirin to thrombus by a target carrier Arg-Gly-Asp-Val tetrapeptide, ACS Nano, 2013, 7, 7664–7673 CrossRef CAS PubMed.
- G. deVeber, In pursuit of evidence-based treatments for paediatric stroke: the UK and chest guidelines, Lancet Neurol., 2005, 4, 432–436 CrossRef.
- S. L. H. Zeiger, E. S. Musiek, G. Zanoni, G. Vidari, J. D. Morrow, G. J. Milne and B. McLaughlin, Neurotoxic lipid peroxidation species formed by ischemic stroke increase injury, Free Radical Biol. Med., 2009, 47, 1422–1431 CrossRef CAS PubMed.
- A. Vegotsky, K. Harada and S. W. Fox, The characterization of polyaspartic acid and some related compounds, J. Am. Chem. Soc., 1958, 80, 3361–3366 CrossRef CAS.
- J. Kovacs, H. N. Kovacs, I. Könyves, J. Császár, T. Vajda and H. Mix, Chemical studies of polyaspartic acids, J. Org. Chem., 1961, 26, 1084–1091 CrossRef.
- Y. Fujita, M. Mie and E. Kobatake, Construction of nanoscale protein particle using temperature-sensitive elastin-like peptide and polyaspartic acid chain, Biomaterials, 2009, 30, 3450–3457 CrossRef CAS PubMed.
Footnote |
† Electronic supplementary information (ESI) available. See DOI: 10.1039/c4md00331d |
|
This journal is © The Royal Society of Chemistry 2015 |
Click here to see how this site uses Cookies. View our privacy policy here.